Theme 1 Module 3 Script PDF 2021
Document Details
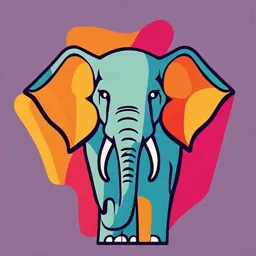
Uploaded by AppropriatePigeon3507
McMaster University
Tags
Summary
This document contains lecture notes on proteins, covering their roles, composition, and the endomembrane system. It includes slides and diagrams describing the synthesis and structure of proteins, as well as different types of protein structures.
Full Transcript
Theme 1 Module 3 Script 1 Slide 1: Theme 1, Module 3: Proteins YOUR SUMMARY NOTES: Slide 2: The learning objectives for you in this module are to: Identify the various roles of proteins in th...
Theme 1 Module 3 Script 1 Slide 1: Theme 1, Module 3: Proteins YOUR SUMMARY NOTES: Slide 2: The learning objectives for you in this module are to: Identify the various roles of proteins in the cell. Describe the composition of a polypeptide and the four levels of protein structure. Outline the role of the endomembrane systems in protein synthesis and protein transport. Use examples of specific proteins to represent general mechanisms of protein synthesis, structure and distribution within the cell. Slide 3: Let's start with proteins, what do they do, how are they made, what do they look like? Slide 4: As we have been looking at the outer membrane and organelles of the cell, we have seen many different types of proteins. In Module 1, we saw transport proteins embedded in the cell membrane that move substances across the cell membrane. In Module 2, we learned proteins can act as enzymes to speed up the synthesis of carbohydrates. We also saw enzymes in the mitochondria that allow for the release of stored energy from carbohydrates. Proteins also serve to make up a large part of the structures that are important for moving an individual cell or for moving large cargo or molecules within cells. This is the case when we see the interaction between actin and myosin that slide past each other in contractile bundles. Certain proteins embedded in the cell membrane interact with carbohydrates and are also important for cell to cell signalling. Cytoplasmically situated proteins allow for further amplification of these cell to cell signals, often leading to the appropriate cellular responses. Other important proteins are those involved in defense mechanisms in our bodies. These proteins are made by specialized immune cells and serve the role of antibodies that can destroy disease-causing viruses and bacteria. We know of the different types of proteins, but how were these proteins made? What do they look like? Where do we see proteins in the cell? To answer these questions, we need to look further into the importance of the endomembrane system of the cell. Theme 1 Module 3 Script 2 Slide 5: The various proteins in our bodies can have YOUR SUMMARY NOTES: different sizes and shapes. Many of these structural differences relate to their functions. For example, the TATA-box binding protein, which interacts with DNA molecules, is able to do so due to a groove in the protein that allows for this interaction. Porin proteins are important for transport of water for example across the cell membrane and have a structural hydrophilic pore that allows for this to occur. Most soluble cellular proteins may take more globular shapes with a hydrophobic interior and a hydrophilic exterior that allows the protein to be soluble in the aqueous environment of the cytosol, such as haemoglobin at the bottom. Slide 6: How do scientists represent protein structures? Here are two examples of ways of representing a subunit of the insulin protein. On the left is a space-filling model. This shows the actual relative size and location of each atom, representing atoms with different colours. On the right is a ribbon diagram. The lines represent the backbone of the protein polymer. The broader line represents an organized reiterated structure called an alpha helix while the thinner line represents a less ordered loop. Slide 7: Now lets look at how proteins are synthesized in the cell. Slide 8: The basic principles are the same for both prokaryotic and eukaryotic cells. The genetic information for every protein is encoded in the DNA of the cell. The first step is to transcribe that information into RNA which serves as the template for synthesis of a polypeptide. In the prokaryotic cell this is closely linked with translation of the RNA into proteins by ribosomes. In a eukaryotic cell the primary RNA transcript is processed to messenger or mRNA and transported into the cytoplasm where it is then translated into the protein by the ribosomes. Theme 1 Module 3 Script 3 Slide 9: YOUR SUMMARY NOTES: The nucleus contains most of the genes in eukaryotic cells. As seen in this high-resolution electron micrograph, the double-membraned nuclear envelope surrounds the nucleus and encloses the genetic material (chromosomes), separating the nuclear contents from the cytoplasm. The nucleus contains a region called the nucleolus. It is within the nucleolus that the ribosomal RNA molecules that are an important component of the ribosomes are transcribed. Before protein synthesis can occur, the appropriate segment of DNA is read and transcribed into RNA within the nucleus. Slide 10: The double membrane of the nuclear envelope has embedded nuclear pore complexes that allows for material to flow into and