Theoretical Genetic Revision PDF 24-25
Document Details
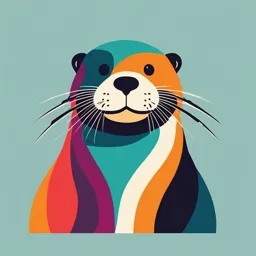
Uploaded by FantasticUniverse8061
MTI University
Tags
Related
Summary
This document provides a theoretical genetic revision for a medical biochemistry department, covering topics such as nucleic acid structure, DNA structure, and related concepts.
Full Transcript
Theoritical Genetic Revision Medical Biochemistry Department 24-25 Nucleic Acid Structure Basic Structure of Nucleotide Acid 5’...
Theoritical Genetic Revision Medical Biochemistry Department 24-25 Nucleic Acid Structure Basic Structure of Nucleotide Acid 5’ Base 1’ 4’ Adenine Monophosphate Guanine Purine Diphosphate Sugar Thymine Triphosphate Cytosine Pyrimidine 3’ 2’ Uracil Ribose, Deoxyribose Nucleoside (Adenosine) Nucleotide (Adenosine monophosphate, AMP) Nitrogenous Bases Nitrogenous Bases Pyrimidines Purines Cytosine Uracil Thymine Adenine guanine Nitrogenous Pentose Nucleoside base Nitrogenous base Pentose Phosphate Nucleotide P P P Adenine pentose Nucleoside (adenosine) Nucleoside monophosphate (AMP= Adenylic a) Nucleoside Diphosphate (ADP) Nucleoside triphosphate (ATP) Nucleoside Nucleotide Base +deoxyribose Purines Adenine Adenosine Adenylic (AMP) Guanine Guanosine Guanylic (GMP) Pyrimidine Thymine Thymidine Thymidylic (TMP) Cytosine Building units of nucleic acids : DNA, RNA Energy Transfer: ATP act as energy Importance carriers within cells. of nucleosides Constituents of co-enzymes e.g. and FAD, NAD nucleotides Signal Transduction: cAMP serve as second messengers. A methyl donor e.g. S-Adenosyl methionine (SAM) nucleoside DNA structure Primary structure (polydeoxyribonucleotides) Secondary structure (DNA double helix) Tertiary structure (chromosomes) Primary structure (Polydeoxyribonucleotides) Pentose sugar The order of the 2-deoxyribose. nucleotide bases determines the primary Nitrogenous structure or sequence bases of the DNA. A, G, C and T. Nucleotides d-AMP, d-GMP, d-CMP and d- TMP. Primary structure (Polydeoxyribonucleotides) DNA A=T A G=C In each DNA strand, the G alternating sugar and phosphate units form the C backbone (S-P-S-P-S-P). G A Nitrogenous bases which are C linked to the pentose sugar, are projecting to the inside of T the 2 strand at right angle. G Primary structure (Polydeoxyribonucleotides) – Each DNA strand has 2 terminal ends. 1. 3` terminus at which there is a free OH of pentose sugar. 2. 5` terminus at which there is a free phosphate group. P OH Sequence of nucleotide in DNA strand is written from 5`→ 3` direction. e.g. 5‘-GAC-3' Secondary structure (DNA double helix) Features of the double helix: Double helix: The two strands are antiparallel: Base pairing rule: Dimensions: Base pairing Rule and complementary bases A = T & C = G (Chargraff’s Rule) ❑ The 2 strands are complementary. ❑ The sequence of bases in one strand determines the sequence of bases in the other strand. Question: If there is 30% Adenine, how much Cytosine is present? There would be 20% Cytosine Adenine (30%) = Thymine (30%) Guanine (20%) = Cytosine (20%) Therefore, 60% A-T and 40% C- G 16 Nucleic Acid Chemistry DNA Denaturation Renaturation (Melting) DNA Denaturation Separation of 2 DNA strands by disruption of hydrogen bonds between 2 DNA strands by: Change in PH Heating Denatured DNA ATGAGCTGTACGATCGTG ATGAGCTGTACGATCGTG ATGAGCTGTACGATCGTG TACTCGACATGCTAGCAC TACTCGACATGCTAGCAC Double stranded DNA Double stranded DNA TACTCGACATGCTAGCAC Single stranded DNA Changes in pH Extreme pH levels (both high and low) ionization of bases disruption of H-bonds. DNA denaturation Heating (thermal denaturation) - Increasing the temperature can cause the hydrogen bonds to break. - This process is known as thermal denaturation Regions of the duplex that have predominantly A-T base-pairs will be less stable than those rich in G-C base-pairs. Melting point (Tm) Point is reached at which 50% of DNA molecule exist as single strands G-C content of sample (high GC contents, high Tm ) Tertiary structure (chromosomes) Eukaryotic cells must fit approximately up to 2 m of unpacked DNA into the spherical nucleus, which is a