Transport Across Membranes Mechanisms PDF
Document Details
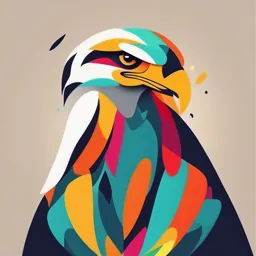
Uploaded by AchievableProtactinium
University of Veterinary Medicine
Máté Mackei
Tags
Summary
This document provides an overview of transport mechanisms across cell membranes. It includes details about passive transport, active transport, facilitated diffusion, and the special case of the Gibbs-Donnan equilibrium. Diagrams and examples are used to illustrate the key concepts.
Full Transcript
Transport across the membrane Máté Mackei Clinical importance: uptake of feed constituents, small and big molecules, drugs, etc. into the cells, organs, organism TYPES OF TRANSPORT Passive transport Active transport Down the...
Transport across the membrane Máté Mackei Clinical importance: uptake of feed constituents, small and big molecules, drugs, etc. into the cells, organs, organism TYPES OF TRANSPORT Passive transport Active transport Down the Against concentration/ concentration/ Direction electrochemical electrochemical gradient gradient Energy Generally no (but at Carrier facilitated yes) Specific inhibition Passive transport across the plasma membrane Passive transport: The molecule or ion crosses the membrane by moving down a concentration or electrochemical gradient and without energy demand, without specific inhibitor Another name is diffusion: simple or facilitated. Passive transport Simple diffusion: molecules can diffuse passively through the lipid components of cellular membranes. Facilitated diffusion: molecules can diffuse passively through proteins of cellular membranes which act as carrier molecules or produce pores, gates. If the solvent is transported through the cellular membrane from the less concentrated solution in the direction of the more concentrated solution is called as: osmosis Osmosis Simple diffusion Small uncharged molecules: oxygen, carbon dioxide, ammonia, ethanol, urea, etc. Usually lipid-soluble non-polar molecules The rate limiting step for simple diffusion across a cell membrane is movement of the molecule from the aqueous environment outside or inside the cell into the lipid bilayer of the membrane Ions and charged molecules diffuse across the lipid bilayer of cell membranes very poorly. Facilitated diffusion There are certain molecules that must get across the plasma membrane BUT the lipid bilayer of the membrane is impermeable for them Their transport must therefore be "facilitated" by proteins that span the membrane and provide an alternative route or bypass Carrier molecules: mostly proteins (transporters): glucose transporters for glucose uptake by the cells carnitine (non-protein) and translocase (protein) for fatty acid uptake by the mitochondrion Hydrophilic pores (gates): such as aquaporin for water uptake Facilitated diffusion of glucose: different glucose transporters in different organs GLUT: glucose transporter GLUT-1: red blood cells, brain non-insulin dependent GLUT-2: intestinal epithelium (basolateral side), liver, kidney partly insulin dependent GLUT-3: brain non-insulin dependent GLUT-4: muscle, adipose tissue insulin dependent: diabetes! Facilitated diffusion of glucose Facilitated diffusion of fatty acids Reason: ß-oxidation of fatty acids in the mitochondrial matrix – Carnitine transports fatty acid molecule into the mitochondrial matrix: fatty acyl~CoA bound to carnitine fatty acyl-carnitine – Translocase: fatty acyl carnitine/carnitine antiporter protein in the mitochondrial inner membrane makes possible for fatty acyl-carnitine to enter into the mitochondrial matrix and then for carnitine to leave the mitochondrion This transport-system is called as „carnitine shuttle”, see next page and later in the „Intermediary metabolism of fatty acids” Enzymes: CPTI=CATI CPTII=CATII binding and (Carnitine releasing AcylTransferase carnitine: I and II) Aquaporin channel Gibbs-Donnan-Equilibrium Basic rule: results in unequal ion distribution on both side of the semipermeable membrane membrane potential J. Willard Gibbs Frederick G. Donnan 1839 - 1903 1870 - 1956 Gibbs-Donnan Equilibrium Solution Equilibrium The Gibbs–Donnan equilibrium can be illustrated using a vessel separated into two chambers by a semi–permeable membrane. A KCl solution is added to one side of