Eddy Current Testing PDF
Document Details
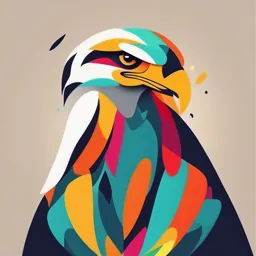
Uploaded by PopularOrientalism
2024
Rob Bilney
Tags
Summary
This document is a technical guide on Eddy Current Testing, covering introduction, principles, factors affecting eddy currents (conductivity, permeability, frequency, geometry, proximity), and applications in aerospace. It includes diagrams and tables to illustrate the concepts.
Full Transcript
Number : TESTIA-UK-TRA-ET-003-EN Issue: 3.0 Date: 06/03/2024 Approvals: Compiled By: Approved By:...
Number : TESTIA-UK-TRA-ET-003-EN Issue: 3.0 Date: 06/03/2024 Approvals: Compiled By: Approved By: Authorisation: Rob Bilney Keith Phillips Ajay Surendran Amendment History: Issue No Reason Date 1.0 Initial release 29/01/2014 2.0 Transfer to new template 25/07/2022 3.0 Logo change and addition of review table 06/03/2024 Template Ref.: TESTIA-UK-GEN-006-F01-EN issue 2 UNCONTROLLED WHEN PRINTED Page 1 of 2 © Testia Ltd. – all rights reserved Number : TESTIA-UK-TRA-ET-003-EN Issue: 3.0 Date: 06/03/2024 CONTENTS Chapter No Title Issue Issue date Latest Reviewed Review by Chapter 1 INTRODUCTION AND 02 25-07-22 06-03-24 Robin FACTORS AFFECTING Bilney (RB) EDDY CURRENT Chapter 2 IMPEDANCE PLANE 02 25-07-22 06-03-24 RB ANALYSIS Chapter 3 COILS AND PROBES 03 06-03-24 06-03-24 RB Chapter 4 ELECTRICAL THEORY 03 06-03-24 06-03-24 RB Chapter 5 TYPES OF TEST 03 06-03-24 06-03-24 RB EQUIPMENT Chapter 6 PRACTICAL 02 25-07-22 06-03-24 RB APPLICATIONS Chapter 7 WI AND REPORT 02 25-07-22 06-03-24 RB WRITING APPENDICES Appendix Title Issue Date Latest Reviewed No Review by Appendix A GLOSSARY OF 02 25-07-22 06-03-24 RB TERMS Template Ref.: TESTIA-UK-GEN-006-F01-EN issue 2 UNCONTROLLED WHEN PRINTED Page 2 of 2 © Testia Ltd. – all rights reserved Chapter 1 Page 1 of 17 EDDY CURRENT TESTING CHAPTER 1 INTRODUCTION AND FACTORS AFFECTING EDDY CURRENTS CONTENTS Paragraphs 1. Introduction.................................................................................................................................... 3 1.1. Principles of Non-Destructive Testing in Aerospace............................................................... 3 1.2. Definition................................................................................................................................ 4 1.3. History of Eddy Current Testing.............................................................................................. 4 1.4. Applications............................................................................................................................. 5 1.5. Capabilities.............................................................................................................................. 5 1.6. Advantages.............................................................................................................................. 6 1.7. Limitations............................................................................................................................... 6 1.8. Surface Preparation................................................................................................................ 6 2. Electromagnetic induction.............................................................................................................. 7 3. The production of Eddy Currents.................................................................................................... 7 4. The magnetic effects of Eddy Currents........................................................................................... 8 5. Impedance...................................................................................................................................... 9 6. Factors affecting Eddy Currents...................................................................................................... 9 6.1. Conductivity............................................................................................................................ 9 Heat treatment........................................................................................................................... 9 Chemical composition................................................................................................................. 9 Temperature............................................................................................................................... 9 6.2. Permeability.......................................................................................................................... 10 6.2.1. Ferrous Effect.................................................................................................................... 