less than 10 micron diameter space. The tertiary structure of DNA refers to its three-dimensional arrangement within cell as chromatin. DNA-binding proteins non- Histones histones Histones Main packaging proteins 5 classes: H1, H2A, H2B, H3, H4. Rich in Lysine and Arginine 24 24 From DNA (2ry structure) to Chromosomes (3ry structure) Chromosome Chromatin fibers nucleosomes DNA double helix Nucleosome structure DNA HISTONE NUCLEOSOME ❑ The binding of DNA by histones generates a structure called the nucleosome. Nucleosome structure The nucleosome core contains an octamer protein structure consisting of 2 subunits each of H2A, H2B, H3 and H4 and 2 DNA turns. Histone H1 occupies the internucleosomal DNA and is identified as the linker histone. DNA replication DNA Replication DNA replication is the biological process by which a cell makes an identical copy of its DNA. It is essential process for the transmission of genetic information from one generation to the next in living organisms. DNA Replication When DNA replicates, each new double helix consists of one strand from the DNA replication is semiconservative original DNA molecule (the parental strand) and one newly synthesized complementary strand. DNA replication is bidirectional At the origin of replication two replication forks are formed which move in opposite direction to each other i.e. they move in both directions away from the origin of replication. DNA replication steps I- Separation of the 2 DNA strands and formation of the replication fork II- Synthesis of DNA strands In In Prokaryotes Eukaryotes I-Separation of the 2 DNA strands Enzymes and proteins responsible for DNA strands separation Replication steps: Separation of the 2 DNA strands Dna A protein It recognizes the ORI C produces local opening and unwinding of the DNA double helix. This process requires ATP Replication steps: Separation of the 2 DNA strands DNA helicase (DnaB protein) Unwinds DNA It separates the 2 DNA strands by cleavage of the hydrogen bonds between the two DNA strands It requires replication energy fork is formed provided by ATP Replication steps: Separation of the 2 DNA strands DNA binding proteins (SSB) binds to the single-stranded DNA prevents the renaturation of the double helix & stabilizes the separated strands. protects the DNA from the nuclease enzyme that cleave single stranded DNA DNA supercoiling DNA Topoisomerases Topoisomerases http://thepointeedition.lww.com/FullTextService/CT%7b06b9ee1beed59419cc706ceee9ddda6cc77b987fd7479682287ccc275ac7ca3bb38b2b3d0236d7708568555d93246333%7d/DA6C29FF11.gif?geom=200x200 are enzymes that are responsible for the elimination of supercoils. Solve the problem of DNA supercoiling. Toposiomerase has both Nuclease (strand cutting) Ligase (strand resealing) Toposiomerase Function +ve Supercoil Nicking Clinical significance Quinolones are a class of broad-spectrum antibiotics used to treat a variety of bacterial infections inhibiting bacterial DNA gyrase (topoisomerase II) which are crucial for bacterial DNA replication preventing bacterial DNA replication, leading to bacterial cell death. Clinical significance Bloom syndrome rare autosomal recessive disorder Caused by mutations in the gene, which encodes a DNA helicase. - Characterized by: short stature, sun-sensitive skin changes, and a high risk of various cancers. DNA replication steps II- Synthesis of DNA strands (in Prokaryotes( DNA replication steps (II- Synthesis of DNA strands) 1-Initiation of DNA Synthesis: (Formation of RNA primer) 2- Elongation of DNA strand 3-Excision of RNA primer and filling of gaps by DNA Players of New DNA strand synthesis RNA Primer Synthesis of newSynthesis DNA strands In Primase Prokaryote s To initiate DNA synthesis RNA primer (10 bases) is formed by the action of primase (DNA dependent RNA polymerase). Main player of DNA chain elongation In Prokaryote s DNA polymerase III 2- Elongation of DNA strand Synthesis of leading Synthesis of lagging - Leading strand strand is - strand Lagging strand is synthesized synthesized continuously in discontinuously in short fragments called Okazaki fragments. the direction of the - It is synthesized in opposite the replication fork direction. direction of replication fork. - DNA polymerase III - DNA polymerase III synthesizes synthesizes the leading each Okazaki fragment, starting strands. from an RNA primer synthesized by primase. 