Biophysics Lecture Notes - Action Potential
Document Details
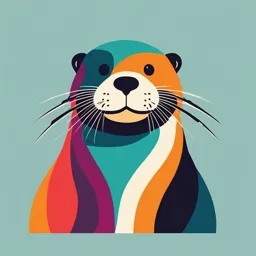
Uploaded by ComfortingAestheticism
University of Debrecen
null
Zoltan Varga
Tags
Summary
This document contains lecture notes on resting potential, action potential, and electrical excitability. It covers the measurement of membrane potential and related concepts.
Full Transcript
Ver. 2, revised November 2021 Resting potential, action potential, and electrical excitability. Measurement of membrane potential. The text under the slides was written by Zoltan Varga...
Ver. 2, revised November 2021 Resting potential, action potential, and electrical excitability. Measurement of membrane potential. The text under the slides was written by Zoltan Varga 2019 Revised in 2021 by Gyorgy Panyi This instructional material was prepared for the biophysics lectures held by the Department of Biophysics and Cell Biology Faculty of Medicine University of Debrecen Hungary https://biophys.med.unideb.hu Ver. 2, revised November 2021 Connection with earlier lectures: resting membrane potential Goldman-Hodgkin-Katz equation Donnan potential Na/K pump The lecture is about the action potential generated by excitable cells: what are the ion channel activities and ion movements that generate the AP, what is its mechanism of propagation and what techniques can be used to measure the cellular membrane potential. 2 Ver. 2, revised November 2021 The previous lecture has introduced the cellular membrane potential (MP). An electric potential difference (= voltage) can be measured between the two sides of the membrane surrounding living cells, which is called membrane potential. The earliest electrophysiological experiments were performed in the late 40’s, early 50’s on the giant axon of the squid, since this was large enough to insert electrodes in it and observe excitability and ion channel operation. The MP is most easily measured by two electrodes, but optical methods also exist for its measurement. 3 Ver. 2, revised November 2021 One advantage of fluorescent dyes is that there is no need to put an electrode into the cell, it can remain intact. In addition, the MP can be measured in many cells simultaneously or within a short time. 4 Ver. 2, revised November 2021 The greatest contribution to the cell’s resting potential comes from the diffusion potential described by the GHK equation. The increase in the permeability of a given ion shifts the MP toward the equilibrium potential of that ion. The Na+ / K+ pump has a direct contribution to the MP since it is electrogenic. This means that the net charge moved in a cycle is not zero. Since 3 Na+ ions are removed and 2 K+ ions are moved into the cell, this means the net removal of a single positive charge, which corresponds to a small continuous outward (hyperpolarizing) current that shifts the MP in the negative direction. The magnitude of this is 2-16 mV depending on the cell type. Thus, if the pumps are blocked the MP immediately shifts in the positive direction by this amount. However, the greater significance of the pumps comes from the fact that these create the uneven ion distributions that serve as the driving force of the diffusion potential (GHK, indirect effect). In the absence of pump function (e.g. inhibition or shortage of ATP) the Na+ and K+ gradients decrease with time and the MP shifts in the positive direction and approaches the value of the Donnan potential. So with active pumps the MP may be -70 mV, while without pump function it will approach -18 mV. The contribution of the Donnan potential is negligible with active pumps, its significance increases at reduced pump function as the cell approaches Donnan equilibrium. 5 Ver. 2, revised November 2021 At given ion concentration gradients the diffusion potential is determined by the instantaneous permeability values (see previous lecture, GHK simulator). 6 Ver. 2, revised November 2021 The AP works by an “all or nothing” principle, which means that stimuli not reaching the threshold do not generate an AP at all, whereas stimuli exceeding the threshold evoke a full-sized AP always with the same amplitude. A stronger stimulus generates more APs that occur with a higher frequency. Since during the propagation of the AP, newer and newer channels are activated along the axon, the signal is constantly amplified, therefore the amplitude does not decrease. 7 Ver. 2, revised November 2021 The shape, duration and amplitude of the AP strongly depends on the cell type. In neurons and skeletal muscle cells APs last a few ms, whereas the length of cardiac APs is much longer, 200-400 ms. But even the same cell types (e.g. neurons) may have different APs depending the number and type of ion channels in their membranes. The AP of cardiac cells usually does not reach such positive values at the depolarization peak as those of the neurons or skeletal muscle cells. 