Thermodynamics (Chemistry) Lecture Companion PDF
Document Details
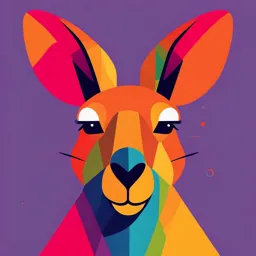
Uploaded by AttractiveSpatialism
Silver Oak University
Prof.Pranjal Chauhan
Tags
Summary
This document is a lecture companion on thermodynamics, suitable for undergraduate students of chemistry. It covers fundamental concepts and principles in chemistry, as well as solved problems. The author is Prof. Pranjal Chauhan.
Full Transcript
SUBJECT (CHEMISTRY) LECTURE COMPANION SEMESTER: I/II PREPARED BY: Prof.Pranjal Chauhan CHAPTER 1 PHYSICAL CHEMISTRY 1.2 THERMODYNAMICS 1.2.1...
SUBJECT (CHEMISTRY) LECTURE COMPANION SEMESTER: I/II PREPARED BY: Prof.Pranjal Chauhan CHAPTER 1 PHYSICAL CHEMISTRY 1.2 THERMODYNAMICS 1.2.1 Introduction 1.2.2 Basic Thermodynamics Terms 1.2.3 Zeroth Law of Thermodynamics 1.2.4 First Law of Thermodynamics 1.2.5 Concept of Enthalpy 1.2.6 Reversible Expansion 1.2.7 Concept of Heat Capacity 1.2.8 Second Law of Thermodynamics & Entropy 1.2.9 Third Law of Thermodynamics & Absolute Entropy 1.2.10 Hess’s Law *Proprietary material of SILVER OAK UNIVERSITY Page | 1 SUBJECT (CHEMISTRY) LECTURE COMPANION SEMESTER: I/II PREPARED BY: Prof.Pranjal Chauhan THERMODYNAMICS 1.2.1 Introduction: The branch of physics / physical chemistry which deals with heat, work, and temperature, and their relation to energy, radiation, and physical properties of matter is called Thermodynamics. Branches of Thermodynamics includes: Classical thermodynamics Statistical mechanics Chemical thermodynamics Equilibrium thermodynamics Scope of Thermodynamics: Most of the important laws of Physical Chemistry, including the van’t Hoff law of lowering of vapour pressure, Phase Rule and the Distribution Law, can be derived from the laws of thermodynamics. It tells whether a particular physical or chemical change can occur under a given set of conditions of temperature, pressure and concentration. It also helps in predicting how far a physical or chemical change can proceed, until the equilibrium conditions are established. Limitations of Thermodynamics: Thermodynamics is applicable to macroscopic systems consisting of matter in bulk and not to microscopic systems of individual atoms or molecules. It ignores the internal structure of atoms and molecules. Thermodynamics does not bother about the time factor. That is, it does not tell anything regarding the rate of a physical change or a chemical reaction. It is concerned only with the initial and the final states of the system. Thermodynamics is principally based on a set of four laws which are universally valid when applied to systems that fall within the constraints implied by each. Laws of thermodynamics: *Proprietary material of SILVER OAK UNIVERSITY Page | 2 SUBJECT (CHEMISTRY) LECTURE COMPANION SEMESTER: I/II PREPARED BY: Prof.Pranjal Chauhan 1. Zeroth Law of Thermodynamics 2. First Law of Thermodynamics 3. Second Law of Thermodynamics 4. Third Law of Thermodynamics 1.2.2 Basic Thermodynamic Terms: System & Surroundings: System is that part of the universe which is under thermodynamic study and the rest of the universe is surroundings. Boundary: The real or imaginary surface separating the system from the