The imprecise nature of V.docx
Document Details
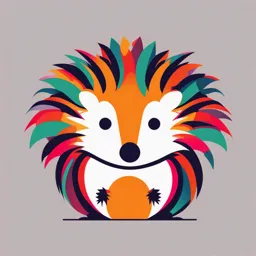
Uploaded by VigilantJasper
Full Transcript
The imprecise nature of V(D)J recombination is a double-edged sword. Although it produces increased diversity in the antibody repertoire, it also results in many unsuccessful rearrangements. Pro-B cells therefore need a way of testing whether a potentially functional heavy chain has been produced. T...
The imprecise nature of V(D)J recombination is a double-edged sword. Although it produces increased diversity in the antibody repertoire, it also results in many unsuccessful rearrangements. Pro-B cells therefore need a way of testing whether a potentially functional heavy chain has been produced. They do this by incorporating a functional heavy chain into a receptor that can signal its successful production. This test takes place in the absence of light chains, whose loci have not yet rearranged. Instead, pro-B cells make two invariant ‘surrogate’ proteins that together have a structural resemblance to the light chain and can pair with the μ chain to form the pre-B-cell receptor (pre-BCR) (see Fig. 8.5). The assembly of a pre-B-cell receptor signals to the B cell that a productive rearrangement has been made, and the cell is then considered a pre-B cell. The surrogate chains are encoded by nonrearranging genes separate from the antigen-receptor loci, and their expression is induced by E2A and EBF (see Fig. 8.4). One is called λ5 because of its close resemblance to the C domain of the λ light chain; the other, called VpreB, resembles a light-chain V domain but has an extra region at the amino-terminal end. Pro-B cells and pre-B cells also express the invariant proteins Igα and Igβ, introduced in Chapter 7 as the signaling components of the B-cell receptor complex on mature B cells. As components of the pre-B-cell receptor, Igα and Igβ transduce signals by interacting with intracellular tyrosine kinases through their cytoplasmic tails, just Formation of the pre-B-cell receptor and signaling through this receptor provide an important checkpoint that mediates the transition between the pro-B cell and the pre-B cell. In mice that either lack λ5 or have mutant heavy-chain genes that cannot produce the transmembrane domain, the pre-B-cell receptor cannot be formed and B-cell development is blocked after heavy-chain gene rearrangement. In normal B-cell development, the pre-B-cell receptor complex is expressed transiently, perhaps because the production of λ5 mRNA stops as soon as pre-B-cell receptors begin to be formed. Although present at only low levels on the cell surface, the pre-B-cell receptor generates signals required for the transition from pro-B cell to pre-B cell. No antigen or other external ligand seems to be involved in signaling by the receptor. Instead, pre-B-cell receptors are thought to interact with each other, forming dimers or oligomers that generate signals as described in Section 7-16. Dimerization involves ‘unique’ regions in the amino termini of λ5 and VpreB proteins that are not present in other immunoglobulin-like domains and which mediate the cross-linking of adjacent pre-B-cell receptors on the cell surface (Fig. 8.6). Pre- B-cell receptor signaling requires the scaffold protein BLNK and Bruton’s tyrosine kinase (Btk), an intracellular Tec-family tyrosine kinase (see Section 7-20). In humans and mice, deficiency of BLNK leads to a block in B-cell development at the pro-B-cell stage. In humans, mutations in the BTK gene cause a profound B-lineage-specific immune deficiency, Bruton’s X-linked agammaglobulinemia (XLA), in which no mature B cells are produced. The block in B-cell development caused by mutations in BTK is almost total, interrupting the transition from pre-B cell to immature B cell. A similar, but less severe, defect called X-linked immunodeficiency, or xid, arises from mutations in the Btk gene in mice. Pre-B cells rearrange the light-chain locus and express cellsurface immunoglobulin. The transition from the pro-B-cell to the large pre-B-cell stage is accompanied by several rounds of cell division, expanding the population of cells with successful in-frame joins by about 30- to 60-fold before they become resting small pre-B cells. A large pre-B cell with a particular rearranged heavy-chain gene therefore gives rise to numerous small pre-B cells. RAG proteins are produced again in the small pre-B cells, and rearrangement of the light-chain locus begins. Each of these cells can make a different rearranged light-chain gene, and so cells with many different antigen specificities are generated from a single pre-B cell, which makes an important contribution to overall B-cell receptor diversity. Light-chain rearrangement also exhibits allelic exclusion. Rearrangements at the light-chain locus generally take place at only one allele at a time, a process regulated by a mechanism not currently understood. The lightchain loci lack D segments, and rearrangement occurs by V to J joining; and if a particular VJ rearrangement fails to produce a functional light chain, repeated rearrangements of unused V and J gene segments at the same allele can occur (Fig. 8.8). Several attempts at productive rearrangement of a lightchain gene can therefore be made on one chromosome before initiating any rearrangements on