out of the nucleus. Most ribosomal RNAs are manufactured in the nucleolus where they bind to proteins to form the ribosomal subunits. These structures are then exported to the cytoplasm. Slide 11: The nuclear pore complexes allow the transcribed RNA to exit the nucleus for further processing in the extranuclear regions as seen in the animation. Messenger RNA which has been transcribed from DNA and carries the information to make proteins are also transported to the cytoplasm through these nuclear pores. However, nuclear pores are not only one way complexes. While they can transport RNA and ribosomes out of the nucleus, they can also import the important building blocks of DNA and RNA into the nucleus, along with enzymes that are important for the transcription of DNA into RNA. Theme 1 Module 3 Script 4 Slide 12: YOUR SUMMARY NOTES: As mentioned earlier, the cellular machinery that are involved in the synthesis of proteins are called ribosomes. The structural components of ribosomes are made in the nucleolus and are then shipped out of the nucleus. Once exported from the nucleus, these ribosomes assemble and have large and small subunit components that both contain RNA molecules and proteins. Ribosomes are able to remain soluble in the cytoplasm as free ribosomes or can attach to the endoplasmic reticulum to become bound ribosomes. These two different types of ribosomes can produce different proteins that will be destined for different parts of the cell or even secretion. Let us take a closer look at the sequence of events that occurs during protein synthesis. Slide 13: All cells produce a large number of distinct proteins. While the number of distinct proteins in a single cell depends upon the type of cell that you are looking at, estimates range from a few hundred to tens of thousands. What is incredible, is that all the possible proteins are derived from just 20 core amino acids. All of the 20 amino acids have a common structure: a central carbon atom bound to an amino group, a carboxyl group, a hydrogen atom and a variable side chain (represented by the R). Amino acid monomers are put together to make linear strands which we call polymers or polypeptides. A polypeptide is a strand of amino acids that are covalently bound to one another through a condensation reaction which releases water. This bond can also be broken through the process of hydrolysis which requires the addition of water. During protein synthesis, the unique sequence of amino acids in each protein is referred to as the primary structure of that protein. Theme 1 Module 3 Script 5 Slide 14: YOUR SUMMARY NOTES: The condensation reactions that join amino acids are catalyzed within the ribosome. Here we can see the process of translation. A polymer of amino acids can be seen at the top in green. The elongating chain is extruded out of the large subunit of the ribosome. The specific sequence of the amino acid polymer is determined by translating the information in the messenger RNA (or mRNA) seen at the bottom tracking through the cleft between the large and small subunits of the ribosome. This process of translation is achieved by a molecule called a transfer RNA (or tRNA). This molecule matches the sequence of nucleotides on the mRNA and carries the appropriate amino acid to the next position in the growing polypeptide chain. We will examine this process in more detail in our Theme: Transcription and Translation. Slide 15: During translation by a ribosome, amino acids polymerize to form the primary structure when a bond forms between the carboxyl group of one amino acid and the amino group of another. The C-N bond that results from this condensation reaction is called a peptide bond. When amino acids are linked together in this manner, the amino acids are referred to as residues and the formed polymer is referred to as a polypeptide. Each of the amino acids incorporated into a polypeptide has a distinct set of properties that are determined by their specific variable side chain (the R group). The amino acids side chains differ in size, shape, and chemical properties. Some side chains are hydrophobic, while others are hydrophilic. The hydrophobic side chains may aggregate in an aqueous environment as a result of thermodynamically stable hydrophobic interactions. The hydrophilic side chains may be charge-polarized and capable of forming ionic bonds, while other side chains favour the formation of hydrogen bonds. It is these interactions between the side chains that will allow the formation of a stable three-dimensional shape called a protein. Theme 1 Module 3 Script 6 Slide 16: YOUR SUMMARY NOTES: Once translation of mRNA to a polypeptide has occurred, the final folded and functional proteins can have many destinations depending on which ribosomes translated the mRNA to the proteins. If the proteins were synthesized by ribosomes that are located in the cytosol (or free ribosomes), then these proteins are destined to remain in the cytosol or be targeted to various cell organelles. Proteins that remain in the cytosol will include the enzymes involved in glycolysis, or serve as the proteins that make up the structural proteins of the cell (these include actin and tubulin). Other proteins that are synthesized by free ribosomes can be transported through nuclear pores into the nucleus