10 6.2.2. Hysteresis Loop................................................................................................................. 11 6.3. Frequency.............................................................................................................................. 13 6.3.1. Skin Effect.......................................................................................................................... 13 6.4. Geometry.............................................................................................................................. 14 6.4.1. Edge Effect........................................................................................................................ 14 Document Number: TESTIA - UK - TRA - ET – 003A - EN - Issue 2 Registered in England and Wales Testia Limited Company No. 08360678 Airbus Main Gate, Golf Course Lane, Building 07D, Filton, Bristol, UK BS34 7QQ Chapter 1 Page 2 of 17 6.4.2. Mass Effect........................................................................................................................ 15 6.4.3. Thickness Effect................................................................................................................. 15 6.5. Proximity............................................................................................................................... 16 6.6. Discontinuities....................................................................................................................... 16 6.7. Probe handling...................................................................................................................... 17 Tables Table 1: Comparison with other NDT methods...................................................................................... 6 Table 2: Typical conductivity, resistivity and relative permeability values........................................... 10 Diagrams Diagram 1: The principle of electromagnetic induction......................................................................... 7 Diagram 2: The primary magnetic field.................................................................................................. 7 Diagram 3: The production of Eddy Currents......................................................................................... 8 Diagram 4: The secondary magnetic field.............................................................................................. 8 Diagram 5: Ferrous effect..................................................................................................................... 11 Diagram 6: Hysteresis loop................................................................................................................... 12 Diagram 7: Soft iron and hard steel hysteresis loop............................................................................. 12 Diagram 8: The skin effect.................................................................................................................... 13 Diagram 9: High and low frequency eddy current scanning................................................................. 13 Diagram 10: Edge effect........................................................................................................................ 14 Diagram 11: Mass effect....................................................................................................................... 15 Diagram 12: Thickness effect................................................................................................................ 15 Diagram 13: Proximity.......................................................................................................................... 16 Diagram 14: Discontinuities.................................................................................................................. 16 Diagram 15: Probe handling................................................................................................................. 17 Document Number: TESTIA - UK - TRA - ET – 003A - EN - Issue 2 Registered in England and Wales Testia Limited Company No. 08360678 Airbus Main Gate, Golf Course Lane, Building 07D, Filton, Bristol, UK BS34 7QQ Chapter 1 Page 3 of 17 1. Introduction 1.1. Principles of Non-Destructive Testing in Aerospace Non-Destructive Testing (NDT) is a term that embraces all methods of examining materials in order to detect flaws, or measure properties without affecting the serviceability of that material. The aims of Non-Destructive Testing in aerospace are: To offer aircraft operators specialist examination methods. Among other things, these specialist methods can be used to check components for integrity, correct assembly and functioning when stripping is impossible or might destroy evidence. To use specialist methods to carry out