3-Excision of RNA primer and filling of gaps by DNA DNA polymerase I enzyme removes the RNA primers using its 5’to 3' exonuclease activity. As it removes the RNA, DNA pol I replaces it with deoxyribonucleotides, synthesizing DNA in the 5'→3' direction (5'→3' polymerase activity). DNA ligase functions to seal breaks in the phosphodiester backbone of DNA strands. The enzyme requires energy. Proofreading of the newly synthesized DNA -As each nucleotide is added to the chain, DNA pol III checks to make certain the added nucleotide is correctly matched to its complementary base on the template. -If it is not, the 3'→5' exonuclease activity removes the error. The 5'→3' polymerase activity then replaces it with the correct nucleotide. DNA Pol I has three activities 1- Exonuclease for excision of RNA primers and repair. activity from 5’→3’ 2- Polymerase for the synthesis of DNA segments activity from 5’→3’ replacing RNA primers. 3- Exonuclease for proof reading activity during activity from 3’→5’ the synthesis of DNA segments. Eukaryotic DNA polymerases Proofreading Function Polymerase activity pol α 1-Primase activity (subunit): RNA primer synthesis No Multisubunit 2-Polymerase activity(another subunit): synthesis enzyme of a short DNA piece extending the primers pol β Repair No Pol γ Replicates mitochondrial DNA Yes pol Elongates okazaki fragments Yes (lagging strand) Pol ε Elongates leading strand Yes Repair Comparison between prokaryotic and eukaryotic DNA replication. Point of Prokaryotic replication Eukaryotic replication difference Location Cytoplasm Nucleus Origin of replication Single Multiple Enzyme responsible for pol α Primase Primer synthesis Processing enzyme DNA Polymerase III DNA pol & Pol ε Enzyme responsible for DNA polymerase I RNase H Removal of primer DNA Repair Causes of DNA Damage Endogenous Factors: - Replication Errors: Mistakes that Exogenous Factors: occur during DNA replication. - UV Radiation: Causes thymine dimers. - Metabolism: Reactive oxygen - Chemicals: Environmental toxins like species generated during normal cellular metabolism. tobacco smoke or industrial chemicals. - Spontaneous Hydrolysis: - Ionizing Radiation: X-rays and gamma Hydrolysis reactions that can alter rays can break DNA strands. DNA bases. General steps of repair: Recognition of the damage (lesion) on the DNA, Removal (excision) of the damage, Replacement (filling the gap) using the sister strand as a template for DNA synthesis Ligation. Mechanisms of DNA Repair Type of DNA damage DNA Repair Diseases due to defect in repair Replication errors Mismatch repair Heredietary non polyposisis cancer coli (escape proofreading) Exposure to UV DNA Direct repair (photoreactivation): Xeroderma pigmentosa strand producing a In prokaryotes only with skin hypersensitivity &cancer dimer (T=T). Nucleotide excision repair: - In prokaytoes and eukaryotes. - It repairs damage affecting longer strands of 2–30 bases. Chemicals e.g Base excision repair: nitrous oxide, resulting in deaminating Cytosine into Uracil (U). Thymine Dimer & Nucleotide Excision Repair Bulky lesions caused by UV radiation e.g. a pyrimidine dimer, thymine,thymine dimer (T=T). TRANSCRIPTION I 58 TRANSCRIPTION TRANSCRIPTION = DNA → RNA The synthesis of RNA molecule using DNA molecule as a template by DNA dependent RNA polymerase. Upstream: on the 5´side of any given position on the coding strand. Downstream: on the 3´side of any given position on the coding strand. Transcription in PROKARYOTES RNA polymerase in prokaryotes RNA Polymerase: involves a core enzyme and several auxiliary proteins. 