Topic 5. Cell Part B PDF
Document Details
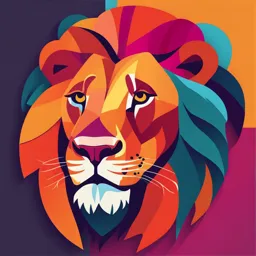
Uploaded by WellManagedParrot
Tags
Related
- Module 7_ The Cytoskeleton - BMSC6010E Fundamental Cell Biology Fall 2023 PDF
- General Biology I - Cell Structure and Function - PDF
- Biology 2e Chapter 4 Cell Structure PDF
- Ibn Sina University College of Medicine First Year Biology Lecture Notes PDF
- Molecular Cell Biology Notes PDF
- Cell Structure 2 - Cell Biology 3 PDF
Summary
This document provides information on the cell, specifically on the cytoskeleton, extracellular components, and cell junctions. Recommended reading from Campbell Biology is also mentioned.
Full Transcript
Topic 5. The cell Part B. Cytoskeleton, extracellular components, cell junctions Copyright © 2005 Pearson Education, Inc. publishing as Benjamin Cummings Learning Objectives (LOBs) 4. Describ...
Topic 5. The cell Part B. Cytoskeleton, extracellular components, cell junctions Copyright © 2005 Pearson Education, Inc. publishing as Benjamin Cummings Learning Objectives (LOBs) 4. Describe the structure and function of the different components and filaments of the cytoskeleton, including the role of the centrosome, the flagella and the cilia. 5. Describe the structure and function of the different extracellular components (of animal and plant cells). 6. Identify the different types of intercellular junctions in animal cells vs plant cells and their function. Recommended reading: Chapter 7 (Campbell Biology) Copyright © 2005 Pearson Education, Inc. publishing as Benjamin Cummings Part I The Cytoskeleton Copyright © 2005 Pearson Education, Inc. publishing as Benjamin Cummings Fig. 7-9a Nuclear envelope ENDOPLASMIC RETICULUM (ER) Nucleolus NUCLEUS Rough ER Smooth ER Flagellum Chromatin Centrosome Plasma membrane CYTOSKELETON: Microfilaments Intermediate filaments Microtubules Ribosomes Microvilli Golgi Peroxisome apparatus Mitochondrion Lysosome Copyright © 2005 Pearson Education, Inc. publishing as Benjamin Cummings The cytoskeleton Cytoskeleton: a network of filaments extending throughout the cytoplasm Composed of three types of filaments: – Microfilaments: actin filaments, the thinnest components – Intermediate filaments: filaments with middle-range diameters, composed by different types of proteins (e.g. keratin) – Microtubules: tubulin filaments, the thickest of the three components of the cytoskeleton Copyright © 2005 Pearson Education, Inc. publishing as Benjamin Cummings Cytoskeleton: Types of filaments Intermediate filaments Microtubules Microfilaments Copyright © 2005 Pearson Education, Inc. publishing as Benjamin Cummings Alberts et al, Molecular Biology of the Cell Copyright © 2005 Pearson Education, Inc. publishing as Benjamin Cummings Roles of the Cytoskeleton: Support, Motility, and Regulation Cytoskeleton functions: 1. Mechanical support to the cell 2. Maintains cell shape 3. Anchors organelles =>organizes the cell’s structures and activities 4. Dynamic: can be assembled and disassembled changing the shape of the cell 5. Cell movement (motility) Copyright © 2005 Pearson Education, Inc. publishing as Benjamin Cummings Cytoskeleton Copyright © 2005 Pearson Education, Inc. publishing as Benjamin Cummings Microtubules 10 µm Column of tubulin dimers 25 nm Tubulin dimer Copyright © 2005 Pearson Education, Inc. publishing as Benjamin Cummings Microtubules Hollow rods, the thickest component of the cytoskeleton Helical polymers made by tubulin monomers => can increase or decrease in size by addition or removal of monomers Microtubule functions: – Mitotic spindle formation => separation of chromosome copies in dividing cells – Shape the cell – Guide movement of organelles Copyright © 2005 Pearson Education, Inc. publishing as Benjamin Cummings Μicrotubules Consist of α and β-tubulin dimers that make up 13 protofilaments Each tubulin dimer has 2 GTP bound (+) end: fast polymerisation (addition of monomers) (-) end: slow polymerisation Subunits (tubulin dimer) α-tubulin β-tubulin Protofilament Copyright © 2005 Pearson Education, Inc. publishing as Benjamin Cummings 10 0 Microtubule stability Continuous polymerisation/depolymerisation of the microtubules depending on the cell requirements The continuous polymerisation/depolymerisation of microtubule is controlled by: GTP hydrolysis: -GTP attached to β-tubulin hydrolyzed to GDP during tubulin polymerisation -The GTP bound to α-tubulin does not hydrolyze during