examinations, which if done in the normal way i.e. stripping and visually examining, would take too many man-hours, cost too much, and reduce aircraft availability. To enhance flight safety by ensuring the structural integrity of aircraft. Just like repeatedly bending a stick one way and then the other to break it, aircraft structures are constantly subjected to alternating loads, vibrations, pressurizations, take offs and landings etc. Essentially, loads applied in one direction and then another progressively weaken the structure causing it to crack and eventually fail and this mode of failure is known as fatigue failure. There are two principles of aircraft design intended to overcome fatigue failure: Safe-Life Design. A 'Safe-Life' structure is one in which no significant fatigue cracking is permitted during the design life of the aircraft. To prove the Safe-Life of an in-service aircraft, an on-going comprehensive ‘Major Airframe Fatigue Test' (MAFT) rig is run by the manufacturers to simulate all the loads that flying will impose on the aircraft structure. Any failure on the MAFT will require modification action on the fleet. It is, therefore, essential that the MAFT is running ahead of the aircraft fleet, in particular the 'fleet leader' (that aircraft with the highest consumption of fatigue life) so that sufficient time is given for modification action and the development of inspection procedures, including NDT. In practice the Safe-Life theory is an inexact science and failures in-service still occur. True flight loads and environmental conditions can never be fully simulated in a test rig and in a reverse situation failures have occurred on the MAFT, which never manifested themselves in the fleet. Damage-Tolerant Design. Unlike small aircraft, it is relatively easy to design large transport aircraft for ease of inspection; therefore they have frequently been designed to damage- tolerant principles. In this case, the designer ensures that defects can exist for some time before they become critical by providing redundant load paths, introducing crack-arrest features, designing the structure so that stress levels are low and choosing materials so that crack lengths are long. A target crack free life may be specified for a damage tolerant aircraft in terms of a convenient parameter such as flights or landings. Document Number: TESTIA - UK - TRA - ET – 003A - EN - Issue 2 Registered in England and Wales Testia Limited Company No. 08360678 Airbus Main Gate, Golf Course Lane, Building 07D, Filton, Bristol, UK BS34 7QQ Chapter 1 Page 4 of 17 Damage-tolerant aircraft are not given a Safe Life fatigue. Airworthiness is guaranteed by inspection. There are many reasons for carrying out Non-Destructive Testing of a particular component or aircraft structure. The requirement could arise from: The detection of flaws found during the fatigue rig-testing programme for an aircraft. The discovery of flaws found during scheduled servicing, repair or reconditioning of aircraft. The investigation of structural failures in service aircraft. The following methods of examination are some of the more common methods used in aerospace Non-Destructive Testing: Visual and visual assisted inspection. Penetrant Testing (PT). Magnetic Particle Testing (MT). Eddy Current Testing (ET). Ultrasonic Testing (UT). Radiographic Testing (RT). 1.2. Definition Eddy Current Testing (ET) is an NDT discipline that uses the effect of electromagnetic induction to induce currents in the component being tested. In turn, these currents are affected by the presence of discontinuities which can then be detected by electronics means. 1.3. History of Eddy Current Testing The phenomenon of eddy currents was discovered by French physicist Leon Foucault in 1851, and for this reason eddy currents are sometimes called Foucault currents. Foucault built a device that used a copper disk moving in a strong magnetic field to show that an electrical current is generated when a conducting material moves within an applied magnetic field. Eddy current testing began largely as a result of the English scientist Michael Faraday's discovery of electromagnetic induction in 1831. Faraday discovered that when a magnetic field passes a conductor (a material in which electrons move easily) or when a conductor passes through a magnetic field, an electric current will flow through the conductor if there is a closed path through Document Number: TESTIA - UK - TRA - ET – 003A - EN - Issue 2 Registered in England and Wales Testia Limited Company No. 08360678 Airbus Main Gate, Golf Course Lane, Building 07D, Filton, Bristol, UK BS34 7QQ Chapter 1 Page 5 of 17 which the current can circulate. In 1879, another breakthrough was made when another English scientist, David Hughes, demonstrated