the vessel and water to the other side. The membrane in this model is completely permeable to the K+ and Cl– ions. The K + and Cl – ions diffuse passively through the membrane from one side of the vessel to the other. Ions diffuse from high concentrations to low concentrations until equilibrium is attained (each side contains equal concentrations for both the K + and the Cl – ions.) KCl and semipermeable membrane Gibbs-Donnan Equilibrium The system becomes more complicated if an anion is present that is not permeable to the membrane. https://www.youtube.com/watch?v=MhSfQio8mp0 Permeability Properties In this case, the impermeable anion is Pr – a common constituent of cells. K + and Cl – are permeable to the membrane. K + and Cl – easily penetrate the membrane separating the two chambers. Pr – is not permeable and is retained on side. Gibbs-Donnan Equilibrium An unstable situation occurs if one side of the semi–permeable membrane contains a solution consisting of a permeable cations (K+) with an impermeable anions (Pr –) whereas the other side contains a solution of K+ and Cl–, both of which are permeable to the membrane. The K+ concentrations are equimolar on both sides. Since one side of the membrane does not contain Cl–, the Cl– will diffuse along the Cl– concentration gradient. This results in a negative charge on this side relative to the other side due to the excess concentration of anions (Pr – + Cl–). The Cl– concentrations will not become equal on both sides because the negative charge will repel Cl– movement. Gibbs-Donnan Equilibrium Between cytoplasm and ECF exists the Gibbs-Donnan equilibrium, but the active transport of ions will modify this. Gibbs-Donnan Equilibrium Unequal ion distribution on both side of the membrane The net result at equilibrium is that K+ and Cl– move in equimolar amounts together from one side to the other. Each side is electrically neutral within itself due to equimolar concentrations of cations and anions, but there is an unequal concentration of the diffusible ions: Concentration of diffusible catione [K+] is higher on side where non-diffusible Pr – can be found and diffusible anione is [Cl–] lower on side where non-diffusible Pr– can be found: this means the unequal ion distribution on both side of the membrane! Resting membrane potential: -20 mV (but it depends on the cell type!!!) Only for demonstration! It will be not asked! K+ and Cl- are diffusible ions, but Pr– is non- Side II. diffusible Side I. K+=C1 X mmol will cross K+=C1 C1=initial the membrane concent- Cl-=C1 X Pr–=C1 ration Cl-=x Cl-=x Cl-=C1-x Concentration gradient K+=x K+=C1-x Electrochemical gradient K+=C1+x Based on the mass effect rule: the product of the diffusible ion is the same on both side of the membrane (C1-x)2=(C1+x)x C12-2C1x+x2=C1x+x2 X=C12/3C1 if C1=6 X=36/18=2 mmol Cl-=C1-x=6-2=4 mmol So, unequal ion distribution Cl-=x=2 mmol K+=C1-x=6-2=4 mmol K+=C1+x=6+2=8 mmol Clinical importance: uptake of feed constituents, small and big molecules, drugs, etc. into the cells, organs, organism TYPES OF TRANSPORT Passive transport Active transport Down the Against concentration/ concentration/ Direction electrochemical electrochemical gradient gradient Energy Generally no (but at Carrier facilitated yes) Specific inhibition Active transport Active transport involves expenditure of metabolic energy to move ions or other solutes across membranes against their electrochemical gradient Inhibiting the production of ATP: The concentration of ions inside the cell soon drifts toward that of extracellular fluid and the cell dies! Such as in case of myocardial infarction the cardiac muscle cells die or in stroke the neurocytes die 1. Death of cardiac muscle cells 2. Obstruction of artery Active transport Transport of solutes against an electrochemical gradient Primary active transport: involves a carrier protein that directly hydrolyzes ATP to drive the transport process. Secondary active transport (cotransport): utilizes energy stored in concentration or electrochemical gradients of ions to drive transport - these gradients are established and maintained by carrier proteins that directly hydrolyze ATP. Active transport Primary active transport Sodium-potassium pump (Sodium-potassium ATPase) ABC transporter