tubulin polymerisation (has structural role) Cytosolic calcium concentration: - [Ca+2] > 0.5 mM=> depolymerisation Copyright © 2005 Pearson Education, Inc. publishing as Benjamin Cummings Microtubule polymerisation (+) end (-) end Copyright © 2005 Pearson Education, Inc. publishing as Benjamin Cummings Alberts et al, Molecular Biology of the Cell Microtubule stability: clinical correlations Drugs that affect microtubule stability/formation: Αnti-mitotic drugs: inhibit the mitotic spindle formation Example: Anti-inflammatory and anti-cancer drugs: - Colchicine: binds to tubulin monomers => inhibits microtubule polymerisation - Τaxol: binds to tubulin monomers => stabilises microtubules by inhibiting their depolymerisation during mitotic anaphase Copyright © 2005 Pearson Education, Inc. publishing as Benjamin Cummings Microtubule polymerisation Begins at the ΜΤOC (Microtubule organizing centers) of the cells Microtubule organizing centers (MTOC): - Centrosome: in most non-dividing cells - Βasal body: in flaggelated and ciliated cells - Polar body: in some fungi (part of the nuclear envelope) - Chromosomal kinetochores of the mitotic spindle: in dividing cells (during metaphase) Microtubule orientation: - Τhe (-) end is oriented towards the cell center (MTOC) - The (+) end is oriented towards cell periphery Copyright © 2005 Pearson Education, Inc. publishing as Benjamin Cummings ΜTOC types Non-dividing cell Flagellated/ciliated cell Dividing (metaphase) cell Microtubule orientation: (-) end towards MCOT (cell center) (+) end towards cell periphery Copyright © 2005 Pearson Education, Inc. publishing as Benjamin Cummings Alberts et al, Molecular Biology of the Cell Microtubules: Centrosomes and Centrioles Centrosome: – a “microtubule-organizing center” (MTOC) – Consists of a centriole pair – Located near the nucleus (cell center) – Found in animal cells only – Yeast and plant cells do not have centrosomes but probably have other structures with the same role Copyright © 2005 Pearson Education, Inc. publishing as Benjamin Cummings Microtubules: Centrosomes and Centrioles Centrosome structure: has 2 centrioles (centriole pair) Centrioles: each consists of 9 triplets of microtubules (9+0 arrangement) Centrosome Centriole pair: a pair of cylindrical rods Microtubule at right angles Centrioles to one another 0.25 µm Longitudinal section Microtubules Cross section Figure 7.22 of one centriole of the other centriole Copyright © 2005 Pearson Education, Inc. publishing as Benjamin Cummings Centrosome structure and function Pericentriolar material (cloud) = space around centrosome - Function: microtubule nucleation (initiation of polymerisation) - contains γ-tubulin: facilitates the nucleation of the α/β tubulin dimers by binding to the (-) end of microtubules induces their nucleation (polymerisation) by forming rings into which the microtubule assemble and elongate γ-tubulin Copyright © 2005 Pearson Education, Inc. publishing as Benjamin Cummings Centrosome structure and function Copyright © 2005 Pearson Education, Inc. publishing as Benjamin Cummings Microtubules: role in motility Microtubules are used as “monorails” for the movement of cellular cargo (vesicles, organelles and chromosomes) From the cell centre to the periphery and vice versa Microtubules Nucleus Copyright © 2005 Pearson Education, Inc. publishing as Benjamin Cummings Microtubules: role in motility Microtubules interact with motor proteins to produce motility Vesicles can travel along the network of fibers provided by the cytoskeleton Vesicle ATP Receptor for motor protein Motor protein Microtubule (ATP powered) of cytoskeleton (a) Motor proteins that attach to receptors on organelles can “walk” the organelles along microtubules or, in some cases, microfilaments. Microtubule Vesicles 0.25 µm (b) Vesicles containing neurotransmitters migrate to the tips of nerve cell axons via the mechanism in (a). In this SEM of a squid giant axon, two vesicles can be seen moving along a microtubule. (A separate part of the Figure 7.21 experiment provided the evidence that they were in fact moving.) Copyright © 2005 Pearson Education, Inc. publishing as Benjamin Cummings Microtubules: role in motility Motor proteins: transport cellular cargo toward opposite ends of microtubules 2 cytoplasmic motor proteins: Dynein: involved in transport from periphery to the cell center (retrograde to microtubule; from + to – end). Kinesin: involved