how the properties of a coil change when placed in contact with metals of different conductivity and permeability. However, it was not until the Second World War that these developments in the transmitting and receiving of electromagnetic waves were put to practical use for materials testing. Beginning in 1933, in Germany, while working for the Kaiser-Wilhelm-Institute, Professor Friedrich Förster adapted eddy current technology to industrial use, developing instruments for measuring conductivity and for sorting mixed-up ferrous components. In 1948, Förster founded his own company in Reutlingen, a business based on eddy current testing that continues to this day. Other companies soon followed. Many advances were made throughout the 1950s and 1960s, especially in the aircraft and nuclear industries. There have been many recent developments in eddy current testing, leading to improved performance and the development of new applications. Eddy current testing is now a widely used and well-understood inspection technique for flaw detection as well as for thickness and conductivity measurements. 1.4. Applications Eddy current techniques may be applied to a variety of geometrical shapes including wires, tubes, bars, cylinders, sheet metal and many other forms into which materials are fashioned during their cast or wrought stages of production. It is used in all sectors of engineering (Aerospace, oil + gas, nuclear, rail and power generation). Eddy current is not just used for ‘standard’ defects; it can be used in other applications such as corrosion detection, conductivity testing, paint thickness measurement and material sorting. 1.5. Capabilities Eddy Current techniques are capable of detecting both surface and, to some extent, subsurface discontinuities associated with the many problems experienced in the production, working and in service use of metals. i.e. Inherent Problems: Discontinuities arising at the cast or wrought stages of production such as bursts, inclusions, laps and porosity. Processing Problems: Faults resulting from extruding, machining or welding such as stringers, grinding cracks, shrinkage cracks, poor heat treatment and inadequate stress relieving procedures. In-Service Problems: Flaws occurring during the normal operation of the component or material such as erosion, corrosion, wear and metal fatigue. Document Number: TESTIA - UK - TRA - ET – 003A - EN - Issue 2 Registered in England and Wales Testia Limited Company No. 08360678 Airbus Main Gate, Golf Course Lane, Building 07D, Filton, Bristol, UK BS34 7QQ Chapter 1 Page 6 of 17 1.6. Advantages Eddy Current testing is advantageous (Table 1) in that it provides instantaneous results with minimal surface preparation using portable equipment which may also be readily adapted to automation. It is also intrinsically safe as an inspection tool, requiring only the coupling of an alternating magnetic field with the component under test to provide the interrogatory medium, thus causing no damage to the material and presenting no health hazards to the operator. Flaw to be Material to be Flaw Methods Advantages Disadvantages detected inspected Orientation Removal of the Cheap, simple, coating, chemicals Open to the Minimal PT All except porous Any used and unable surface equipment to use on rough required surfaces Open to the Perpendicular surface and Instant results, Ferrous materials MT Ferromagnetic to the magnetic near the cheap only field surface Deep sub Any material Ionising radiation surface defects, Open to the (although some hazardous to Parallel to the X few restraints, RT surface and produce better health, expensive, ray beam can image Internal results than Access difficulties, multi layer others) Slow results products Open to the Instant results, Perpendicular surface and Electrically minimal Conductive metals ET to scan near the conductive preparation, only direction surface accurate High degree of Open to the Deep sub Perpendicular interpretation skill UT surface and Any material surface defects, to sound path required, surface Internal Instant results condition critical Table 1: Comparison with other NDT methods 1.7. Limitations Eddy Current testing is limited to those metals which are considered to be conductors of electrical currents. For a comparison of eddy current against other NDT methods please see Table 1. 