protein family Proton pump Respiratory chain in the epithelial cells of stomach mucous membrane Secondary active transport SGLT-1 and SGLT-2 transporters Sodium-potassium pump: Na+-K+ ATPase One of the most important transport protein In most cells, one-third to one-half of the total energy expended is used to run these ion pumps (nerve cells: 70%) Importance: resting membrane potencial (nerve, muscle) driving force for secondary active transport osmotic gradient Sodium-potassium pump: Na+-K+ ATPase Primary active transport involves a protein which uses ATP to pump molecules against their concentration gradients. The same protein may be used to pump two different substances in opposite directions. This is the case for the very important sodium-potassium pump The pump, with bound ATP, binds 3 intracellular Na+ ions (Mg2+ is also needed for ATP binding) Sodium-potassium pump: Na+-K+ ATPase The Na+ ion concentration is high in the cytoplasm ATP is hydrolyzed, leading to phosphorylation of a cytoplasmic loop of the pump and release of ADP A conformational change in the pump exposes the Na+ ions to the outside, where they are released. The pump binds 2 extracellular K+ ions K+ ions will be released inside the cell and the alpha subunits will be dephosphorylated Sodium-potassium pump: Na+-K+ ATPase Sodium-potassium ATPase 270 kD protein consists of 4 subunits: 2 alpha and 2 beta subunits Inhibition of the sodium-potassium ATPase: cardiac glycosides Widely used to increase the strength of contraction of the heart Inhibition of sodium pump activity in cardiac myocytes → increase in intracellular sodium concentration This leads to an increase in intracellular calcium concentration by sodium-calcium exchange (sodium efflux, Ca influx) Enhancing cardiac contractility Inhibition of the sodium-potassium ATPase: cardiac glycosides Digitalis purpurea (Foxglove) Digitalis lanata Pharmacology: digitalis, digoxin Inhibition of the sodium-potassium ATPase: cardiac glycosides Strophanthus gratus (Climbing oleander, cream fruit; Africa) Pharmacology: strophantin, cardiac glicosides Toxicology: ouabain, arrow toxin Proton pump (primary active transport) Proton pump in the respiratory chain (primary active transport), see later in Biochemistry 2. Proton pump in the epithelial cells of stomach mucous membrane (HCl-Secretion): primary active transport also! ABC transporter protein family ABC = ATP-Binding Casette Consists of 2 transmembrane domains and 2 ATP-binding domains (casettes): MDR = multi-drug resistance protein Pumping non-polar molecules (drugs) out of the cell Drug resistance The tumor cells have MDR against cytostatic drugs Secondary active transport Transport across membranes can be fueled not only by ATP, but by the energy stored in ion gradients In such cases, the free energy released during the transport of ions down an electrochemical or concentration gradient is used to pump other ions or molecules up the gradient. This process is called cotransport because one carrier protein mediates the transport of both species Some cotransporters carry both solutes in the same direction (symport), while others transport one solute into the cell and the other out of the cell (antiport) Forms of secondary active transport Secondary active transport: SGLT-1, SGLT-2 A well-studied example of symport is the cotransport of glucose and 2 sodium ions SGLT-1: small intestinal absorptive epithelial cells (apical side; sodium-glucose linked transporter) Transport of glucose from the intestinal epithelial cells-basolateral side into the circulation happens by facilitated diffusion: GLUT-2 SGLT-2: Kidney Glucose is reabsorbed together with 2 sodium into the tubular epithelial cells from the filtrate by SGLT-2 transporter. Cotransport, symport Secondary active transport: SGLT-1, SGLT-2 Secondary active transport: The glucose/Na+ symport (from the lumen into the intestinal epithelial cells) Uses the energy stored in the Na+ gradient (produced by the Na+/K+ ATPase) to transport glucose against its concentration gradient. Even if there is more glucose in the enterocytes than in the lumen, glucose will be absorbed in the enterocytes Secondary active transport: The one glucose / two Na+ symport Thank you very much for your kind attention!