1-Core enzyme 2-Holoenzyme: 2 (α), 1 (β), and 1(β'), - Consists of σ subunit Core are responsible for the “sigma factor” & core enzyme enzyme assembly. enzyme. cannot recognize the The σ subunit “sigma Holoenzyme promoter region (lacks factor” enables RNA pol to specificity). recognize promoter regions sigma on the DNA and increases factor the affinity of holoenzyme to the promoter region. Types of prokaryotic promotors Pribnow or –35 TATA box: sequence: This is formed of A consensus six nucleotides sequence (5′- (5′-TATAAT-3′( TTGACA-3′), is The Pribnow box centered about 35 is centered at bases to the left of approximately the transcription base -10. start site. Steps of Transcription I-Template binding & formation of preinitiation complex II-Initiation III- Elongation IV-Termination I- Template Binding & Formation of Pre-initiation Complex (PIC): The process of transcription begins with the binding of the RNA polymerase holoenzyme to the promoter to form a preinitiation complex (PIC). Elongation in prokaryotes: After about 10 nucleotides are formed The core enzyme moves along the template The sigma subunit is released, strand Elongating the RNA transcript TERMINATION The elongation of the single- stranded RNA chain continues until a termination signal is reached. Termination can be intrinsic (spontaneous) or dependent upon the participation of a protein known as the r (rho) factor. Rho-dependent termination (ATP dependent) A protein factor (termination factor( called ρ factor "Rho". This factor binds to a C-rich region near the 3'-end of the newly synthesized RNA. Rho factor has ATP-dependent RNA-DNA helicase activity that hydrolyzes ATP, Rho factor uses the energy to release the 3'-end of the transcript from the template. Rho-independent Termination (Intrinsic Termination) Terminators or termination signals Consist of Specific inverted regions of repeat DNA cause sequences termination rich in GC of followed by transcription. AT rich region. Transcription of this region by RNA polymerase generates a hairpin (loop) structure Rho-independent Termination (Intrinsic Termination) Terminators or termination signals Inverted repeats of followed by AT rich region Followed by Transcription of this uracil rich region by RNA sequence at the polymerase generates a 3' end of hairpin (loop) structure nascent RNA. -The hairpin structure causes RNA polymerase to pause and the presence of UUUU sequence facilitates unwinding of nascent RNA from DNA because of weak attraction between AU pair. -This leads to termination of transcription. Clinical Correlation: Some antibiotics prevent bacterial cell growth by inhibiting RNA synthesis: Rifampicin is important in the treatment of tuberculosis. inhibits transcription by binding to the b preventing chain Rifampicin subunit of extension beyond prokaryotic RNA three nucleotides. pol Eukaryotic RNA Polymerases Eukaryotic RNA Polymerases RNA polymerase I Synthesis of rRNA RNA polymerase II Synthesis of mRNA & snRNA RNA polymerase III Synthesis of tRNA, 5SrRNA, snRNA Clinical Application Clinical Significance α amantin A potent toxin produced by the poisonous mushroom Amanita phalloides (sometimes called “the death cap”) Inhibits RNA pol II by forming a tight complex with the enzyme It inhibits mRNA synthesis. 72 Regulation of gene expression in Eukaryotes I- Pre-transcriptional Regulation of gene 1- Chromatin remodeling expression 2- DNA methylation: (Epigenetic mechanisms) II-Transcriptional A-Cis-acting DNA sequences. Regulation of gene B-Trans-acting protein molecules expression (Transcription Factors, TFs) III-Post-transcriptional mRNA Regulation of gene rRNA expression tRNA I- Pre-transcriptional Regulation of gene expression (Epigenetic mechanisms) Changes occurs to histone proteins& DNA: 1-Chromatin remolding 2-DNA (Histone methylation acetylation/deacetylation) 74 Feature Active Chromatin Inactive Chromatin Chromatin Open, relaxed Condensed, Structure (euchromatin) tightly packed (heterochromatin) DNA Accessibility High Low Transcriptional High Low or none Activity (transcriptionally (transcriptionally active) silent) Histone acetylation Acetylation of lysine residues at the amino terminus of histone proteins by histone acetyl transferase (HAT) This eliminates lysine positive charges and thereby decreases the interaction of the histone with the negatively charged DNA. Following acetylation and nucleosomes removal: The promoter is opened Transcription is active. 