1.8. Surface Preparation There is no electrical connection required between the probe and the item under inspection, therefore the surface finish does not necessarily need to be removed. Changes in the distance between the probe and the item will affect the inspection so the surface finish must be even, not Document Number: TESTIA - UK - TRA - ET – 003A - EN - Issue 2 Registered in England and Wales Testia Limited Company No. 08360678 Airbus Main Gate, Golf Course Lane, Building 07D, Filton, Bristol, UK BS34 7QQ Chapter 1 Page 7 of 17 too thick (limits as stated in the procedure) and in good contact with the surface i.e. not flaking, for effective Eddy Current Testing to be carried out. The surface needs to be free from obstructions i.e. sealant, shims, swarf (particularly ferrous swarf), loose or flaking paint etc and then cleaned and degreased with an approved cleaning product. 2. Electromagnetic induction In accordance with Faraday’s Law, when an electrically conducting circuit is linked by a changing magnetic field, an electromotive force (EMF) is induced which causes a current to flow within the electrical circuit. The induced EMF, and hence current, is proportional to the rate of change of the flux linkage (Diagram 1). Ammeter Movement Movement Current flow N S Diagram 1: The principle of electromagnetic induction 3. The production of Eddy Currents When an alternating current is passed through a conductor wound into a coil, a changing magnetic field is produced. This is called the primary magnetic field, often referred to as the primary field (Diagram 2). Diagram 2: The primary magnetic field Document Number: TESTIA - UK - TRA - ET – 003A - EN - Issue 2 Registered in England and Wales Testia Limited Company No. 08360678 Airbus Main Gate, Golf Course Lane, Building 07D, Filton, Bristol, UK BS34 7QQ Chapter 1 Page 8 of 17 When the primary field from the coil is placed near to a component which is electrically conductive (nearly all metals), an EMF is induced in the component which cause currents to flow in circular paths linking the magnetic flux from the coil. These currents are Eddy Currents (Diagram 3). Diagram 3: The production of Eddy Currents 4. The magnetic effects of Eddy Currents It was explained in paragraph 2 that when a current flows through a conductor, a magnetic field is produced. Therefore, the eddy currents themselves also produce a magnetic field which is called the secondary magnetic field, or secondary field (Diagram 4). This secondary field is always orientated in such a direction as to oppose the effects of the primary field which induced the eddy currents originally. This is an example of Lenz’s law which states that the direction of an induced EMF is such that the magnetic field from the current it produces, will oppose the change in the magnetic field inducing the EMF. Diagram 4: The secondary magnetic field Document Number: TESTIA - UK - TRA - ET – 003A - EN - Issue 2 Registered in England and Wales Testia Limited Company No. 08360678 Airbus Main Gate, Golf Course Lane, Building 07D, Filton, Bristol, UK BS34 7QQ Chapter 1 Page 9 of 17 5. Impedance Impedance is the opposition to AC current flow in an electrical circuit. In a coil this impedance is partly due to resistance of the wire and partly due to the primary field which induces an EMF into the coil windings, which oppose the voltage applied to the coil. As the secondary field opposes the change in the primary field this will also affect the impedance of the coil. Therefore, any change in the eddy currents will change the impedance of the coils. It is this change of impedance that the instrument displays. 6. Factors affecting Eddy Currents Summarising the previous paragraphs, it can now be seen that the composition of the eddy currents affects the secondary field, which in turn opposes, and thus affects, the primary field. This affects the coil impedance which is detected and displayed by the instrument. Consider now the eddy currents, any change in them will ultimately show as an instrument display, and thus any factor that affects eddy currents will in turn also affect our instrument display and give us indications. Unfortunately, there are many factors that affect eddy currents, not just faults, and it is these factors that we will consider next. 6.1. Conductivity Although we may be more familiar with the general term resistance when referring to electrical circuits, there are other terms that have more specific applications. Resistivity (ρ) is defined as the resistance offered by a 1 metre cube of a material at 0°c. It is usually expressed in ohm metres. Thus, resistivity = RA / L where R is the resistance of a uniform conductor of length L and cross sectional area A. Conductivity is the reciprocal of resistivity and is a measure of the ease with which electrons will flow within a material. A material having a high conductivity, e.g. copper, will permit current to flow more than a material having a low conductivity, e.g. lead or non-metals. Thus more eddy currents will be induced in a material having high conductivity than one having low conductivity. Conductivity changes in materials can be caused by variations in: Heat treatment