Thermodynamics PDF
Document Details
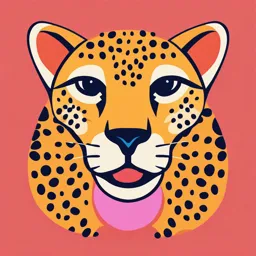
Uploaded by NimbleGravity7619
Tags
Summary
This document covers the fundamental concepts of thermodynamics, including thermal equilibrium, internal energy, and thermodynamic systems. It also explores various types of thermodynamic systems, such as open, closed, and isolated systems, and explains the different ways in which the internal energy of a system can be changed.
Full Transcript
Thermodynamics The engine of a vehicle as well as our muscles do some work and both produce some heat both objects reach the same temperature after some time. Thermodynamics is the branch of physics that deal...
Thermodynamics The engine of a vehicle as well as our muscles do some work and both produce some heat both objects reach the same temperature after some time. Thermodynamics is the branch of physics that deals with the concepts of heat and temperature and the inter-conversion of heat and other forms of energy Thermal Equilibrium When a piece of ice is kept in water at room temperature, the ice melts. This is because the water at room temperature (higher than the ice temperature) transfers its heat to ice and helps ice melt. Similarly, when hot water is mixed with cold water, it transfers its heat to the cold water. The hot water cools down. In both these examples, we notice that the two components reach a stage where there is no more transfer of heat. In such cases, we assume that heat is something that is transferred from a substance at a higher temperature to that at a lower temperature. This transfer continues till the level of heat content in both the substances is the same. Then we say that a thermal equilibrium is reached between the two substances. We can say that when two objects are at the same temperature, they are in thermal equilibrium. Thermal equilibrium: Two systems in thermal contact with each other are in thermal equilibrium if they do not transfer heat between each other. Laws of thermodynamics Zeroth Law of Thermodynamics “If two systems are each in thermal equilibrium with a third system, they are also in thermal equilibrium with each other” The same principle is used to measure temperature of an object When the thermometer and the object are in thermal equilibrium, the thermometer indicates the temperature of the object. This law also enables us to use a thermometer to compare the temperatures of different objects. Internal Energy: Every system consists of a large number of molecules. The energy associated with the random, disordered motion of the molecules of a system is called Internal energy. Thermodynamic system collection or a group of objects that have ability to exchange energy with its surroundings A thermodynamic system is a collection or a group of objects that can form a unit which may have ability to exchange energy with its surroundings. Anything that is not a part of the system is its surrounding or its environment Types of Thermodynamic systems An open system is a system that freely allows exchange of energy and matter with its environment. For example, water boiling in a kettle is an open system. Heat escapes into the air. This is the exchange of energy with the surroundings. At the same time, steam also escapes into the air. This is exchange of matter with the surroundings. A closed system, on the other hand, does not allow the exchange of matter but allows energy to be transferred. For example, water boiling in a boiler is a closed system. It allows heat to be transferred from the source of heat (a burner) to the water (system) inside. Similarly, heat is also transferred to the surroundings. Steam (matter) is not allowed to escape as long as the valve is kept closed. An isolated system is completely sealed (isolated from its environment). Matter as well as heat cannot be exchanged with its environment. A thermos flask is a very familiar example of an isolated system. Thermodynamic Process A process in which the thermodynamic state of a system is changed is called a thermodynamic process. For example, when water contained in a vessel is heated externally, water starts boiling after some time and steam is produced which exerts pressure on the walls of the vessel. In this case, the state of the water in the container is changed. This is because, the temperature (T), the volume (V ), and the pressure (P ) of the water inside the vessel change when it starts boiling. Thus, we can describe the state of a system by using temperature, pressure and volume as its variables. Heat Consider a glass filled with water on a table Let 𝑻𝑺 𝐚𝐧𝐝 𝑻𝑬 be the temperatures of the system and its environment respectively. Let Q be the energy transferred between the system and its environment. Change in Internal Energy of a System Figure shows a cylinder filled with some gas in it. This cylinder is provided with a movable, massless, and frictionless piston at one end. The gas inside the cylinder is system and the rest is its environment. Let the temperature of the gas be TS and that of the environment be TE. Internal energy of the system (the gas) can be changed in two different ways or by both. The cylinder can be brought in contact with a source of heat such as a burner as shown in Figure. The temperature difference between the source of heat (environment) and the system will cause a flow of energy (heat) towards the gas in the cylinder. This is because TE > TS. Thus, there will be an increase in the internal energy of the gas. Such exchange of energy is possible in another way also. If the surrounding is at temperature lower than the gas, TS > TE, the gas will lose energy to its environment and cool down. The other way to increase the internal energy of the gas is to quickly push the piston inside the cylinder, so that the gas is compressed, as shown in Figure. In this case, we know that the piston does some work on the gas in moving it through some distance. The gas gains