in transport from the cell center to the periphery (anterograde to microtubule; from – to + end) Copyright © 2005 Pearson Education, Inc. publishing as Benjamin Cu mmings Microtubules: role in motility Membrane-bound vesicles (transport vesicles) Golgi Mitochondrion apparatus Lysosome Lysosome Cytosolic dynein Cytosolic kinesin Spindle kinesin https://www.youtube.com/watch?v=y-uuk4Pr2i8 Copyright © 2005 Pearson Education, Inc. publishing as Benjamin Cummings Microtubules: motor proteins Cytoplasmic organelle or vesicle Cell periphery Cell centre Movement Movement direction direction Kinesin Dynein (+) (+) end (-) end Microtubule Tubulin dimer (α,β tubulin) Copyright © 2005 Pearson Education, Inc. publishing as Benjamin Cummings Cell motility using microtubules: Cilia and Flagella Cilia and flagella: – Permanent locomotor appendages of some eukaryotic cells – contain specialized arrangements of microtubules Flagella vs cilia motility pattern Copyright © 2005 Pearson Education, Inc. publishing as Benjamin Cummings Flagella Typically a single flagellum per cell (in eukaryotes) Flagella motility pattern: snakelike motion Example: sperm cells (a) Motion of flagella. A flagellum usually undulates, its snakelike Direction of swimming motion driving a cell in the same direction as the axis of the flagellum. Propulsion of a human sperm cell is an example of flagellate locomotion (LM). Figure 7.23A 1 µm Copyright © 2005 Pearson Education, Inc. publishing as Benjamin Cummings Cilia Typically a lot of cilia per cell Ciliary motility pattern: back-and-forth motion Example: trachea cells, protists, fallopian tubes (b) Motion of cilia. Cilia have a back- and-forth motion that moves the cell in a direction perpendicular to the axis of the cilium. A dense nap of cilia, beating at a rate of about 40 to 60 strokes a second, covers this Colpidium, a freshwater protozoan (SEM). 15 µm Figure 7.23 B Copyright © 2005 Pearson Education, Inc. publishing as Benjamin Cummings Cilia and flagella structure Cilia and flagella share a common ultrastructure Consist of the axoneme surrounded by the plasma membrane Αxoneme: - The central strand of a cilium or flagellum - Composed of microtubules (9 pairs around 2 single central ones= 9+2 arrangement) Axonemal proteins: - Dynein: motor protein responsible for motility - Nexin: connects microtubule doublets (pairs) between them Copyright © 2005 Pearson Education, Inc. publishing as Benjamin Cummings Cilia and flagella structure Central microtubule pair (doublet) Cross section of an axoneme Copyright © 2005 Pearson Education, Inc. publishing as Benjamin Cummings Cilia and flagella structure Basal body: a protein structure found at the base of a eukaryotic cilium or flagellum. Consists of 9 triplets of microtubules (like centrioles). Outer microtubule Plasma doublet membrane 0.1 µm Dynein arms Central microtubule Outer doublets cross-linking proteins inside Microtubules Radial Plasma spoke membrane (b) Cross section of flagella Basal body 9+2 arrangement 0.5 µm 0.1 µm (a) Longitudinal section of flagella Triplet 9+0 Figure 7.24 A-C (c) Cross section of basal body arrangement Copyright © 2005 Pearson Education, Inc. publishing as Benjamin Cummings Cilia and flagella structure 1= axoneme 2= cell membrane 3= intraflagellar transport 4=Basal body 5=cross section of flagella 6=microtubule triplets of basal body Copyright © 2005 Pearson Education, Inc. publishing as Benjamin Cummings Cilia and flagella structure Axonemal dynein: responsible for the bending movement of cilia and flagella - Similar but larger than cytosolic dynein - has ATPase activity => ATP hydrolysis => bending of flagella/cilia Microtubule doublets ATP Dynein arm (a) Powered by ATP, the dynein arms of one microtubule doublet grip the adjacent doublet, push it up, release, and then grip again. If the two microtubule doublets were not attached, they would slide Figure 7.25A relative to each other. Copyright © 2005 Pearson Education, Inc. publishing as Benjamin Cummings Cilia and flagella structure Outer doublets ATP cross-linking proteins Axonemal dynein: - links peripheral 9 microtubule doublets -causes bending of Anchorage cilium/flagellum by in cell (basal differential sliding body) of doublets (b) In a cilium or flagellum, two adjacent doublets cannot slide far because they are physically restrained by proteins, so they bend. (Only two of Figure 7.25 B the nine outer doublets in Figure 