Chemical composition Temperature Instruments based upon eddy current detection can be used to measure conductivity, usually for the purpose of metal sorting or defining areas of heat damage. Eddy current instruments designed for measuring conductivity are calibrated in percentage International Annealed Copper Standard (IACS). This is a standard whereby a sample of pure copper is said to be 100% IACS, all other conductivities being compared to it. Table 2 gives examples of typical conductivity values. Document Number: TESTIA - UK - TRA - ET – 003A - EN - Issue 2 Registered in England and Wales Testia Limited Company No. 08360678 Airbus Main Gate, Golf Course Lane, Building 07D, Filton, Bristol, UK BS34 7QQ Chapter 1 Page 10 of 17 Resistivity Conductivity Relative Metal / Alloy Micro Ohms % IACS Permeability Metre Aluminium 65.1 0.0265 1 Cadmium 25 0.069 1 Copper 100 0.017 1 Gold 71.8 0.024 1 Lead 8.2 0.21 1 Magnesium 43.1 0.04 1 Silver 107.8 0.016 1 Titanium 3.3 0.53 1 Soft Iron 18.9 0.01 10,000 Mild Steel 11.5 0.15 2,000 Stainless Steel 1.79 0.96 1 18% Cr../8% Ni Typical Aluminium Alloy 34-42 0.051-0.041 1 Aircraft Skin Typical Aluminium Alloy 34-42 0.051-0.041 1 Aircraft Forging Typical Aluminium Alloy 9-36 0.192-0.048 1 Aircraft Wheel Table 2: Typical conductivity, resistivity and relative permeability values 6.2. Permeability Absolute magnetic permeability (μ) is the ratio of magnetic flux density (B) in a body to the external magnetic field strength (H) which produces it. Thus μ = B / H It is usual to refer to the relative permeability (μr) of materials. That is absolute permeability (μ) divided by permeability of a vacuum (μ0). Non magnetic materials have relative permeabilities of 1, whilst magnetic materials have values ranging from 1 to 10,000 (Table 2). 6.2.1. Ferrous Effect If the magnetic field from the coil couples with a ferro-magnetic material the magnetic flux density will be greater than if the material was non-magnetic, the actual density depending upon the relative permeability. This in turn will produce stronger eddy currents which in turn have a greater effect on the coil’s impedance. The effect is so great that most eddy current scanning is limited to non-magnetic materials. Problems arise in practice when scanning near to ferro-magnetic items such as steel bolts or ferrous swarf which swamp the effects from the non-magnetic material under test. Document Number: TESTIA - UK - TRA - ET – 003A - EN - Issue 2 Registered in England and Wales Testia Limited Company No. 08360678 Airbus Main Gate, Golf Course Lane, Building 07D, Filton, Bristol, UK BS34 7QQ Chapter 1 Page 11 of 17 This is known as the ferrous effect (Diagram 5). Despite these problems it is still possible to test ferro-magnetic materials. Diagram 5: Ferrous effect 6.2.2. Hysteresis Loop The hysteresis loop of material is a graphical representation of the magnetic properties of a material and is formed by plotting the changes in flux density (B) against variations in the magnetising force (H) as shown in Diagram 6. Starting at the origin with the material in the unmagnetised condition and increasing the magnetising force, the flux density increases as shown by the dotted line until point “a” is reached beyond which any increase in the magnetising force will not increase the flux density. At this point the material is magnetically saturated. If the magnetising force is reduced to zero again, the flux density reduces to point “b”. The value of the flux density at position “b” represents the amount of residual magnetism remaining in the material. When the magnetising force is reversed and increased in value, the flux density reduces to zero at point “c” and the coercive force (the reverse magnetising force required to remove the residual magnetism) is represented by the line O-C. Increasing the magnetism force still further results in saturation point being reached at point “d”, but this time in the opposite polarity to that at point “a”. On reducing the magnetising force to zero again, the amount of residual magnetism is indicated by the position of point “e”. When the magnetising force is again reversed and increased in value, the flux density reaches zero at point “f” and then increase in the opposite polarity until saturation at point “a” is reached. The cycle is now complete. There is a lag between the magnetising force and flux density throughout the cycle and this is called hysteresis. Diagram 7 shows two hysteresis loops for low and high permeability materials. Document Number: TESTIA - UK - TRA - ET – 003A - EN - Issue 2 Registered in England and Wales Testia Limited Company No. 08360678 Airbus Main Gate, Golf Course Lane, Building 07D, Filton, Bristol, UK BS34 7QQ Chapter 1 Page 12 of 17 Diagram 6: Hysteresis loop Soft Iron (high μ) Hard Steel (low μ) Diagram 7: Soft iron and hard steel hysteresis loop Document Number: TESTIA - UK - TRA - ET – 003A - EN - Issue 2 Registered in England and Wales Testia Limited Company No. 08360678 Airbus Main Gate, Golf Course Lane, Building 07D, Filton, Bristol, UK BS34 7QQ Chapter 1 Page 13 of 17 6.3. Frequency Consider a cross section through 2 identical conductors (Diagram 8). When direct current (DC) or, current produced by a low frequency voltage, flows through a conductor, the current density is uniform throughout its cross section. 