to act as histone proteins or transcription factors, and can also head towards the mitochondria or chloroplasts and for example can serve the role of the many membrane proteins in these organelles. When proteins are targeted to organelles, it is a special sequence on the protein that targets a particular protein to an organelle. Slide 17: Checkpoint question #1 Slide 18: Once a polypeptide has been synthesized how does it obtain the shape that is required for its function? Theme 1 Module 3 Script 7 Slide 19: YOUR SUMMARY NOTES: In module 1, we saw that the amphipathic properties of the phospholipids were essential for the formation of the bilipid membrane. The hydrophilic head groups face the aqueous environment of the cytosol or the exterior of the cell and the hydrophobic core is hidden in the core of the membrane. In the same way, the side chains of the amino acid residues, whether they are hydrophilic or hydrophobic, determines the overall three-dimensional shape of the protein. At the beginning of this lecture, we highlighted the important cellular functions that can be played by proteins, all proteins have distinct three- dimensional shapes that are critical to their functions. The three-dimensional folded shape of that polypeptide is essential to allow for the proper function of the protein. All proteins, whether synthesized by free or bound ribosomes take on a three-dimensional folded shape. Here we see a globular protein found in the cytosol. The exterior of the protein is hydrophilic, allowing the protein to be soluble in the cytosol. The core is hydrophobic. Similar protein folding occurs by proteins that are formed by bound ribosomes. The difference is that these proteins continue through the ER into the aqueous environment of the lumen of the ER. It is in the ER that they become folded and functional. How are these folded protein structures achieved? Slide 20: A protein's primary structure will largely determine the manner in which it folds. At the top of this slide, we see a polypeptide strand where each amino acid monomer is represented by a rectangle. When we look at the three- dimensional shape of proteins, we can simplify the figure with a ribbon diagram where we only look at the backbone. At the bottom of the slide, we see a protein polymer that is represented by a convoluted and folded green line. There are three ways in which the backbone is distinguished here: a spiral, a broad line with an arrowhead, and a thin line. All are part of the same polymer and we will see what each of these represent. As we do so, we will see how we can get from a linear array to a three dimensional structure. Once an mRNA molecule is translated into a protein, that polypeptide strand begins a folding cascade determined by the properties of the amino acids making up that polypeptide. Theme 1 Module 3 Script 8 Slide 21: YOUR SUMMARY NOTES: Let's start with the simple folded structure illustrated as a spiral in the ribbon diagram - the secondary structure called an alpha helix. In this diagram the thick band is representing the backbone and the side chains are indicated. The amino and carboxyl groups are attached by covalent bonds called peptide bonds to form the polypeptide chain. In addition, the polymer is turned into a spiral or coil by the formation of noncovalent bonds called hydrogen bonds. Specifically, these hydrogen bonds form between the carbonyl of the carboxyl group of one amino acid residue and the amide of the amino group of another amino acid residue four positions away. As a result, all of those variable side chains (R- groups) stick out from the helix. This is where we see the importance of the differences between the 20 amino acids. The properties of the amino acids - specifically their R-groups - will determine the properties of the whole structure. Slide 22: In addition to forming alpha helices, secondary structures can also include beta pleated sheets, which are illustrated as broad lines with arrow heads in the ribbon diagram. Beta-pleated sheets are made up of parallel protein strands with hydrogen bonds also formed between the carboxyl and amino groups of adjacent strands. Unlike the alpha helices, the beta pleated sheets have hydrogen bonds that allow for a pleated like organization of this secondary structure. Slide 23: It's time to see this for yourself! Before you move, print out the Theme1-Module3 PDF file. This will be your starting primary polypeptide sequence that you will be able to cut out and fold to provide you with a better visualization into how a polypeptide strand can form its secondary structure. Theme 1 Module 3 Script 9 Slide 24: As we just observed, it is the interaction between YOUR SUMMARY NOTES: components of a protein's backbone that are responsible for the formation of alpha helices and beta sheets, the secondary structure of the protein. With regards to the overall shape or tertiary structure of a protein, this is all due to the interactions between the variable side chains or R-groups that allows the protein backbone to bend and fold, and therefore leading to the 3D shape of the protein. There are various interactions that can occur between these R- groups. These can include hydrogen bonds, Van der Waals interactions, covalent and ionic bonds, disulfide bonds, and hydrophobic interactions. Slide 