the second chromosome. This greatly increases the chances of eventually generating an intact light chain, especially as there are two different light-chain loci. As a result, many cells that reach the pre- B-cell stage succeed in generating progeny that bear intact IgM molecules and can be classified as immature B cells. Figure 8.4 lists some of the proteins involved in V(D)J recombination and shows how their expression is regulated throughout B-cell development. Figure 8.5 summarizes the stages of B-cell development up to the point of assembly of a complete surface immunoglobulin. Developing B cells that fail to assemble a complete surface immunoglobulin undergo apoptosis in the bone marrow, and are eliminated from the B-cell pool. light chains also display isotypic exclusion, that is, the expression of only one type of light chain—κ or λ—by an individual B cell. Again, the mechanism regulating this process is not known. In mice and humans, the κ light-chain locus tends to rearrange before the λ locus. This was first deduced from the observation that myeloma cells secreting λ light chains generally have both their κ and λ light-chain genes rearranged, whereas in myelomas secreting κ light chains, generally only the κ genes are rearranged. This order is occasionally reversed, however, and λ gene rearrangement does not absolutely require the previous rearrangement of the κ genes. The ratios of κ-expressing versus λ-expressing mature B cells vary from one extreme to the other in different species. In mice and rats it is 95% κ to 5% λ, in humans it is typically 65%:35%, and in cats it is 5%:95%, the opposite of that in mice. These ratios correlate most strongly with the number of functional Vκ and Vλ gene segments in the genome of the species. They also reflect the kinetics and efficiency of gene segment rearrangements. The κ:λ ratio in the mature lymphocyte population is useful in clinical diagnostics, because an aberrant κ:λ ratio indicates the dominance of one clone and the presence of a lymphoproliferative disorder. 8-6 Immature B cells are tested for autoreactivity before they leave the bone marrow. Once a rearranged light chain has paired with a μ chain, IgM can be expressed on the cell surface (as a surface IgM, or sIgM) and the pre-B cell becomes an immature B cell. At this stage, the antigen receptor is first tested for reactivity to self antigens, or autoreactivity. The elimination or inactivation of autoreactive B cells ensures that the B-cell population as a whole will be tolerant of self antigens. The tolerance produced at this stage of B-cell development is known as central tolerance because it arises in a central lymphoid organ, the bone marrow. However, B cells leaving the bone marrow are not fully mature and require additional maturation steps that take place in peripheral lymphoid organs (see Section 8-8). As we shall see later in the chapter and in Chapter 15, self-reactive B cells that escape central tolerance may still be removed from the repertoire after they have left the bone marrow, a process that takes place during the final peripheral stages of B-cell maturation, and is referred to as peripheral tolerance, described in Section 8-7. sIgM associates with Igα and Igβ to form a functional B-cell receptor complex, and the fate of an immature B cell in the bone marrow depends on signals delivered from this receptor complex when it interacts with ligands in the environment. Igα signaling is particularly important in dictating the emigration of B cells from the bone marrow and/or their survival in the periphery: mice that express Igα with a truncated cytoplasmic domain that cannot signal show a fourfold reduction in the number of immature B cells in the marrow, and a hundredfold reduction in the number of peripheral B cells. The release of immature B cells from the bone marrow into the circulation is also dependent on their expression of S1PR1, a G-protein-coupled receptor that binds to the lipid ligand S1P and promotes cell migration towards the high concentrations of S1P that exist in the blood (see Section 8-27). Immature B cells that have no strong reactivity to self antigens continue to mature (Fig. 8.9, first panel). They leave the marrow via sinusoids that enter the central sinus, enter the circulation, and are carried by the venous blood supply to the spleen. If, however, the newly expressed receptor encounters a strongly cross-linking antigen in the bone marrow—that is, if the B cell is strongly self-reactive—development is arrested at this stage. Experiments using genetically modified mice that enforce the expression of self-reactive B-cell receptors have shown that there are four possible fates for self-reactive immature B cells (see Fig. 8.9, last three panels). These fates are the production of a new receptor by a process known as receptor editing; cell death by apoptosis, resulting in clonal deletion; the induction of a permanent state of unresponsiveness to antigen, or anergy; and a state of immunological ignorance in which antigen concentrations are too low to stimulate B-cell receptor signaling. The outcome for each self-reactive B cell is dependent on the interaction of the B-cell receptor with the self antigen. Immature B cells that express an autoreactive receptor recognizing a multivalent self antigen can be rescued