6.3.1. Skin Effect With higher frequency alternating current (AC) the current density is greatest near the surface and gets less with distance into the material. The higher the frequency, the closer to the surface the electrons flow. This is known as the skin effect. DC AC Diagram 8: The skin effect In coils positioned perpendicular to the surface, eddy currents produced by a high frequency alternating magnetic field tend to concentrate nearer the surface of the component than eddy currents produced by a low frequency alternating magnetic field (Diagram 9). Diagram 9: High and low frequency eddy current scanning Document Number: TESTIA - UK - TRA - ET – 003A - EN - Issue 2 Registered in England and Wales Testia Limited Company No. 08360678 Airbus Main Gate, Golf Course Lane, Building 07D, Filton, Bristol, UK BS34 7QQ Chapter 1 Page 14 of 17 To give a measure of the depth of penetration we regard the Standard Depth of Penetration (SDP) as being the depth where the current density is 37% of the value at the surface and we use the formula detailed. In practice, we will still detect faults that lie beyond the SDP, therefore the effective depth of penetration (EDP) is greater than the SDP. 𝜌 SDP = 503 × √μ ×𝑓 r Where SDP = Standard deph of penetration 1.7241 𝜌= Resistivity (micro ohm metres) %𝐼𝐴𝐶𝑆 μr = Relative permeability f = Frequency (Hz) 6.4. Geometry The geometrical shape and dimensions of the component under test will affect the eddy currents. There are 3 effects associated with geometry: 6.4.1. Edge Effect As the probe approaches an edge, the eddy currents will be restricted and cause a spurious indication. In order to scan near the edge of a component, the equipment must be balanced at a set distance from the edge and a scan path carried out parallel to the edge (Diagram 10). Diagram 10: Edge effect Document Number: TESTIA - UK - TRA - ET – 003A - EN - Issue 2 Registered in England and Wales Testia Limited Company No. 08360678 Airbus Main Gate, Golf Course Lane, Building 07D, Filton, Bristol, UK BS34 7QQ Chapter 1 Page 15 of 17 6.4.2. Mass Effect If a scan is carried out near to a large mass, for example into a radius, the magnetic field around the sides of the probe will couple with the mass (Diagram 11). This increases the total amount of eddy current induced and causes a spurious indication. Practical methods of overcoming this problem will be dealt with later. Diagram 11: Mass effect 6.4.3. Thickness Effect When the frequency is such that the eddy currents penetrate the full thickness of the material (Diagram 12), any changes in thickness will give rise to indications. Diagram 12: Thickness effect Document Number: TESTIA - UK - TRA - ET – 003A - EN - Issue 2 Registered in England and Wales Testia Limited Company No. 08360678 Airbus Main Gate, Golf Course Lane, Building 07D, Filton, Bristol, UK BS34 7QQ Chapter 1 Page 16 of 17 6.5. Proximity The distance from the probe tip to the material being tested will determine how much of the coil’s magnetic flux couples with the material. Any change in this distance can cause indications (Diagram 13). This is referred to as lift off. Diagram 13: Proximity 6.6. Discontinuities Any discontinuities such as cracks, scores, corrosion pits, laps and inclusions etc, will disrupt the eddy currents and may cause an indication (Diagram 14). Diagram 14: Discontinuities Document Number: TESTIA - UK - TRA - ET – 003A - EN - Issue 2 Registered in England and Wales Testia Limited Company No. 08360678 Airbus Main Gate, Golf Course Lane, Building 07D, Filton, Bristol, UK BS34 7QQ Chapter 1 Page 17 of 17 6.7. Probe handling Variations in the angle that the probe makes with the surface being scanned can alter the magnetic coupling. The probe should therefore be held perpendicular to the surface both during calibration and during scanning of the component to ensure consistent results (Diagram 15). Furthermore, some probes can be susceptible to hand capacitance and these must be held the same way throughout the calibration and inspection. Diagram 15: Probe handling Document Number: TESTIA - UK - TRA - ET – 003A - EN - Issue 2 Registered in England and Wales Testia Limited Company No. 08360678 Airbus Main Gate, Golf Course Lane, Building 07D, Filton, Bristol, UK BS34 7QQ Chapter 2 Page 1 of 7 EDDY CURRENT TESTING CHAPTER 2 IMPEDANCE PLANE ANALYSIS CONTENTS Paragraphs 1. Introduction.................................................................................................................................... 