76 2-DNA methylation Methylation is an epigenetic process in which methyl groups are added to the DNA molecule. addition of methyl groups at the C5 position of cytosine by DNA methyltransferase (SAM acts as methyl donor). reversed by DNA demethylase 77 DNA methylation Methylation is related to the Heterochromatin formation transcriptionally (tightly packaged form of DNA) silent genes Transcriptionally active chromatin is predominantly high levels unmethylat of ed/hypom acetylated ethylated histone. 78 Differences between cis and Trans acting elements Cis-acting elements Trans-acting elements Definition Influence the expression of the Diffuse through the cytoplasm, acts adjacent genes on the same at target DNA sites on any DNA chromosomes molecules inside the cells examples Promotor General (basal)transcription factors Binds to promoter Enhancer Activators and co-activators protein Bind to enhancer Silencer Repressor protein 79 Bind to silencer Cis –acting elements Trans- acting elements 80 Eukaryotic Promoters 2. CAAT box and GC box 1. TATA box: regions: It defines where transcription is to commence along the DNA. Present upstream (-40-200 bp) of the transcription start site This region has the sequence of TATAAA. Contribute to the mechanisms that control how frequently The TATA box is usually located 20-30 bp this event is to occur. upstream from the transcription start site. Post-Transcriptional Modification 82 In Eukaryotes messenger RNA Post-transcriptional modifications 1-Capping at 5’end 2- Splicing (Removal of introns & splicing of exons) 3-Addition of poly (A) tail at 3’ end. Importance of capping a- It protects the 5`-end from ribonucleases. b- It facilitates initiation of protein synthesis. 2. Addition of a poly-A tail at the 3`end Most eukaryotic mRNA have a chain of 40–250 adenine nucleotides attached to the 3’-end. Poly (A) polymerase (PAP) is responsible for the addition of poly(A) tail at the 3`end of pre- mRNA. Importance of poly A tail: It protects mRNA against the attack by ribonucleases. It binds poly A tail-binding proteins which increases the efficiency of translation. Splicing Primary mRNA snRNA Exons Introns Exons Introns Exons Introns Exons Introns Spliceosome snRNP: snurps: spliceosomes Special (snRNA + protein + primary transcript). Proteins A specific base sequence at the exon-intron junction determines the site of splicing. Exons Exons Exons Exons 3. Eukaryotic tRNAs processing 1- Removal of the extra Small scissors 2- Replacement of 5`sequence (leader UU nucleotides with sequence).Small scissors the CCA sequence at the 3`end. 4- Modification of some bases e.g. methylation 3-Removal of short intron, which is present in the anticodon loop. Features of the Genetic Code Specificity Degeneracy Universality Unidirectional Continuous Non overlapping Types of Mutations I-Base Substitution (Point Mutations) A point mutation is a genetic alteration where a single nucleotide base in the DNA sequence is changed(the most common type ) Transition Transversion in which one purine is replaced in which a purine is replaced by by another purine or one pyrimidine is replaced by a pyrimidine, or a pyrimidine is another pyrimidine. replaced by a purine. Missense Consequences of point mutation mutation (Changing a single Nonsense nucleotide can lead mutation to any one of three results:) Silent mutation A- Missense mutation amino acid Missense a changed change in the mutation codon protein product of that gene. B- Nonsense mutation Translation of the protein conversion of the mRNA product is an amino acid Nonsense from that usually non- codon to a mutation gene is functional stop or non- prematurely e.g. sense codon. terminated thalassemias c- Silent mutation no change occurs in the formation of a codes for the Silent amino acid synonym same amino mutation sequence of codon acid the gene products. Translation Dr. Walaa Ibrahim Components Required for Translation Amino acids Aminoacyl-tRNA synthetases Three classes of RNA 1. tRNAs 2. mRNA (template for the synthesis of protein). 