8 Ver. 2, revised November 2021 Action potentials were first observed in the giant axon of the squid. When current is injected into the axon by a stimulating electrode, for sub-threshold stimulations only a weak transient depolarization is the response (blue lines), but if the injected charge is sufficient to shift the MP above the threshold, then the activation of voltage-gated Na+ channels starts the AP. According to their electrochemical gradient, Na+ ions flow into the cell, shifting the MP toward ENa (depolarization). As Na+ channels begin to inactivate (not closing!) and K+ channels begin to open with a delay, the MP starts shifting back in the negative direction (repolarization). When all the Na+ channels are non-conducting, but there’s still an efflux of K+ ions, the MP “overshoots” the resting value and hyperpolarization occurs. This decays with the closure of the K+ channels. 9 Ver. 2, revised November 2021 For an excitable cell to fire an AP, the membrane must be sufficiently depolarized close to the voltage- gated Na+ channels, so that the MP reaches their opening threshold. This depolarization is usually caused by the influx of positive charges (e.g. through neurotransmitter receptor channels). The membrane as a capacitor needs to be charged by a certain amount of charge (Q) to change the MP by a given value. Since Q = I t, the necessary charge can be achieved by a shorter duration combined with larger current, or by a longer duration combined with a smaller current. The green curve above connects those duration – stimulus intensity (current) pairs, which pass just enough charge into the cell to initiate an AP. All points above the curve are suitable for this, while the points below are not. Since a membrane full of transport proteins is not a perfect insulator, a very small current is not enough to evoke an AP even if it is applied for a very long time (e.g. if there is a small hole on the bottom of a pot and we pour water very slowly into it, the water level will never reach a certain level.) The minimal current with which an AP can be evoked with a very long lasting stimulation is called the rheobase. The minimal stimulation time that is required to evoke an AP when applying a current twice the rheobase is called the chronaxie. So the rheobase indicates the lowest point of the curve on the y-axis, while the chronaxie characterizes the steepness of the curve. These two parameters vary among the different cell types and depend on the density and distribution of the ion channels and the geometry of the cell. 10 Ver. 2, revised November 2021 A historical experiment performed on the squid giant axon, which proved the Na+ dependence of the AP. Reduction of the EC Na+ concentration decreases the Na+ gradient and shifts the Na+ equilibrium potential in the negative direction. Due to this a reduced Na+ current flows into the cell during an AP, which decreases the steepness of the upstroke of the AP, and the peak of the AP does not reach such a positive voltage as it would with a normal Na+ gradient. 11 Ver. 2, revised November 2021 Since the flow of charges across the membrane changes the MP, it is difficult to study the voltage- dependent behavior of ion channels. That’s why the voltage-clamp technique was developed, which stabilizes the MP at a given value via a feedback circuit, and at the same time measures the current flowing through the membrane. The potential of the reference electrode immersed in the EC fluid is taken to be 0. The MP is measured with an electrode placed into the cell relative to the reference electrode. The amplifier compares the command potential (desired MP) chosen by us with the measured MP, and injects current into the cell (Im) so that the measured MP should approach the desired value. To change the MP (dV/dt), the capacitance of the membrane needs to be charged, during which the current injected (Im) differs from the ionic current flowing through the membrane (Ii), but at constant MP (dV/dt = 0) the two currents are equal, so the current through the ion channels can be measured at a stable membrane potential. 12 Ver. 2, revised November 2021 If the membrane contains more types of ion channels, the sum of the currents can be observed. In the squid axon the voltage-gated Na+ and K+ channels are activated simultaneously when depolarization is applied by voltage-clamp. The Na+ current flows into the cell (negative current) while the K+ flows out (positive current). If tetrodotoxin (TTX) is applied, which specifically blocks Na+ channels, the Na+ current disappears and the pure K+ current remains. If this is subtracted from the total current, we get the pure Na+ current. 13 Ver. 2, revised November 2021 By depolarizing the membrane with voltage-clamp, the voltage-gated ion channels can be activated. The Na+ conductance quickly rises due to the fast opening of the Na + channels (opening of the red activation gate). Then the conductance quickly drops due to inactivation (closing of the blue inactivation gate). K+ channels are also activated by depolarization, but these open more slowly than the Na+ channels. The K+ channels close as the MP repolarizes. 