surroundings is called the boundary. Homogeneous System: When a system is uniform throughout, it is called a Homogeneous System. Heterogeneous system: A heterogeneous system is one which consists of two or more phases. Homogeneous System Heterogeneous System *Proprietary material of SILVER OAK UNIVERSITY Page | 3 SUBJECT (CHEMISTRY) LECTURE COMPANION SEMESTER: I/II PREPARED BY: Prof.Pranjal Chauhan Open System: An open system is one which can transfer both energy and matter to and from its surroundings. Closed System: A closed system is one which cannot transfer matter but can transfer energy in the form of heat, work and radiation to and from its surroundings. Isolated System: An isolated system is one that can transfer neither matter nor energy to and from its surroundings. Intensive Property: A property which does not depend on the quantity of matter present in the system is known as Intensive Property. (Examples: Temperature, Pressure, Density, Boiling Point, Surface Tension, Refractive Index, Viscosity, Freezing Point, etc.) Extensive Property: A property that does depend on the quantity of matter present in the system is called an Extensive Property. (Examples: Mass, Volume, Internal Energy, Enthalpy, Entropy, etc.) The extensive properties are additive while intensive properties are not. Isothermal Process: Those processes in which the temperature remains fixed, are termed isothermal processes (dT = 0). Adiabatic Process: Those processes in which no heat can flow into or out of the system, are called adiabatic processes (dq = 0). Isobaric Process: Those processes which take place at constant pressure are called isobaric processes (dP = 0). Isochoric Process: Those processes in which the volume remains constant are known as isochoric processes (dV = 0). *Proprietary material of SILVER OAK UNIVERSITY Page | 4 SUBJECT (CHEMISTRY) LECTURE COMPANION SEMESTER: I/II PREPARED BY: Prof.Pranjal Chauhan Cyclic Process: When a system in a given state goes through a number of different processes and finally returns to its initial state, the overall process is called a cycle or cyclic process (dE = 0, dH = 0). Spontaneous Process: A process which proceeds of its own accord, without any outside assistance, is termed a spontaneous or natural process. Nonspontaneous Process: The reverse process which does not proceed on its own is referred to as a nonspontaneous or unnatural process. Spontaneity: The tendency of a process to occur naturally is called the spontaneity. Criteria of Spontaneity: 1. A spontaneous change is one-way or unidirectional. (For reverse change to occur, work has to be done.) 2. For a spontaneous change to occur, time is no factor. (A spontaneous reaction may take place rapidly or very slowly.) 3. If the system is not in equilibrium state (unstable), a spontaneous change is inevitable. (The change will continue till the system attains the state of equilibrium.) 4. Once a system is in equilibrium state, it does not undergo any further spontaneous change in state if left undisturbed. (To take the system away from equilibrium, some external work must be done on the system.) 5. A spontaneous change is accompanied by decrease of internal energy or enthalpy (ΔH). 