25: The formation of a 3D protein from a linear amino acid polypeptide sequence can best be seen in this video. From the initial polypeptide amino acid sequence, all proteins are able to form interactions both within the protein backbone and between the variable side chain R groups. These interactions are essential to produce properly folded and functional proteins. Slide 26: While some protein folding occurs spontaneously as the polypeptide leaves the ribosome, there are also cellular mechanisms that assist protein folding. One mechanism is the use of molecular chaperones. These proteins bind to hydrophobic regions of the nascent polypeptide and prevent incorrect folding just long enough for the correct structure to form. Another mechanism is the use of chaperonins. These are large molecular complexes that form isolation chambers. Inside the chamber, a single nascent protein is sequestered away from other proteins so that it can fold without interference and the formation of incorrect bonds. Slide 27: Let’s review this process in the context of the protein hemoglobin. The primary structure is the sequence of amino acids synthesized from the mRNAs transcribed from the globin genes. Based on the properties of the amino acids, these polypeptide chains fold into secondary structures such as alpha helices and beta sheets. The tertiary structure forms because of interactions between the R groups of the amino acids. The final step is formation of the quaternary structure which is the association of different polypeptide subunits to form the fully functional protein. Theme 1 Module 3 Script 10 Slide 28: Checkpoint question #2 YOUR SUMMARY NOTES: Slide 29: We saw that proteins synthesized by free ribosomes remain in the cytosol, but what of proteins that need to be secreted from the cell? Slide 30: Today's eukaryotes were derived and modified from an ancestral prokaryote that contained genetic material of inheritance. With the formation of early eukaryotes, the invagination of the cell membrane formed the endomembrane system. This endomembrane system includes the nuclear envelope, endoplasmic reticulum, Golgi apparatus and lysosomes, and allows for compartmentalization of the cell for different functional roles. We can step through the components of the endomembrane system by examining protein synthesis. In addition to free ribosomes that are present in the cell, there are ribosomes that are bound to the membranes of the rough endoplasmic reticulum. These ribosomes produce proteins that are able to enter the lumen of the ER for further processing including protein folding. But how is this accomplished? How do the proteins then move through to the Golgi apparatus and reach their final destinations? We answer these questions as we continue through this module. Slide 31: mRNAs that encode proteins that are destined for the endomembrane system include a special signal sequence that once translated causes the ribosomes to become bound to the endoplasmic reticulum. This special signal sequence is synthesized by the ribosome at the very initial part of the protein. Once this signal sequence appears, it binds a signal recognition particle (SRP) which then binds to a signal recognition particle receptor (SRPR) in the ER membrane. Once this interaction occurs, the polypeptide can continue to be translated and is able to enter into the lumen (or the central canal) of the ER. Inside the lumen of the endoplasmic reticulum, the signal sequence is removed, and the translated polypeptide is now able to undergo important changes that allow for the final stages of protein processing and maturation to occur. These stages will be highlighted in the subsequent slides that follow. Theme 1 Module 3 Script 11 Slide 32: While some proteins remain in the ER many YOUR SUMMARY NOTES: proteins will continue to journey to their final destination within vesicles: small membrane- bound compartments in the cell that bud off from the ER. The ER is also the site where proteins get modified by the addition of one or more carbohydrate chains (a process called glycosylation). Glycosylation occurs on most secreted and membrane bound proteins and can help contribute to protein stability, folding and even cell-cell recognition. Once released from the ER the next destination is the Golgi apparatus. Some glycosylation reactions occur in the lumen of the ER, while some can occur in the Golgi apparatus. Proteins are transported to the Golgi apparatus in vesicles that pinch off from the ER. These vesicles are then able to fuse with the Golgi and deposit their contents in the lumen of the Golgi apparatus. Slide 33: In the Golgi apparatus, further protein modification occurs. Protein modifications can include the addition of carbohydrate groups. Again, this may be the home of the protein, but other proteins will enter into new vesicles and travel further through the endomembrane system of the cell. Some proteins will go to the cell membrane (such as porins, receptors), others will be secreted out of the cell (such as antibodies, hormones, enzymes), or perhaps to other organelles such as the peroxisome, the lysosome, or vacuoles. How is it that proteins are sorted to each of these destinations? Slide 34: There is evidence to suggest that each protein that comes out the Golgi apparatus has a TAG