14 Ver. 2, revised November 2021 The time courses of Na+ and K+ permeabilities (conductances) are shown on the same scale, and the resulting changes in the MP, which form the AP. 15 Ver. 2, revised November 2021 An AP is induced if the MP reaches the opening threshold of the voltage-gated Na+ channels. Some Na+ channels open and according to the electrochemical gradient Na+ ions flow into the cell, which causes further depolarization. This causes the opening of additional Na+ channels (positive feedback – a self-reinforcing process) and thus further Na+ influx. Because of the fast gating of the channels and the positive feedback loop, depolarization occurs very quickly. During depolarization the theoretical maximum value of the MP is the equilibrium potential of Na+ (this would occur at maximal pNa and if for all other permeating ions px=0 were true). This value is usually not reached by the AP, because as it approaches the peak, a fraction of Na+ channels have already inactivated (pNa decreases) and a fraction of K+ channels have already opened (pK >0). As pNa further decreases and pK increases, the MP shifts in the negative direction toward EK according to the GHK equation (repolarization). When all Na+ have inactivated (pNa = 0), but still a number of K+ channels are open (pK > 0), the MP “overshoots” the resting MP and hyperpolarization occurs (theoretical lower limit is EK) until most K+ channels close. 16 Ver. 2, revised November 2021 Schematic representation of the positive feedback regulating Na+ channels: The green connector and the arrowhead, as well as the “+” sign next to it, indicate that the output amplifies the input, i.e., depolarization results in further depolarization and even more Na+ channel openings. 17 Ver. 2, revised November 2021 Schematic representation of the negative feedback regulating K+ channels: The red conector and the flat end, as well as the “” sign next to it, indicate that the output inhibits the input, i.e. the repolarizing K+ current inhibits the depolarization that triggered the opening of the K+ channels and thus the output triggers the closure of the K+ channels. 18 Ver. 2, revised November 2021 Summary of the relationship between AP, ion currents, ion channel gating, and membrane permeability changes. 19 Ver. 2, revised November 2021 Without Na+ channel inactivation, the MP would stay continuously at a positive value due to the positive feedback, and the individual APs would not be separated. While the Na + channels are inactivated, they cannot be activated again, which introduces a natural “break” between subsequent APs. This is called the absolute refractory period (ARP). The recovery of Na + channels from inactivation occurs at sufficiently negative membrane potentials. During the hyperpolarized period the Na+ channels can already be activated again, but since the MP is more negative than the resting value, the membrane needs a stronger than normal depolarization to reach the opening threshold and start a new AP. This is the relative refractory period. 20 Ver. 2, revised November 2021 B1 A1 A2 A3 B2 As the AP propagates, it always activates new resting channels and because the equilibrium potentials of the permeating ions are the same along the axon, the generated AP keeps its amplitude and shape all along its propagation. If a depolarization is created at one point of the axon, the electric potential decays exponentially with the distance as it passively propagates. Figure A1 shows that the depolarization (red zone) creates depolarization in the direction of propagation of the AP (red/yellow mixing). The depolarization has not reached the measuring electrode therefore it shows the resting potential. If the voltage-gated Na+ channels are close enough so that the signal hasn’t weakened too much and they can be activated, then they amplify the signal and pass it on. This is in A2, the depolarization reaches the measuring electrode, peak of the AP is reached. Depolarized membrane segment is in the forward direction (yellow/red), black forward arrows show how Na+ entering the axon depolarizes the nearby membrane section. The ARP ensures the one-way propagation (rectification) of the AP, since behind the AP the Na+ channels are still inactivated so propagation cannot occur in that direction. This axon segment is indicated by dark brown color. Panel A3 shows that the AP has passed the recording electrode and the membrane is in the after-hyperpolarization phase, in the RRP. Panels B1 and B2 show two snapshots of the AP on the same axon as it propagates. The black arrows inside the axon show how Na+ entering spreads the depolarization in the nearby axon segments. AP can only be generated in the direction of the resting membrane (segment 3). The propagation speed of the electric signal is faster in thicker axons and those insulated by a myelin sheath 21 Ver. 2, revised November 2021 AP propagates point-by-point along the axon of an unmyelinated axon. This is quite slow as time is required for the activation of the channels and the currents to change the membrane potential. Speed of the propagation can be increased if the red/yellow depolarized region of the axon shown in the previous slide was longer (e.g. in A1), i.e., the depolarization of the axon where the AP is could potentially spread over longer distance within the axon. In this case ion channels