1.2.3 Zeroth Law of Thermodynamics: Statement: If two thermodynamic systems are in thermal equilibrium with a third, they are also in thermal equilibrium with each other. *Proprietary material of SILVER OAK UNIVERSITY Page | 5 SUBJECT (CHEMISTRY) LECTURE COMPANION SEMESTER: I/II PREPARED BY: Prof.Pranjal Chauhan A generalized statement about bodies in contact at thermal equilibrium and is the basis for the concept of temperature. Given by: Ralph H. Fowler When two objects are separately in thermodynamic equilibrium with a third object, they are in equilibrium with each other. Objects in thermodynamic equilibrium have the same temperature. A system in thermal equilibrium is a system whose macroscopic properties* (like pressure, temperature, volume, etc.) are not changing in time. *Macroscopic Properties: Macroscopic properties of a matter are the properties in bulk matter, can be visible to naked eye, i.e. Pressure, Temperature, Density, Volume, etc. Two systems are said to be in thermal equilibrium when, there is no net flow of thermal energy between them when they are connected by a path permeable to heat. Two substances/systems are said to be in thermal equilibrium if when separated by a diathermic wall, there is no net energy transfer between them. A system is said to be in thermodynamic equilibrium, if there is no net change in it’s macroscopic properties with time. A system is said to be in thermodynamic equilibrium if it is in mechanical, chemical and thermal equilibrium. Systems in thermodynamic equilibrium are always in thermal equilibrium, but the converse is not always true. *Proprietary material of SILVER OAK UNIVERSITY Page | 6 SUBJECT (CHEMISTRY) LECTURE COMPANION SEMESTER: I/II PREPARED BY: Prof.Pranjal Chauhan 1.2.4 First Law of Thermodynamics: Statement: The total energy of an isolated system remains constant though it may change from one form to another. Explanation using Example: When a system is changed from state A to state B, it undergoes a change in the internal energy from EA to EB. Thus, ∆E = EB – EA This energy change is brought about by the evolution or absorption of heat and/or by work being done by the system. Mathematical Equation: ΔE = q – W Where, q = the amount of heat supplied to the system W = work done by the system Consider the system of ‘expanding hot gas’ The gas expands against an applied constant pressure by volume ΔV. The total mechanical work done is given by the relation, W = P × ΔV ΔE = q – (P × ΔV) Other Statements for First Law of Thermodynamics: 1. The net energy change of a closed system is equal to the heat transferred to the system minus the work done by the system. 2. Whenever energy of a particular type disappears equivalent amount of another type must be produced. 