that allows for it to be packaged into a particular type of transport vesicle. These transport vesicles also have their own respective tags that allow for them to be transported to the appropriate destination- the cell membrane, lysosome, or even back to the endoplasmic reticulum. Once these vesicles reach their destination, the vesicle phospholipid bilayer will fuse with that of the cell membrane, lysosome or ER. This allows for the release of soluble proteins, or even for embedding of transmembrane proteins in the target membranes. Yet how are these vesicles able to reach their destination? Theme 1 Module 3 Script 12 Slide 35: There are structural and motor components of the YOUR SUMMARY NOTES: cell that play a very important role in being able to transport vesicles toward the cell membrane and in reverse towards the nucleus. The cytoskeleton in particular, is a dense network of fibers that helps maintain and also change cell shape. One of these cytoskeletal components are the microtubules. These structures are protein polymers that form long fibers which stretch through the cell. These structures function as cellular roadways that allow for vesicles to be transported along. Motor proteins such as kinesin and dynein have the ability to attach to a transport vesicle, and then walk along microtubules using the energy released from the hydrolysis of ATP. Slide 36: Many soluble proteins are synthesized and folded in the cytosol where they continue to function. Others are transported to various destinations in the cell. Proteins destined for the mitochondria, chloroplast, and peroxisomes (a membrane- bound organelle required for fatty acid metabolism) are synthesized on free ribosomes. In some cases the proteins fold before transport to the organelle, in other cases folding occurs after transport. In addition, some proteins are targeted to the lumen of the organelle (the aqueous interior) while other proteins are embedded in the membranes of the organelle. Slide 37: Checkpoint question #3 Slide 38: How does protein structure relate to function? Slide 39: Aquaporins are important proteins that are embedded in the plasma membrane and allow for the movement of water from one side of the cell membrane to the other. These proteins are widely distributed in all kingdoms of life, including bacteria, animals and plants. The important structural features of this protein includes a hydrophobic exterior that allows for embedding in the cell membrane, and a hydrophilic core that allows for the passage of water from one side of the cell to the other. Let's take a closer look at this protein. We will use this as a model for the important relationship between protein structure and function that holds true for all proteins and their varied roles in the cell. Theme 1 Module 3 Script 13 Slide 40: Each aquaporin is made up 4 protein subunits YOUR SUMMARY NOTES: (monomers) that together form the tetrameric aquaporin channel (its quaternary structure). This structural organization is important as it relates to the protein function. Each subunit contains membrane-spanning alpha-helices that form a central pore. As a result, each monomer forms a functionally independent water pore, and allows water to move through in either direction. If we examine a single aquaporin monomer, we find that the centralized core allows for several water molecules to move simultaneously through the channel. Interestingly aquaporin proteins do not undergo any changes in shape while transporting water. Instead, each water molecule is able to form specific hydrogen bonds with hydrophilic amino acid side groups that line the core of the monomer, displacing nearby water molecules that are passing ahead through the channel. Slide 41: Similarly, prokaryotic cells have membrane pores as well. In this example of a bacterial porin we can see the use of beta sheets to form a barrel with a hydrophobic exterior and hydrophilic interior. It is interesting that this porin structure is seen in the membranes of mitochondria and chloroplasts as well, further evidence for their prokaryotic ancestry. Slide 42: Proteins carry out many essential functions. This means that the genetic information to produce the proteins has to be encoded in our DNA, that these proteins need to be properly made in our bodies, and that they need to be sent to the appropriate locations where they will fulfill important functions. Cystic fibrosis is a genetic disease that causes the accumulation of mucus in many organs. Cystic fibrosis has been linked to mutations that lead to malfunction of an important cystic fibrosis transmembrane conductance regulator (or CFTR) ion channel protein. This leads to many consequences. In particular, one such consequence is seen in the accumulation of thick mucus in the lungs that can then result in bacterial infections. Slide 43: Checkpoint question #4 Theme 1 Module 3 Script 14 Slide 44: YOUR SUMMARY NOTES: we have learned that Cytosolic and ER- bound ribosomes synthesize proteins. we have also examined how proteins fold into diverse structures that relate to their function. and how the endomembrane system further processes and transports some proteins. Finally, we have identified how Proteins are delivered to various sites in the cell where they will fulfill various important cellular functions.