7.24b are shown here.) Copyright © 2005 Pearson Education, Inc. publishing as Benjamin Cummings Cilia and flagella structure 1 3 2 (c) Localized, synchronized activation of many dynein arms probably causes a bend to begin at the base of the cilium or flagellum and move outward toward the tip. Many successive bends, such as the ones shown here to the left and right, result in a wavelike motion. In this diagram, the two central Figure 7.25 C microtubules and the cross-linking proteins are not shown. Copyright © 2005 Pearson Education, Inc. publishing as Benjamin Cummings Summary of motor protein types and functions Motor protein Function Cytosolic kinesin Vesicle and organelle transport from the cell centre to the periphery (anterograde to microtubule; from – to + end) Cytosolic dynein Vesicle and organelle transport from periphery to the cell centre (retrograde to microtubule; from + to – end) Axonemal dynein On axonemal microtubules. Causes movement of cilia/flagella. Spindle kinesin Mitotic spindle assembly and chromosome segregation during cell division All the above motor proteins have ATPase activity => ATP hydrolysis => produce energy used for motility Copyright © 2005 Pearson Education, Inc. publishing as Benjamin Cummings Microfilaments 10 µm Actin subunit 7 nm Copyright © 2005 Pearson Education, Inc. publishing as Benjamin Cummings Microfilaments (Actin Filaments) Consist of actin protein monomers 2 twisted chains of actin polymers Functions: - Support cell shape: e.g. the microfilaments that make up the microvilli core of intestinal epithelial cells - Cell motility: formation of pseudopodia => cell movement and phagocytosis (e.g. amoeba) actin-myosin contractile system in muscle cells Copyright © 2005 Pearson Education, Inc. publishing as Benjamin Cummings Microfilaments: found in intestinal microvilli Microvillus Plasma membrane Microfilaments (actin filaments) Intermediate filaments Figure 7.26 0.25 µm Copyright © 2005 Pearson Education, Inc. publishing as Benjamin Cummings Microfilaments that function in cellular motility Actin-myosin contractile system in muscle cells => Contraction of muscle cells Muscle cell Actin filament Myosin filament Myosin arm Figure 7.27A (a) Myosin motors in muscle cell contraction. Copyright © 2005 Pearson Education, Inc. publishing as Benjamin Cummings Amoeba (protozoon): pseudopodia formation Pseudopodia: transient (non-permanent) locomotor (motility) appendages pseudopodia Copyright © 2005 Pearson Education, Inc. publishing as Benjamin Cummings Microfilaments that function in cellular motility Amoeboid movement: involves the contraction of actin and myosin filaments => pseudopodia formation Filaments in the direction of cell movement lengthen (polymerize) and shorten (depolymerize) in the opposite direction Cortex (outer cytoplasm): gel with actin network Inner cytoplasm: sol with actin subunits Extending Microfilaments pseudopodium shorten Microfilaments lengthen Figure 7.27 B (b) Amoeboid movement Copyright © 2005 Pearson Education, Inc. publishing as Benjamin Cummings Microfilaments that function in cellular motility Cytoplasmic streaming: another form of locomotion created by microfilaments Cytoplasmic streaming is the directed flow of cytosol and organelles around the central vacuole in large fungal and plant cells Nonmoving cytoplasm (gel) Chloroplast Streaming cytoplasm (sol) Parallel actin filaments Cell wall Figure 7.27 C (b) Cytoplasmic streaming in plant cells Copyright © 2005 Pearson Education, Inc. publishing as Benjamin Cummings Microfilament polymerisation Actin monomers= G-actin (globular) Actin polymers = F-actin (filamentous) Microfilament assembly/polymerisation: - G-actin conversion to F-actin - Energy provided by ATP hydrolysis - (+) end (barbed end): fast polymerisation - (-) end (pointed end): slow polymerisation - Nucleation: spontaneous formation of G-actin trimers/tetramers => polymer formation (F-actin) nucleus nucleus nucleus G-actin F-actin end F-actin (+) end Elongation Stability Nucleation Actin filament polymerisation Depoly- merisation polymerisation G-actin F-actin Microfilament polymerisation Intermediate Filaments Intermediate filaments: larger than microfilaments but smaller than microtubules Function: – Support cell shape – Fix organelles in place – More permanent than other filaments – Composed of different protein family categories (e.g. keratins) Copyright © 2005 Pearson