3. Total energy of a system and surroundings remains constant. *Proprietary material of SILVER OAK UNIVERSITY Page | 7 SUBJECT (CHEMISTRY) LECTURE COMPANION SEMESTER: I/II PREPARED BY: Prof.Pranjal Chauhan 4. It is impossible to construct a perpetual motion machine that can produce work without spending energy on it. Special Forms of First Law of Thermodynamics: 1. For a cyclic process involving isothermal expansion of an ideal gas, ΔE = 0 q=W 2. For an isochoric process (no change in volume) there is no work of expansion, W = 0 ΔE = qv 3. For an adiabatic process there is no change in heat gained or lost, q = 0 ΔE = - W 1.2.5 Concept of Enthalpy: Definition: The total heat content of a system at constant pressure is equivalent to the internal energy E plus the PV energy. Enthalpy = en (in) + thalpos (heat) [Greek meaning] Mathematical Expression: H = E + PV Change in Enthalpy: If ΔH be the difference of enthalpy of a system in the final state (H2) and that in the initial state (H1), ΔH = H2 – H1 ΔH = (E2 + P2V2) – (E1 + P1V1) ΔH = (E2 – E1) + (P2V2 - P1V1) *Proprietary material of SILVER OAK UNIVERSITY Page | 8 SUBJECT (CHEMISTRY) LECTURE COMPANION SEMESTER: I/II PREPARED BY: Prof.Pranjal Chauhan ΔH = (ΔE) + (ΔPV) If P is constant while the gas is expanding, then ΔH = (ΔE) + (PΔV) ΔH = (ΔE) + W According to the First Law, ΔE = q – W ΔH = q, when change in state occurs at constant pressure ΔH = qp Sign Conventions: ΔH is positive if H2 > H1 and the process or reaction will be endothermic. ΔH is negative if H2 < H1 and the process or reaction will be exothermic. ΔH = Hproducts – Hreactants = qp Units: kilocalories (kcal) or kilojoules (kJ) 1.2.6 Reversible Expansion: Consider an ideal gas confined in a cylinder with a frictionless piston. Suppose it expands in a reversible manner from volume V1 to V2 at a constant temperature. The pressure of the gas is successively reduced from P1 to P2. The reversible expansion of the gas takes place in a finite number of infinitesimally small intermediate steps. Providing external pressure (Pext) that equals the internal pressure (gas pressure) (Pgas) keeping the piston stationary. *Proprietary material of SILVER OAK UNIVERSITY Page | 9 SUBJECT (CHEMISTRY) LECTURE COMPANION SEMESTER: I/II PREPARED BY: Prof.Pranjal Chauhan If Pext is decreased by an infinitesimal amount dP the gas expands reversibly and the piston moves through a distance dL. Pext = Pgas = P Work done by the gas can be expressed by dW, dW = P x A x dL dW = P x dV (dV = A x dL) The total amount of work done by the isothermal reversible expansion of the ideal gas from V1 to V2 is, 𝑉2 W = − ∫ 𝑃 · 𝑑𝑉 𝑉1 But according to ideal gas equation (PV = nRT), 𝑛𝑅𝑇 𝑃= 𝑉 𝑉2 𝑛𝑅𝑇 W=−∫ · 𝑑𝑉 𝑉 𝑉1 𝑉2 1 W = − 𝑛𝑅𝑇 ∫ · 𝑑𝑉 𝑉 𝑉1 𝑉2 W = − 𝑛𝑅𝑇 ln 𝑉1 But as we know, P1V1 = P2V2 𝑉1 𝑃2 = 𝑉2 𝑃1 *Proprietary material of SILVER OAK UNIVERSITY Page | 10 SUBJECT (CHEMISTRY) LECTURE COMPANION SEMESTER: I/II PREPARED BY: Prof.Pranjal Chauhan 𝑃1 W = − 𝑛𝑅𝑇 ln 𝑃2 𝑃1 W = −2. 303 · 𝑛𝑅𝑇 𝑙og 𝑃2 Isothermal compression work of an ideal gas may be derived similarly and it has exactly the same value with the sign changed. Pressure on the piston, Pext, is increased by dP which reduces the volume of the gas. 𝑃1 W = 2. 