by further gene rearrangements that replace the autoreactive receptor with a new receptor that is not self-reactive. This mechanism is termed receptor editing (Fig. 8.10). When an immature B cell first produces sIgM, RAG proteins are still being made. If the receptor is not self-reactive, the absence of sIgM cross-linking allows gene rearrangement to cease and B-cell development continues, with RAG proteins eventually disappearing. For an autoreactive receptor, however, an encounter with the self antigen results in strong cross-linking of sIgM; RAG expression continues, and light-chain gene rearrangement can continue, as described in Fig. 8.8. These secondary rearrangements can rescue immature self-reactive B cells by deleting the self-reactive light-chain gene and replacing it with another sequence. If the new light chain is not autoreactive, the B cell continues normal development. If the receptor remains autoreactive, rearrangement continues until a non-autoreactive receptor is produced or until no additional light-chain V and J gene segments are available for recombination. The importance of receptor editing as a mechanism of tolerance is well established, as defects in this process contribute to the human autoimmune diseases systemic lupus erythematosus and rheumatoid arthritis, two diseases characterized by high levels of autoreactive antibodies (see Chapter 15). It was originally thought that the successful production of a heavy chain and a light chain caused the almost instantaneous shutdown of light-chain locus rearrangement and that this ensured both allelic and isotypic exclusion. The unexpected ability of self-reactive B cells to continue to rearrange their lightchain genes, even after having made a productive rearrangement, suggests an alternative mechanism of allelic exclusion, where the fall in the level of RAG proteins that follows a successful non-autoreactive rearrangement could be the principal means by which light-chain rearrangement is terminated. It is now apparent that allelic exclusion is not absolute, as there are rare B cells that express two different light chains. Cells that remain autoreactive when receptor editing efforts fail to generate a non-autoreactive receptor undergo a process known as clonal deletion, in which they are subjected to cell death by apoptosis to eliminate their specific autoreactivity from the repertoire. Early experiments using transgenic mice expressing both chains of an immunoglobulin specific for H-2Kb MHC class I molecules, in which nearly all developing B cells expressed the anti-MHC immunoglobulin as sIgM, suggested that clonal deletion was a predominant mechanism of B-cell tolerance. These studies found that transgenic mice not expressing H-2Kb had normal numbers of B cells, all bearing the transgene-encoded anti-H-2Kb receptors. However, in mice expressing both H-2Kb and the immunoglobulin transgenes, B-cell development was blocked. Normal numbers of pre-B cells and immature B cells were found, but B cells expressing the anti-H-2Kb immunoglobulin as sIgM never matured to populate the spleen and lymph nodes; instead, most of these immature B cells died in the bone marrow by apoptosis. However, more recent studies, using mice bearing transgenes for autoantibody heavy and light chains that have been placed within the immunoglobulin loci by homologous recombination (see Appendix I, Section A-35, for details of this method), indicate that receptor editing, rather than clonal deletion, is the more likely outcome for immature autoreactive B cells. We have so far discussed the fate of newly formed B cells that undergo multivalent cross-linking of their sIgM. Immature B cells that encounter more weakly cross-linking self antigens of low valence, such as small soluble proteins, respond differently. In this situation, some self-reactive B cells are inactivated and enter a state of permanent unresponsiveness, or anergy, but do not immediately die (see Fig. 8.9). Anergic B cells cannot be activated by their specific antigen even with help from antigen-specific T cells. Again, this phenomenon was elucidated using transgenic mice. Hen egg-white lysozyme (HEL) was expressed in soluble form from a transgene in mice that were also transgenic for high-affinity anti-HEL immunoglobulin. The HEL-specific B cells matured and emigrated from the bone marrow, but could not respond to antigen. Furthermore, the migration of anergic B cells is impaired, as the cells are detained in the T-cell areas of peripheral lymphoid tissues and are excluded from lymphoid follicles, thereby reducing their life-span and their ability to compete with immunocompetent B cells (described further in Section 8-8). Under normal circumstances, where few self-reactive anergic B cells successfully mature, these cells die relatively quickly. This mechanism ensures that the long-lived pool of peripheral B cells is purged of potentially self-reactive cells. The fourth potential fate of self-reactive immature B cells is that nothing happens to them; they remain in a state of immunological ignorance of their self antigen (see Fig. 8.9). Immunologically ignorant cells have affinity for a self antigen but for various reasons do not sense and respond to it. The antigen may not be accessible to developing B cells in the bone marrow or spleen, or may be in