energy and its temperature is increased. On the other hand, if the gas pushes the piston out, so that the gas is expanded, some work is done by the gas. It loses some of its energy and the gas cools down. Thus, we see that the internal energy of a system can be changed in two different ways: 1) by heating it or 2) by doing work on it. The experiment we discussed just now can be carried out in a very meticulous way so that we achieve the same change in temperature of the gas by both the methods. First Law of Thermodynamics: (Work and Heat are related) Figure shows an arrangement of thermodynamic system which consists of some quantity of an ideal gas enclosed in a cylinder with a movable, massless, and frictionless piston. In this, the gas inside the cylinder is the system and the cylinder along with the piston is its environment. First, consider the work done by the system (the gas) in increasing the volume of the cylinder. During expansion, the gas molecules which strike the piston lose their momentum to it, and exert a pressure on it. As a result, the piston moves through a finite distance. The gas does a positive work on the piston. When the piston is pushed in so that the volume of the gas decreases, the gas molecules striking it gain momentum from the piston. The gas does a negative work on the piston. o Consider Figure which shows a system enclosed in a cylinder with a movable, massless, and frictionless piston so that its volume can change. o Let the cross sectional area of the cylinder be A, and the constant pressure exerted by the system on the piston be P. The quantities W and Q can be positive, negative or zero, therefore, ∆U can be positive, negative, or zero 4. A gas contained in a cylinder fitted with a frictionless piston expands against a constant external pressure of 1 atm from a volume of 5 litres to a volume of 10 litres. In doing so it absorbs 400 J of thermal energy from its surroundings. Determine the change in internal energy of system 5. A system releases 125 kJ of heat while 104 kJ of work is done on the system. Calculate the change in internal energy. Thermodynamic state variables Any measurable or observable characteristic or property of a system when the system remains in equilibrium. Examples: Pressure, temperature, Volume, density etc. Types of Thermodynamic state variables Intensive and Extensive variables Intensive variables do not depend on the size of the system. Extensive variables depend on the size of the system Thermodynamic Equilibrium A system is in thermodynamic equilibrium if the following three conditions of equilibrium are satisfied simultaneously. These are, 1) Mechanical equilibrium, 2) Chemical equilibrium, and 3) Thermal equilibrium. Mechanical equilibrium When there are no unbalanced forces within the system and between the system and its surrounding, the system is said to be in mechanical equilibrium. The system is also said to be in mechanical equilibrium when the pressure throughout the system and between the system and its surrounding is the same. Whenever some unbalanced forces exist within the system, they will get neutralized with time to attain the condition of equilibrium. A system is in mechanical equilibrium when the pressure in it is the sane throughout and does not change with time. Chemical equilibrium A system is said to be in chemical equilibrium when there are no chemical reactions going on within the system, or there is no transfer of matter from one part of the system to the other due to diffusion. A system is in chemical equilibrium when its chemical composition is the sane throughout and does not change with time. Thermal equilibrium When the temperature of a system is uniform throughout and does not change with time, the system is said to be in thermal equilibrium. Thermodynamic State Variables and Equation of State Thermodynamic state variables describe the equilibrium states of a system The mathematical relation between the state variables is called the equation of state. For example, for an ideal gas, the equation of state is the ideal gas equation, PV = nRT Where, p, V and T are the pressure, the volume and the temperature of the gas, n is the number of moles of the gas and R is the gas constant. The graphical representation of equation of state of a system (of a gas) is called the P - V diagram, or the P - V curve (the pressure – volume curve), or the indicator diagram of the system. Figure below shows a typical P-V diagram for an ideal gas at some constant temperature. 𝑽𝟐 𝑽𝟐 The P - V diagram 𝑾= 𝒅𝑾 = 𝒑𝒅𝑽 𝑽𝟏 𝑽𝟏 The area under the curve in the p-V diagram, is the graphical representation of the value of the integral in the above equation. Since this integral represents the work done in changing the volume of the gas, the area under the p-V curve also represents the work done in this process. Consider a gas confined to a cylinder with a movable, frictionless, and massless piston can be, 1) expanded with varying pressure or 2) it can be compressed with varying pressure or 3) it can expand at constant pressure Figure shows expansion of the gas. Its volume changes due to outward displacement of the piston and the pressure of the gas decreases. The work done by the gas in this case is positive because the volume of the gas has increased. Figure shows compression due to inward displacement of the piston. The pressure of the gas is increased and the work done by the gas is now negative. Figure shows the P-V diagram when the volume of the gas changes from V1 to V2 at a constant pressure. The curve is actually a line parallel to the volume axis. The work done during volume change at constant pressure. 10. A hypothetical thermodynamic cycle is shown in the figure. Calculate the work done in 25 cycles. 