must only be present at a long distance from the position of the AP on panel A1 (at t=0), previous slide, to relay the AP to the next relay station. The structure of the myelinated nerve is compatible with these requirements: o thick insulation by the myelin sheathdepolarization can spread to farther distances o Ranvier nodes accumulate ion channels and thus create the relay stations. The axons of some neurons are covered by a lipid-rich myelin sheath, which is formed by glial cells in the central NS and by Schwann-cells in the peripheral NS. This is a good insulator and due to its thickness it decreases the capacitance of the membrane. Because of this, the electric signal (depolarization) can spread to farther distances passively. Thus, the continuous presence of Na + channels in the axon membrane is not required, only at larger distances (up to 1.5 mm), where they act as relay stations amplifying the signal. These are the Ranvier nodes, where the myelin sheath is missing and the channels are present in large numbers. This way the electric signal does not propagate continuously, but instead “jumps” between the nodes and is amplified over and over again. This is called saltatory conduction. Its main advantage is that it is much faster than the continuous propagation because the passive spreading of the electric signal is very fast and it does not require the continuous activation of the channels all along the axon, which is a much slower 22 Ver. 2, revised November 2021 process. Its other advantage is that the propagation is much more economical, since fewer ions cross the membrane passively, therefore the pumps must use less ATP to restore the gradients. Multiple sclerosis is an autoimmune disease, in which the immune system attacks and damages the myelin sheath covering the axons in the brain and spinal cord. Due to the reduced insulation the signal cannot passively propagate from one relay station to the other (Ranvier nodes) so the signal propagation slows down or eventually stops and cannot be passed on to the next cell. This causes neurological symptoms of variable severity. The AP propagating in the neuron is passed on to the postsynaptic cell at the synapse. At the axon terminal neurotransmitters are released due to the AP, which bind to the receptors on the postsynaptic cell and modify the cell’s function. Review questions What techniques can be used to measure the membrane potential? What is the action potential, what are its properties and how does it propagate? What ion cannel gating steps occur and what is the timing of these? What ion fluxes occur and how do these modify the membrane potential? What feedback loops operate during the AP? What are the refractory periods and what causes them? 23 Topic 19: Resting potential, action potential, and electrical excitability. Measurement of membrane potential. Minimals: 1. Give the definition and unit of conductivity! 2. Give the current carried by ion x through the cell membrane if the membrane potential is Em! 3. Define the action potential and interpret the ionic basis of its generation. 4. How do the concentrations of Na+ and K+ in the intra- and extracellular spaces change during a single action potential? 5. What causes the abrupt membrane potential changes during an action potential in a neuron? 6. What is the role of the Na+/K+ ATPase in the membrane potential changes during a single action potential? Why? 7. What is the principle of voltage-clamp? 8. List the methods that can be used for measuring the membrane potential of living cells! 9. What kind of currents can be measured by patch-clamp? 10. What are the most important features of ion channels? True or False: 1) The Na+/K+ ATPase is responsible for the depolarization phase of the action potential. 2) During a single action potential the intracellular concentration of Na+ significantly increases. This document was created by The Debrecen Tutor. Check us out on insta @TheDebrecenTutor 3) The Na+/K+ ATPase is responsible for the restoration of the resting membrane potential in the repolarization phase of the action potential. Essay: 1. a. Define action potential in your own words, what are the 2 most important cell types that can fire action potential? What is the function of the Aps in these cells? (3p) b. Draw a neural action potential, label the measured quantities on the 2 axes and approximate values of the measures quantities. (3p) c. Identify the phases of the neural AP, and explain the ionic background of these phases (which ion channels are in which state, what causes it). (5p) d. Explain why the quantity measured on the vertical axis of the AP figure reaches a min + max value (why it cannot go below and above these values), what determines this values. (2p) e. Explain in a few sentences why AP travels in one direction only along on axon, what prevents background propagation. (2p) 2. a)Draw the typical action potential of a neuron in a coordinate system. Label the measured quantities on the quantities on the axes and indicate their approximate values. b) Explain what gating steps occur in a sodium channel during an AP (what state they start from