low concentration, or may bind so weakly to the B-cell receptor that it does not generate an activating signal. Because some ignorant cells can be (and in fact are) activated under certain conditions such as inflammation or when the self antigen becomes available or reaches an unusually high concentration, they should not be considered inert, and they are fundamentally different from cells with non-autoreactive receptors that could never be activated by self antigens. The fact that central tolerance is not perfect and some self-reactive B cells are allowed to mature reflects the balance that the immune system strikes between purging all self-reactivity and maintaining the ability to respond to pathogens. If the elimination of self-reactive cells were too efficient, the receptor repertoire might become too limited and thus unable to recognize a wide variety of pathogens. Some autoimmune disease is the price of this balance: we shall see in Chapter 15 that ignorant self-reactive lymphocytes can be activated and cause disease under certain circumstances. Normally, however, ignorant B cells are held in check by a lack of T-cell help, the continued inaccessibility of the self antigen, or the tolerance that can be induced in mature B cells following their emigration from the bone marrow, which is described below. 8-7 Lymphocytes that encounter sufficient quantities of self antigens for the first time in the periphery are eliminated or inactivated. While large numbers of autoreactive B cells are purged from the population of new lymphocytes in the bone marrow, only lymphocytes specific for autoantigens that are expressed in or can reach this organ are affected. Some antigens, like the thyroid product thyroglobulin, are highly tissue specific, or are compartmentalized so that little if any is available in the circulation. Therefore, newly emigrated self-reactive B cells that encounter their specific autoantigen must be eliminated or inactivated also. This tolerance mechanism, which acts on newly emigrated B cells that are still immature, is known as peripheral tolerance. Like self-reactive lymphocytes in the central lymphoid organs, lymphocytes that encounter self antigens de novo in the periphery can have several fates: deletion, anergy, or survival (Fig. 8.11). In the absence of an infection, newly emigrated B cells that encounter a strongly cross-linking antigen in the periphery will undergo clonal deletion. This was elegantly shown in studies of B cells expressing B-cell receptors specific for H-2Kb MHC class I molecules. These B cells are deleted even when, in transgenic animals, the expression of the H-2Kb molecule is restricted to the liver by the use of a liver-specific gene promoter. There is no receptor editing: B cells that encounter strongly cross-linking antigens in the periphery undergo apoptosis directly, unlike their counterparts in the bone marrow, which attempt further receptor rearrangements. This difference may be due to the fact that the B cells in the periphery are somewhat more mature and can no longer rearrange their light-chain loci. As with immature B cells in the bone marrow, newly developed peripheral B cells that encounter and bind an abundant soluble antigen become unresponsive. This was demonstrated in mice by placing the HEL transgene under the control of an inducible promoter that can be regulated by changes in the diet. It is thus possible to induce the production of lysozyme at any time and thereby study its effects on HEL-specific B cells at different stages of maturation. These experiments have shown that both peripheral and immature bone marrow B cells are inactivated when they are chronically exposed to soluble antigen. 8-8 Immature B cells arriving in the spleen turn over rapidly and require cytokines and positive signals through the B-cell receptor for maturation and long-term survival. When B cells emerge from the bone marrow into the periphery, they are still functionally immature. As discussed above, their final maturation in the periphery provides an opportunity for the immature B cells to encounter peripheral self antigens and to undergo tolerance. Immature B cells express high levels of sIgM but little sIgD, whereas mature B cells express low levels of IgM and high levels of IgD; while the changes in expression of sIgM and sIgD as B cells mature is well documented, the function of sIgD on mature B cells is not known. Most immature B cells leaving the bone marrow will not survive to become fully mature B cells. Figure 8.12 shows the possible fates of newly produced B cells that enter the periphery. The daily output of new B cells from the bone marrow is roughly 5–10% of the total B-lymphocyte population in the steadystate peripheral pool. In unimmunized animals, the size of this pool seems to remain constant, due to homeostasis, which means that the stream of new B cells needs to be balanced by the removal of an equal number of peripheral B cells. However, the majority of peripheral mature B cells are long-lived, and only 1–2% of these die each day. Thus, most of the B cells that die are in the rapidly turning-over immature peripheral B-cell population, of which more than 50% die every 3 days. The failure of most newly formed B cells to survive for more than a few days in the periphery seems to be due to competition