303 · 𝑛𝑅𝑇 𝑙og 𝑃2 1.2.7 Concept of Heat Capacity: Definition: Heat absorbed by unit mass in raising the temperature by one degree (K or º C) at a specified temperature. Mathematical Expression: If Q calories is the heat absorbed by mass m and the temperature rises from T1 to T2, the heat capacity (c) is given by, O 𝑐= 𝑚 × (𝑇2 − 𝑇1) If mass is considered as 1 mol, O O 𝐶= = (𝑇2 − 𝑇1) ∆𝑇 Where, C = molar heat capacity = the amount of heat required to raise the temperature of one mole of the substance (system) by 1 K. Heat capacity (C) varies with temperature so its true value will be given as, 𝑑O 𝐶= 𝑑𝑇 Unit: cal K-1 mol-1 or J K-1 mol-1 Heat is not a state function, neither is heat capacity. *Proprietary material of SILVER OAK UNIVERSITY Page | 11 SUBJECT (CHEMISTRY) LECTURE COMPANION SEMESTER: I/II PREPARED BY: Prof.Pranjal Chauhan The two important types of molar heat capacities are those: 1. At constant volume 2. At constant pressure Molar Heat Capacity at Constant Volume: According to the first law of thermodynamics, 𝑑q = 𝑑𝐸 + 𝑝 · 𝑑𝑉 Dividing both sides by dT and applying the conditions, 0 𝑑q 𝑑𝐸 + 𝑝 · 𝑑𝑉 𝑑𝐸 = = ( ) = 𝐶𝑉 𝑑𝑇 𝑑𝑇 𝑑𝑇 𝑉 Definition: Rate of change of internal energy with temperature at constant volume. Molar Heat Capacity at Constant pressure: According to the first law of thermodynamics, 𝑑q 𝑑𝐸 𝑝 · 𝑑𝑉 =𝐶= + 𝑑𝑇 𝑑𝑇 𝑑𝑇 But as we know, H = E + PV so differentiating this equation w.r.t. T keeping pressure constant 𝑑𝐻 𝑑𝐸 𝑑𝑉 ( ) =( ) + 𝑃 · ( ) = 𝐶𝑝 𝑑𝑇 𝑝 𝑑𝑇 𝑝 𝑑𝑇 𝑝 Definition: Rate of change of enthalpy with temperature at constant pressure. Relation between CP & CV: 𝑑𝐻 ( ) = 𝐶𝑝 𝑑𝑇 𝑝 𝑑𝐸 ( ) = 𝐶𝑉 𝑑𝑇 𝑉 *Proprietary material of SILVER OAK UNIVERSITY Page | 12 SUBJECT (CHEMISTRY) LECTURE COMPANION SEMESTER: I/II PREPARED BY: Prof.Pranjal Chauhan By definition, H = E + PV for 1 mole of an ideal gas and thus, H = E + RT Differentiating it w.r.t. Temperature T, 𝑑𝐻 𝑑𝐸 = +𝑅 𝑑𝑇 𝑑𝑇 𝐶𝑝 = 𝐶𝑉 + 𝑅 𝐶𝑝 − 𝐶𝑉 = 𝑅 Cp is greater than CV by a gas constant whose value is 1.987 cal K–1 mol–1 or 8.314 J K–1 mol–1. 1.2.8 Second Law of Thermodynamics & Entropy: Statement 1: Whenever a spontaneous process takes place, it is accompanied by an increase in the total energy of the universe (ΔSuniverse > 0). ΔSuniverse = ΔSsystem + ΔSsurroundings When a reversible process occurs, the entropy of the system remains constant. (ΔSuniverse = 0) Statement 2: The entropy of the system is constantly increasing. Entropy: Definition: A thermodynamic state quantity that is a measure of the randomness or disorder of the molecules of the system. Symbol: S Units: cal K-1 mol-1 or J K-1 mol-1 or eu (entropy units) A process accompanied by an increase in entropy tends to be spontaneous. 1.2.9 Third Law of Thermodynamics & Absolute Entropy: Statement: At absolute zero, the entropy of a pure crystal is also zero. *Proprietary material of SILVER OAK UNIVERSITY Page | 13 SUBJECT (CHEMISTRY) LECTURE COMPANION SEMESTER: I/II PREPARED BY: Prof.Pranjal Chauhan That is, S = 0 at T = 0 K. Absolute & Standard Entropy: Absolute Entropy: Actual amount of entropy that a substance possesses at any temperature above 0 K is termed as absolute entropy. Standard Entropy: The absolute entropy of a substance at 25ºC (298 K) and one atmosphere pressure is called the standard entropy, So. Absolute entropy of elements is zero only at 0 K in a perfect crystal. Standard entropies of all substances at any temperature above 0 K always have positive values. Once we know the entropies of a variety of substances, we can calculate the standard entropy change, ΔSº, for chemical reactions. ΔSº = ΣSº(products) – ΣSº(reactants) 1.2.10 Hess’s Law: Statement: If a chemical change can be made to take place in two or more different ways whether in one step or two or more steps, the amount of total heat change is same no matter by which method the change is brought about. 