and how they get back to that state) and how these are related to the membrane potential and flow of sodium ions (cause and consequences). (5p) c) Based on your answer above, explain the feedback loop that sodium channels accomplish. Is it positive or negative feedback? d) How does the strength of the stimulus affect the generation and properties (amplitude, shape) of the action potential? Fill in questions: An action potential can be initiated when the cell membrane is ………………. with respect to the resting potential. This causes the opening of ………….. channels, which are ………………. gated. The membrane potential quickly shifts to about ………………….. mV due to the …………………. flow of cations. The transition of these channels into a(n) ………………….. state and the delayed opening of voltage-gated ……………………. channels causes the membrane potential to return close to the This document was created by The Debrecen Tutor. Check us out on insta @TheDebrecenTutor resting value. The time interval during which another action potential cannot be initiated regardless of the stimulus strength is called ………………………. period. 0 120 depolarized Cl- Na+ cAMP Absolute refractory outward Inhibited K+ inactivated chronaxie cGMP open Ca2+ ligand Relative refractory hyperpolarized -80 +50 inward voltage rheobase Membrane stretch repolarized Unstable Uneven closed Fill-in questions: ……………………..-gated …………………………… (the name of the ion) channels are responsible for the depolarization phase of the action potential. Relation analysis: 1) Ionophores have a physiological role during the action potentials of muscle cells because they promote the transfer of ions across lipid membranes. 2) During an action potential a "positive after potential" can be obsereved because in the regeneration phase the voltage gated potassium channels have a delayed closing. 3) The strength-duration curve represents the minimum charge required to reach the action potential threshold because charge can be calculated as a ratio of the duration and amplitude of the current pulse. 4) Rheobase is the minimal current amplitude of indefinite duration that results in the depolarization threshold required to initiate an action potential because initiation of the action potential is based on the opening of voltage-gated potassium channels. 5) Q: In a typical action potential the spike is due to a large sodium influx through voltage-gated sodium channels, because the excitation signal (depolarization) does not activate the voltage gated potassium channels. Q: Analyze an action potential diagram, what kind of feedback control can be found during the process; specify the regions of the action potential curve where these feedback mechanisms operate. This document was created by The Debrecen Tutor. Check us out on insta @TheDebrecenTutor Q: Explain the development of Donnan potential. Q: What is the ionic background of the resting membrane potential? Q: What is the ionic background of action potential? How does it spread? Q: What kinds of positive and negative feedback mechanisms are operational in the generation of an action potential? Q: How does the Na+/K+ ATPase work? Q: What is the contribution of the Na+/K+ ATPase to the maintenance of the resting membrane potential? (Hint: there is more than one!) True or false: Q: The resting membrane potential of living cells: 1. Is about -90mV (for excitable cells) 2. Is dominated by the Donnan potential (equilibrium) 3. Is independent of the intracellular metabolism 4. Substantially decreases upon treatment of cells with ionophore molecules facilitating sodium and potassium diffusion (transport) across the plasma membrane 5. Is dominated by the diffusion potential generated by the mobility difference between positive and negative ions 6. Is about +40mV Q: In a typical AP the spike is due to a large potassium influx through voltage gated potassium channels, because the excitation signal (depolarization) has no influence on the voltage gated Na channel. Essay: 1a) Draw an action potential. Label the measured quantities properly on both axes. Indicate the approximate values with their proper units on the graph. (4p) This document was created by The Debrecen Tutor. Check us out on insta @TheDebrecenTutor b) Explain the ionic background of the generation of an action potential. Mention the types of ion channels involved and the sequence of their gating steps. Describe the positive and negative feedback regulations involved. (9p) Multiple choice 1) Action potential a) The depolarization phase of the action potential is governed by increase in the K+ permeability of the membrane b) Activation of voltage-gated K+ channels and the concomitant K+ flux strengthens the depolarization caused by the Na+ ions. c) The influx of Na+ increases the cytosolic Na+ concentration to such an extent that it will exceed the intracellular K+ concentration. d) Inactivation of Na+ channels decreases the Na+ permeability of the membrane during the repolarization phase. e) K+ channels are regulated by the membrane potential by means of negative feedback 2) This document was created by The Debrecen Tutor. Check us out on insta @TheDebrecenTutor