Education, Inc. publishing as Benjamin Cummings Intermediate filaments 5 µm Keratin proteins Fibrous subunit (keratins coiled together) 8–12 nm Copyright © 2005 Pearson Education, Inc. publishing as Benjamin Cummings Intermediate filaments Intermediate filament types: - Keratin: in epithelial cells - Desmin: in muscle cells - Vimentin: in mesenchymal cells - Neurofilaments: in neurons - GFAP (glial fibrillary acidic proteins): in neuroglia (glia) - Lamins: in nuclear envelope Mesenchymal cells: cells that develop into the lymphatic and circulatory systems tissues, and connective tissues (e.g. bone and cartilage). Copyright © 2005 Pearson Education, Inc. publishing as Benjamin Cummings Intermediate filament functions Support cell shape => provide tissues with resistance to mechanical stress Stretching of a tissue with Stretching of a tissue lacking intermediate filaments intermediate filaments Stretching of cells that contain Stretching of cells that do not intermediate filaments contain intermediate filaments No cell/tissue Cell damage => tissue rupture damage Copyright © 2005 Pearson Education, Inc. publishing as Benjamin Cummings Alberts et al, Molecular Biology of the cell Intermediate filament functions Participate in cell junction formation (e.g. epithelial cell desmosomes) Copyright © 2005 Pearson Education, Inc. publishing as Benjamin Cummings Intermediate filament structure Primary structure Intermediate filament structure α-helical monomer coiled-coil dimer Protofilament (tetramer) Protofibril (2 protofilaments) 10 nm Intermediate (100 Å) filament (4 protofibrils= 8 protofilaments) Κeratins Found in epithelial and epidermal cells In epithelial cell desmosomes => cytokeratins Major component of hair and nails, in intestinal epithelium squamous epidermal epithelium Desmin In muscle cells Connects myofibrils and Ζ-disks of the sarcomeres to each other Sarcomere: basic unit of contraction of striated muscle tissue= the area between the two Z-disks. Z-disk: a thin, dark disk that transversely bisects a striated muscle fiber. Vimentin (another IF) also participates in Z-disk structure organisation in muscle cells Myofibril structure Myofibril sarcomere structure (myosin) (actin) Similar to Figure 7.27 A. The actin-myosin contractile system in the muscle cell sarcomeres. Myofibril structure Desmin and vimentin filaments (IFs) connect the Z disks between them. IFs Z-disk Glia fibrils: in neuroglia Neuroglia (glia): non-neuronal cells that maintain homeostasis, form myelin, and provide support and protection for neurons in the nervous system. Glia fibrils: found in neuroglia (e.g. astrocytes) - GFAP= Glial Fibrillary Acidic Protein => polymerised to form glia fibrils - Role in astrocytic projection formation => CNS morphology Lamins: nuclear envelope Nuclear membrane Ribosomes Lamins Hetero- chromatin nucleus TEM picture: lamin filaments in the inner site of the nuclear envelope (provide structural support) Clinical correlations: cytoskeletal disorders Chediak-Higashi syndrome: - microtubule-based lysosomal mobility inherited defect (lysosomal trafficking defect) resulting in reduced fusion of phagosomes and lysosomes during phagocytosis. - Results in recurrent infections (inability to destroy microorganisms by phagocytosis) Kartagener's syndrome: - immotile cilia/flagella due to a dynein arm inherited defect. - Results in male and female infertility (immotile sperm), sinusitis (bacteria and particles not pushed out) Copyright © 2005 Pearson Education, Inc. publishing as Benjamin Cummings Part II Extracellular components Copyright © 2005 Pearson Education, Inc. publishing as Benjamin Cummings Extracellular components Most cells synthesize and secrete materials that are external to the plasma membrane Extracellular components and connections between cells help coordinate cellular activities These extracellular structures include: – Cell walls of plant cells – The extracellular matrix (ECM) of animal cells – Intercellular junctions Copyright © 2005 Pearson Education, Inc. publishing as Benjamin Cummings Fig. 7-9b Nuclear envelope Rough endoplasmic Nucleolus reticulum NUCLEUS Chromatin Smooth endoplasmic reticulum Ribosomes Central vacuole Golgi apparatus Microfilaments Intermediate CYTO- filaments SKELETON Microtubules Mitochondrion Peroxisome Chloroplast Plasma membrane Cell wall Plasmodesmata Wall of adjacent cell Copyright © 2005 Pearson Education, Inc. publishing as Benjamin