between peripheral B cells for access to the follicles in the spleen. If newly produced immature B cells do not enter a follicle, their passage through the periphery is halted and they eventually die. The limited number of lymphoid follicles cannot accommodate all of the B cells generated each day, and so there is continual competition for entry. The follicle provides signals necessary for B-cell survival. In particular, the TNF-family member BAFF (for B-cell activating factor belonging to the TNF family) is made by several cell types, but is produced abundantly by the follicular dendritic cells (FDCs). FDCs are non-hematopoietic cells resident in the B-cell follicles that are specialized to capture antigens for recognition by B-cell antigen receptors (see Section 9-1). B cells express three different receptors for BAFF, namely BAFF-R, BCMA, and TACI. The BAFF-R is the most important for follicular B-cell survival, because mutant mice lacking BAFF-R have mainly immature B cells and few long-lived peripheral B cells. BCMA and TACI also bind the related TNF family cytokine APRIL, which is not required for the survival of immature B cells but is important for IgA antibody production, as we shall see in Chapter 10. Summary. The stages of thymocyte development up to the expression of the pre-T-cell receptor—including the decision between commitment to either the α:β or the γ:δ lineage—are all independent of interactions with peptide:MHC antigens. With the successful rearrangement of α-chain genes and expression of the T-cell receptor, α:β thymocytes undergo further development that is determined by the interactions of their T-cell receptors with self peptides presented by the MHC molecules on the thymic stroma. CD4+CD8+ doublepositive thymocytes whose receptors interact with self peptide:self MHC complexes on thymic cortical epithelial cells are positively selected, and will eventually mature into CD4 or CD8 single-positive cells. T cells that react too strongly with self antigens are deleted in the thymus, a process driven by bone marrow-derived antigen-presenting cells and AIRE-expressing epithelial cells in the medullary region of the thymus. The outcome of positive and negative selection is the generation of a mature conventional T-cell repertoire that is both MHC-restricted and self-tolerant. Some non-conventional T-cell lineages undergo ‘agonist’ selection following strong T-cell receptor signaling. Precisely how the recognition of self peptide:self MHC ligands by the T-cell receptor leads to either positive or negative selection remains an unsolved problem. Summary to Chapter 8. In this chapter we have learned about the formation of the B-cell and T-cell lineages from an uncommitted hematopoietic stem cell. The somatic gene rearrangements that generate the highly diverse repertoire of antigen receptors—immunoglobulin for B cells, and the T-cell receptor for T cells— occur in the early stages of the development of T cells and B cells from a common bone marrow-derived lymphoid progenitor. Mammalian B-cell development takes place in fetal liver and, after birth, in the bone marrow; T cells also originate from stem cells in the fetal liver or the bone marrow, but undergo most of their development in the thymus. Much of the somatic recombination machinery, including the RAG proteins that are an essential part of the V(D)J recombinase, is common to both B and T cells. In both B and T cells, gene rearrangements begin with the loci that contain D gene segments, and proceed successively at each locus. The first step in B-cell development is the rearrangement of the locus for the immunoglobulin heavy chain, and for T cells the β chain. In each case, the developing cell is allowed to proceed to the next stage of development only if the rearrangement has produced an in-frame sequence that can be translated into a protein expressed on the cell surface: either the pre-B-cell receptor or the pre-T-cell receptor. Cells that do not generate successful rearrangements for both receptor chains die by apoptosis. The course of conventional B-cell development is summarized in Fig. 8.14, and that of α:β T cells in Fig. 8.33. Once a functional antigen receptor has appeared on the cell surface, the lymphocyte is tested in two ways. Positive selection tests for the potential usefulness of the antigen receptor, whereas negative selection removes self-reactive cells from the lymphocyte repertoire, rendering it tolerant to the antigens of the body. Positive selection is particularly important for T cells, because it ensures that only cells bearing T-cell receptors that can recognize antigen in combination with self MHC molecules will continue to mature. Positive selection also coordinates the choice of co-receptor expression. CD4 becomes expressed by T cells harboring MHC class II-restricted receptors, and CD8 by cells harboring MHC class I-restricted receptors. This ensures the optimal use of these receptors in responses to pathogens. For B cells, positive selection seems to occur at the final transition from immature to mature B cells, which occurs in peripheral lymphoid tissues. Tolerance to self antigens is enforced by negative selection at different stages throughout the development of both B and T cells, and positive selection likewise seems to represent a continuous process