12. A system is taken to its final state from initial state in hypothetical paths as shown figure calculate the work done in each case. Thermodynamic Process A procedure by which the initial state of a system changes to its final state is called thermodynamic process. During such a change, there may be a transfer of heat into the system from its environment, (positive heat), for example when water boils heat is transferred to water. Heat may be released from the system to its environment (negative heat). Similarly, some work can be done by the system (positive work), or some work can be done on the system (negative work). When the piston in a cylinder is pushed in, some work is done on the system. These changes should occur infinitesimally slowly so that the system is always in thermodynamic equilibrium. Such processes in which changes in the state variables of a system occur infinitesimally slowly are called quasi static systems. When a thermodynamic system changes from its initial state to its final state, it passes through a series of intermediate states. This series of intermediate states when plotted on a p - V diagram is called a path. Work Done During a Thermodynamic Process: Conclusion the work done by a system depends not only on the initial and the final states, but also on the intermediate states, i.e.,on the paths along which the change takes place. Heat Added During a Thermodynamic Process: Thermodynamic state of a system can be changed by adding heat also. Consider a thermodynamic system consisting of an ideal gas confined to a cylinder with a movable, frictionless, and massless piston. Suppose we want to change the initial volume V1 of the gas to the final volume V2 at a constant temperature. There are two different ways in which this change in volume can be made. Figure shows the first method. In this case, the gas is heated slowly, in a controlled manner so that it expands at a constant temperature. It reaches the final volume V2 isothermally. The system absorbs a finite amount of heat during this process. In the second case, shown in Figure the gas cylinder is now surrounded by an insulating material and it is divided into two compartments by a thin, breakable partition. The partition is now suddenly broken. This causes a sudden, uncontrolled expansion of the volume of the gas. The gas occupies the volume that was empty before the partition is broken. There is no exchange of heat between the gas and its environment because the cylinder is now surrounded by an insulating material. The final volume of the system after the partition is broken is V2. In this case, the gas has not done any work during its expansion because it has not pushed any piston or any other surface for its expansion. Such expansion is called free expansion. A common example of free expansion is abrupt puncturing of an inflated balloon or a tyre. It is experimentally observed that when an ideal gas undergoes a free expansion, there is no change of temperature. Therefore, the final state of the gas in this case also, is the same as the first case. The intermediate states or the paths during the change of state in the first and the second case are different. But the initial and the final states are the same in both cases. Figures (a) and (b) represent two different ways of taking a system from the initial state to the final state. This means we have two different paths connecting the same initial and the final states of a system. In case of the method shown in Figure (a) there is an exchange of heat. In case of the method shown in Figure (b), there is no exchange of heat and also, the system does not do any work at all because there is no displacement of any piston or any other surface. To conclude, heat transferred to a system also depends on the path. Classification of Thermodynamic Processes: 1. Reversible and Irreversible Processes: A reversible process is a change that can be retraced in reverse (opposite) direction. The path of a reversible thermodynamic process is the same in the forward and the reverse direction. Figure shows the path of a reversible thermodynamic process. This path shows a reversible expansion of a gas followed by its reversible compression. Such changes are very slow and there is no loss of any energy in the process and the system is back to its initial state after it is taken along the reverse path. Reversible processes are ideal processes. A real thermodynamic process will always encounter some loss due to friction or some other dissipative forces. An irreversible process is a change that cannot be retraced in reverse (opposite) direction. The path of an irreversible thermodynamic process is not the same in the forward and the reverse direction. Figure shows the path of an irreversible thermodynamic process. There is a permanent loss of energy from the system due to friction or other dissipative forces in an irreversible process. The change of state depends on the path taken to change the state during an irreversible process. An irreversible process shows a hysteresis. Most real life thermodynamic processes that we deal with are irreversible. 2. Isothermal process: A process in which change in pressure and volume takes place at a constant temperature is called an isothermal process or isothermal change. For such a system ∆T = 0. Isothermal process is a constant temperature process. This is possible when a system is in good thermal contact with its environment, and the transfer of heat from, or to the system, is extremely slow so that thermal equilibrium is maintained throughout the change. For example, melting of ice, which takes place at constant temperature, is an isothermal process. 3. Isobaric process It is a constant pressure process. Boiling water at constant pressure, normally at atmospheric pressure, is an isobaric process. Figure shows the p-V diagram of an isobaric process. It is called as an isobar. The different curves shown on the maps provided by the meteorology department are isobars. They indicate the locations having same pressure in a region. For an isobaric process, none of the quantities ∆U, Q and W is zero.