3. ribosomes. Different Protein Source of Factors F. energy (Initiation, (ATP and GTP) elongation, and Aminoacyl-tRNA synthetases: This family of enzymes is required for attachment of amino acids to their corresponding tRNA. Each member of this family recognizes a specific amino acid and the tRNA that correspond to that amino acid. Steps in protein synthesis ❑The mRNA is translated from its 5′-end to its 3′-end, producing a protein synthesized from its amino-terminal end to its carboxyl terminal end. 1 INITIATION 2 ELONGATION 3 TERMINATION Steps of Protein Synthesis. 3 Phases Initiation Elongation Termination 1-Initiation Initiation of protein synthesis involves the assembly of the components of the translation system to form the initiation complex. These components include:: Two ribosomal subunits mRNA to be translated aminoacyl-tRNA specified by the first codon in the message GTP (which provides energy for the process) Initiation factors that facilitate the assembly of this initiation complex. Mechanisms by which the ribosome recognizes the nucleotide sequence that initiates translation: A-In Prokaryotes Shine-Dalgarno Sequence : the ribosome recognizes the Shine-Dalgarno sequence, a purine-rich region located upstream of the start codon (AUG) on the mRNA. This sequence aligns with a complementary sequence on the 16S rRNA of the small ribosomal subunit, facilitating the correct positioning of the ribosome for translation initiation B-In Eukaryotes - The 40S ribosomal subunit binds to the cap structure at the 5′-end of the mRNA and moves down the mRNA until it encounters the initiator AUG. -This “scanning” process requires ATP. Shine-Dalgarno sequence Elongation The Three Steps of Elongation 1] Binding of an Incoming Aminoacyl-tRNA 2]Peptide Bond Formation By Peptidyl transferase in the 50S ribosomal subunit. 3] Translocation (the ribosome advances three nucleotides toward the 3′-end of the mRNA. During translation: The A binds an incoming aminoacyl-tRNA site The occupied by peptidyl-tRNA. This tRNA carries the chain P-site of amino acids The E occupied by the empty tRNA as it is about to exit the site ribosome. - Stop Codon Recognition: The ribosome continues to translate the mRNA until it encounters a stop codon (UAA, UAG, or UGA). - Release Factor Binding: A release factor protein binds to the 3-Termination stop codon in the A site, prompting the ribosome to release the newly synthesized polypeptide chain. - Ribosome Disassembly: The ribosomal subunits, mRNA, and tRNA dissociate, ready to be used again for another round of translation. Trimming Post-translational Covalent modifications alterations Protein degradation POSTTRANSLATION MODIFICATION 1. Trimming Many proteins are initially made as large, precursor molecules that are not functionally active. Trimming refers to the process of removing specific peptide sequences from a polypeptide chain. Specific sequence is removed by specialized endoproteases, resulting in the release of an active molecule. POSTTRANSLATION MODIFICATION 2. Covalent alterations - Proteins may be activated or inactivated by the covalent attachment of a variety of chemical groups. - Examples of these modifications include:. Phosphorylation Glycosylation Hydroxylation. POSTTRANSLATION MODIFICATION 3. Protein degradation - Proteins that are defective or destined for rapid turnover are often marked for destruction by ubiquitination, the attachment of a small protein, called ubiquitin. Proteins marked in this way are rapidly degraded by a cellular component known as the “proteasome,” which is a complex, ATP-dependent, proteolytic system located in the cytosol. Post-translational modifications Post-translational modifications 2-Covalent 3-Protein 1-Trimming Modification degradation Some large, Digestive precursor By specialized Proinsulin enzymes Phosphorylation Destruction by is trimmed ubiquitination molecules endoproteases to produce Glycosylation not , resulting in insulin. (attachment of functionally the release of Hydroxylation ubiquitin). active. an active molecule. Ub is a small protein (76 AA). Degraded by “proteasome,” using ATP.