Cummings Plant Cell Walls The cell wall: – extracellular structure of plant cells that distinguishes them from animal cells – Prokaryotes, fungi and some protists also have cell walls (prokaryotic and fungal cell walls have different composition) Plant cell walls are made of cellulose fibers embedded in other polysaccharides and protein Functions: - protects the plant cell - maintains its shape - prevents excessive uptake of water Copyright © 2005 Pearson Education, Inc. publishing as Benjamin Cummings The Extracellular Matrix (ECM) of Animal Cells Animal cells: – Lack cell walls – covered by the extracellular matrix (ECM) ECM consists of glycoproteins and proteoglycans – Glycoproteins: glycosylated proteins (proteins with attached carbohydrate residues) e.g. collagen, fibronectin, laminin – Proteoglycans: proteinated carbohydrates (carbohydrates with attached protein residues) ECM functions : support, adhesion, movement, regulation of gene expression Copyright © 2005 Pearson Education, Inc. publishing as Benjamin Cummings ECM components Major proteins and glycoproteins: Collagen: major ECM glycoprotein (12 types) Fibronectin: ECM glycoprotein that connects to plasma membrane proteins (integrins) and to other ECM components (e.g. collagen) => connects plasma membrane with extracellular molecules Laminin: basement membrane glycoprotein Entactin: basement membrane glycoprotein Εlastin: connective tissue protein Proteoglycans: composed of proteins + glycosaminoglycans (GAGs) The Extracellular Matrix (ECM) of Animal Cells Integrins: transmembrane proteins that bind to several ECM components A proteoglycan complex Polysaccharide EXTRACELLULAR FLUID molecule Collagen Carbo- hydrates Core protein Fibronectin Plasma Proteoglycan membrane Integrins molecule Integrin CYTOPLASM Micro- Figure 7.29 filaments Copyright © 2005 Pearson Education, Inc. publishing as Benjamin Cummings Ιntegrins: Cell surface receptors for ECM components CAMs= Cell adhesion molecules: Cell surface transmembrane proteins that bind to the different ECM components 20 different types Structure:Heterodimers made of one α and one β subunit Extracellular domain: binds to the ECM glycoproteins (e.g. fibronectin) via a specific tripeptide sequence (Arg-Gly-Asp= RGD sequence) Intracellular domain: binds to cytoskeletal filaments (microfilaments or intermediate filaments) Function: they link ECM components to cytoskeletal components inside the cell => Activation of cell-signalling pathways => signal transduction => cell survival/proliferation Ιntegrin stucture Major integrin types in vertebrates Name Ligands Distribution α1β1 Collagens, laminins Many α2β1 Collagens, laminins Many α3β1 Fibronectin, laminin Many α4β1 Fibronectin, VCAM-1 Hematopoietic cells α5β1 Fibronectin widespread α6β1 laminins widespread α7β1 laminins muscle, glioma Ig superfamily (ICAM-1 and ΙCAM-2) αLβ2 T-lymphocytes Serum proteins (C3b, Factor αMβ 2 Neutrophils and monocytes X,fibrinogen), ICAM-1 αIIbβ3 fibrinogen, fibronectin Platelets ocular melanoma; neurological αVβ1 Vitronectin, fibrinogen tumours Vitronectin, fibronectin, fibrinogen, activated endothelial cells, αVβ3 osteopontin, collagen melanoma, glioblastoma widespread, esp. fibroblasts, αVβ5 vitronectin and adenovirus epithelial cells proliferating epithelia, esp. lung αVβ6 Fibronectin, TGFβ 1+3 and mammary gland αVβ8 fibronectin, TGFβ1+3 neural tissue; peripheral nerve α6β4 Laminins Epithelial cells ECM glycoproteins: Collagen Major ECM glycoprotein and most abundant protein in the human body. Organizes and strengthens extracellular matrix. 12 different collagen types (I-IV most common) Produced by fibroblasts and epithelial cells Composition: - 3 helical chains (triple helix) Repetitive motif Gly-X-Υ (X,Υ= proline, hydroxyproline, or hydroxy-lysine) Copyright © 2005 Pearson Education, Inc. publishing as Benjamin Cummings Collagen structure α-helix Triple helix Collagen fibers Collagen molecules Collagen types Collagen type Tissue location Associated syndromes Type I Skin, tendon, organs, bone Reduced production in (most common) osteogenesis imperfecta (OI) type I. Type II cartilage - Type III skin, blood vessels, uterus, deficient in vascular type Ehlers- fetal tissue, etc Danlos syndrome Type IV Basement membrane Defective in Alport syndrome /smooth muscle cell Basement membrane (basal lamina): specialized ECM type that separates epithelium/mesothelium/endothelium from