𝐴 → 𝐷 + 𝑄1 ∆𝐻 = −𝑄1 𝐴 → 𝐵 + 𝑞1 ∆𝐻1 = −𝑞1 𝐵 → 𝐶 + 𝑞2 ∆𝐻2 = −𝑞2 *Proprietary material of SILVER OAK UNIVERSITY Page | 14 SUBJECT (CHEMISTRY) LECTURE COMPANION SEMESTER: I/II PREPARED BY: Prof.Pranjal Chauhan 𝐶 → 𝐷 + 𝑞3 ∆𝐻3 = −𝑞3 ∆𝐻 = ∆𝐻1 + ∆𝐻2 + ∆𝐻3 Hess' Law of Constant Heat Summation is useful in the determination of enthalpies of the following: Heats of formation of unstable intermediates like CO(g) and NO(g). Heat changes in phase transitions and allotropic transitions. Lattice energies of ionic substances by constructing Born-Haber cycles if the electron affinity to form the anion is known. Electron affinities using a Born-Haber cycle with a theoretical lattice energy Solved Numerical 1: Statement: Calculate the heat of formation of potassium hydroxide from the following data. Reaction No. Reaction ΔH, kcal 1 1 𝐾(𝑠) + 𝐻2𝑂(𝑎𝑞) → 𝐾𝑂𝐻(𝑎𝑞) + 𝐻2(g) - 48.0 2 1 2 𝐻2(g) + 𝑂2(g) → 𝐻2𝑂(𝑙) - 68.5 2 3 𝐾𝑂𝐻(𝑠) → 𝐾𝑂𝐻(𝑎𝑞) - 14.0 Solution: Reaction No. Reaction ΔH, kcal 1 1 𝐾(𝑠) + 𝐻2𝑂(𝑎𝑞) → 𝐾𝑂𝐻(𝑎𝑞) + 𝐻2(g) - 48.0 1 2 𝐻 𝑂 →𝐻 𝑂 2 2(g) + 2 2(g) 2 (𝑙) - 68.5 3 𝐾𝑂𝐻(𝑠) → 𝐾𝑂𝐻(𝑎𝑞) - 14.0 Reverse *Proprietary material of SILVER OAK UNIVERSITY Page | 15 SUBJECT (CHEMISTRY) LECTURE COMPANION SEMESTER: I/II PREPARED BY: Prof.Pranjal Chauhan Reaction No. Reaction ΔH, kcal 1 1 𝐾 +𝐻 𝑂 → 𝐾𝑂𝐻 + 𝐻 - 48.0 (𝑠) 2 (𝑎𝑞) (𝑎𝑞) 2 2(g) 1 𝐻 𝑂 →𝐻 𝑂 2 2(g) + 2 2(g) 2 (𝑙) - 68.5 3 𝐾𝑂𝐻(𝑎𝑞) → 𝐾𝑂𝐻(𝑠) + 14.0 Do the summation of the enthalpies, ΔH = (- 48.0) + (- 68.5) + 14.0 = - 102.5 kcal What will you do? Statement: Determine ΔH of the reaction, 𝐶(𝑠) + 2𝐻2(g) → 𝐶𝐻4(g) Reaction No. Reaction ΔH, kJ 1 𝐶(𝑠) + 𝑂2(g) → 𝐶𝑂2(g) - 393.7 1 2 𝐻2(g) + 𝑂2(g) → 𝐻2𝑂(𝑙) - 285.7 2 3 𝐶𝐻4(g) + 2𝑂2(g) → 𝐶𝑂2(g) + 2𝐻2𝑂(𝑙) - 890.3 Answer: - 74.8 kJ Check Your Knowledge: 1. A thermos flask is an example of *Proprietary material of SILVER OAK UNIVERSITY Page | 16 SUBJECT (CHEMISTRY) LECTURE COMPANION SEMESTER: I/II PREPARED BY: Prof.Pranjal Chauhan (A) Isolated System (C) Open System (B) Closed System (D) Heterogeneous System 2. Zinc granules reacting with dilute hydrochloric acid in an open beaker constitutes (A) Isolated System (C) Closed System (B) Open System (D) None of the above 3. A gas expands from 10 litres to 20 litres against a constant external pressure of 10 atm. The pressure volume work done by the system is (A) 100 lit atm (C) – 100 lit atm (B) – 10 lit atm (D) 10 lit atm 4. Which of the following statements is not correct? (A) Heat is not a state function (C) Neither of these (B) Heat capacity is not a state (D) Both function 5. The enthalpy change of a reaction is independent of (A) State of the reactants and products (C) Initial and final enthalpy change of the reaction (B) Nature of the reactants and (D) Different intermediate reaction products Answers: (1) A (2) B (3) C (4) C (5) A -------- X ------------------ X ------------------ X ------------------ X ------------------ X -------- *Proprietary material of SILVER OAK UNIVERSITY Page | 17