underlying connective tissue Basement membrane (basal lamina) basement membrane Basement membrane (basal lamina): specialized ECM type that separates an epithelial/ endothelial cell layer form the underlying connective tissue Connective tissue: consists mostly of ECM secreted by the fibroblasts Basement membrane structure Perlecan Collagen IV Laminin Entactin Fibronectin Major ECM glycoprotein Function: cell attachment to ECM components - Fibronectin has domains for binding integrins (cell surface receptors) and other ECM glycoproteins/proteoglycans (e.g. collagen, heparin, etc). => It mediates cell adhesion to the ECM Fibronectin structure Collagen binding domain Cell binding domain Heparin binding domain Εlastin Elastin is a stretchy protein in connective tissue Function: allows many tissues (e.g. blood vessels, lungs, ligaments, vocal cords) to resume their shape after stretching or contracting Εlastic fibers: consist of glycoproteins (e.g. fibrillin) connected with cross-linked elastin Major arterial extracellular component => confers elasticity Clinical correlations: - Marfan syndrome: connective tissue disorder caused by a defect in fibrillin, a glycoprotein that forms a sheath around elastin (in elastic fibers) - Wrinkles of aging are due to reduced collagen and elastin production. Elastic fiber structure Laminin and Entactin Laminin: - Located mainly in basement membrane, synthesized by adjacent epithelial cells - Binds to basement membrane components (collagen ΙV, heparin, etc) and cell surface receptors (e.g. integrins) - Function: connects epithelial cells with the ECM (mainly basement membrane) Entactin: ECM glycoprotein - Binds to basement membrane components (laminin, collagen IV and proteoglycans) only (does not bind to integrins) - Function: Assists assembly of basement membrane components E.g. Links laminin with collagen in the basement membrane Laminin structure ECM proteins and glycoproteins Role Collagen Most abundant glycoprotein. Organizes and strengthens extracellular matrix. Binds to the cell (via integrin) Fibronectin Connects cells (via integrins) to ECM components (e.g. collagen) Laminin Connects cells (via integrins) to basement membrane components Entactin Connects basement membrane components between them Elastin Major component of elastic fibers together with fibrillin Proteoglycan structure Proteoglycan composition: core protein (5- 10%) + glycosaminoglycans (GAG; 90-95%) - Example: aggrecan Glycosaminoglycans (GAGs): - Polysaccharide composed from a repetitive disaccharide unit (70-200 units) - Disaccharide unit composed of: N-acetyl-glucosamine or N- acetyl-galactosamine + glucuronic acid or iduronic acid - 4 GAG groups (types): 1. Hyaluronic acid (hyaluronan) 2. Chondroitin sulfate and dermatan sulfate 3. Heparin and heparan sulfate 4. Keratan sulfate Copyright © 2005 Pearson Education, Inc. publishing as Benjamin Cummings Proteoglycan structure Hyaluronic acid (a GAG type) Proteoglycan structure Copyright © Alberts etEducation, 2005 Pearson al, Molecular Biology Inc. publishing of the as Benjamin Cell Cummings Proteoglycan structure Copyright © 2005 Pearson Education, Inc. publishing as Benjamin Cummings Proteoglycans Copyright © 2005 Pearson Education, Inc. publishing as Benjamin Cummings Alberts et al, Molecular Biology of the Cell Proteoglycan structure: example GAGs Aggrecan (GAG type) Aggrecan aggregate structure (GAG (GAG type) type) Aggrecan aggregate = aggrecan + hyaluronic acid Copyright © Alberts etEducation, 2005 Pearson al, Molecular Biology Inc. publishing of the as Benjamin Cell Cummings III. Intercellular Junctions Intercellular junctions: neighbouring cells in tissues, organs, or organ systems often adhere, interact, and communicate through intercellular junctions Function: intercellular junctions help coordinate the behavior of all cells in a tissue Types of intercellular junctions: – Tight junctions – Desmosomes Animal cells – Gap junctions – Plasmodesmata (plant cells) Copyright © 2005 Pearson Education, Inc. publishing as Benjamin Cummings Plant cell walls junctions Plasmodesmata: junctions between plant cell walls => Connect neighbouring plant cells - Channels (communicating junctions) Central Plasma => water, small solutes, vacuole of cell membrane Secondary cell wall macromolecules can pass through Primary cell wall Central vacuole Middle => allow molecule exchange of cell lamella 1 µm Central vacuole Cytosol Plasma membrane Plant cell walls Figure 6.28 Plasmodesmata Copyright © 2005 Pearson Education, Inc. publishing as Benjamin Cummings Plant cell junctions: Plasmodesmata Cell walls Interior of cell Interior of cell Figure 6.30 0.5 µm Plasmodesmata Plasma membranes Copyright © 2005 Pearson Education, Inc. publishing as Benjamin Cummings Types of intercellular junctions in animals Three types of intercellular junctions in animal cells: 1. Tight junctions: prevent leakage of fluid across a layer of cells, do not allow molecule exchange (occluding junctions) 2. Desmosomes: fasten cells together into sheets (anchoring junctions), do not allow molecule exchange 3. Gap junctions: serve as channels allowing ions and small molecules across cells => facilitate communication between cells in tissues (communicating junctions) Copyright © 2005 Pearson Education, Inc. publishing as Benjamin Cummings Types of intercellular junctions in animal cells Tight junctions: prevent intercellular communication (molecule exchange) Desmosomes: anchor cells through ECM Gap junctions: - channels between cells - allow molecule exchange between cells Copyright © 2005 Pearson Education, Inc. publishing as Benjamin Cummings Tight junctions prevent leakage of extracellular fluid from a layer of epithelial cells. Fig. 7-32a Tight junction Intermediate filaments Desmosome Gap junctions Extracellular Space matrix between cells Plasma membranes of adjacent cells Copyright © 2005 Pearson Education, Inc. publishing as Benjamin Cummings Desmosomes fasten cells together into strong sheets. Intermediate filaments (made of keratin in epithelial cells) anchor desmosomes in the cytoplasm. Desmosomes attach muscle cells to each other in a muscle. Some ‘muscle Fig. 6-32a tears’ involve the rupture of desmosomes. Tight junction Intermediate filaments Desmosome Gap junctions Extracellular Space matrix Desmosome between 1 µm cellsPlasma membranes of adjacent cells Copyright © 2005 Pearson Education, Inc. publishing as Benjamin Cummings Desmosomes: Intermediate filament role Intermediate filaments participate in cell junction formation (e.g. desmosomes) Copyright © 2005 Pearson Education, Inc. publishing as Benjamin Cummings Epithelial cell desmosome structure Gap junctions: cytoplasmic channels between adjacent cells through which ions, sugars, amino acids and other small molecules may pass. Common in heart muscle and animal embryos. Tight junction Intermediate filaments Desmosome Gap Gap junction junctions 0.1 µm Extracellular Space matrix between cellsPlasma membranes of adjacent cells Copyright © 2005 Pearson Education, Inc. publishing as Benjamin Cummings Intercellular junctions Summary Junction name Cell type Cell communication Plasmodesmata Plant cells Yes Tight junctions Animal cells No Desmosomes Animal cells No Gap junctions Animal cells Yes The Cell: A Living Unit Greater Than the Sum of Its Parts Cells rely on the integration of structures and organelles in order to function Figure 6.325 µm Example: a macrophage’s ability to destroy bacteria involves coordination of the whole cell components such as the cytoskeleton, lysosomes, and plasma membrane https://www.youtube.com/watch?v=Dn3eNoxQdL0 https://www.youtube.com/watch?v=aUtPUuNWCuA Copyright © 2005 Pearson Education, Inc. publishing as Benjamin Cummings Summary Light vs electron microscope Prokaryotic vs eukaryotic cell Plant vs animal cells Subcellular structures and organelles Cytoskeletal fibers and their functions Extracellular components: ECM and plant cell wall Intercellular junctions Copyright © 2005 Pearson Education, Inc. publishing as Benjamin Cummings SBA example Which type of cell junction is only present in plant cells and not in animal cells? A. Plasmodesmata B. Tight junctions C. Desmosomes D. Gap junctions E. Hemidesmosomes Copyright © 2005 Pearson Education, Inc. publishing as Benjamin Cummings Clinical case studies: Case 1 Patient clinical presentation (symptoms): 25-year old male visits the fertility clinic as part of an evaluation of infertility that he is undergoing with his wife Patient history: Frequent sinus infections (sinusitis) and chronic cough with sputum formation Diagnostic methods (labs): Semen analysis reveals immotile spermatozoa Clinical case study analysis Diagnosis (disease): Kartagener’s syndrome Pathogenesis: Lack of dynein from microtubules of the sperm flagella Type of disorder: genetic disorder (autosomal recessive)