2 2. The A – Y curve................................................................................................................................ 2 2.1. Change of material conductivity............................................................................................. 2 2.2. Change of frequency............................................................................................................... 3 2.3. Changes in lift off.................................................................................................................... 4 3. Impedance plane analysis............................................................................................................... 4 3.1. Improving separation.............................................................................................................. 5 3.2. Rotating probe impedance plane analysis.............................................................................. 6 3.3. Rotating probe – Fault location.............................................................................................. 7 Diagrams Diagram 1: Impedance vectors for probe in air and in contact with aluminium.................................... 2 Diagram 2: The A – Y Curve..................................................................................................................... 3 Diagram 3: Effect of increasing frequency.............................................................................................. 3 Diagram 4: Effect of changes in lift off.................................................................................................... 4 Diagram 5: Typical screen presentation................................................................................................. 5 Diagram 6: Separation through changing frequency.............................................................................. 5 Diagram 7: Screen representation of the effect of Y axis spread........................................................... 6 Diagram 8: The X – Y (Spot) display........................................................................................................ 7 Diagram 9: Typical Y – T (timebase) display............................................................................................ 7 Document Number: TESTIA - UK - TRA – ET – 003B - EN - Issue 2 Registered in England and Wales Testia Limited Company No. 08360678 Airbus Main Gate, Golf Course Lane, Building 07D, Filton, Bristol, UK BS34 7QQ Chapter 2 Page 2 of 7 1. Introduction Older analogue eddy current equipment such as the Hocking Locator, show indications using a simple needle display. This can be a major drawback as changes in conductivity, permeability, the mass effect, ferrous effect, geometry and all discontinuities show themselves as a needle deflection accompanied by an audible beep. It is left to the operator to determine whether he has discovered a fault or has some other reason for the indication. With Impedance Plane Analysis equipment some of these factors can be uniquely displayed and therefore determined by the operator. The impedance of a probe is seen by the eddy current equipment as being resistive and inductive. The various factors which affect Eddy currents, change the values of resistance and inductive reactance differently. By plotting the resistance and the inductive reactance of the probe on a diagram, known as the impedance plane, the movement of this point will yield more information about the test being carried out than a simple meter deflection would. In practice, a cathode ray tube (CRT) or liquid crystal display (LCD) is used to display this information. 2. The A – Y curve 2.1. Change of material conductivity Consider the impedance of an eddy current probe operating at a fixed frequency (Diagram 1). With the probe held in air the resistance and inductive reactance would draw the vector P0 whilst P1 is the vector of the impedance when the probe is in contact with a conductive material such as aluminium. If the probe is now held in contact with a range of non-ferrous metals and the resultant vectors plotted, a line can be drawn through the points (Diagram 2). This line is called the A-Y curve. Reactance P0 (Air) P1 (Aluminium) Resistance Diagram 1: Impedance vectors for probe in air and in contact with aluminium Document Number: TESTIA - UK - TRA – ET – 003B - EN - Issue 2 Registered in England and Wales Testia Limited Company No. 08360678 Airbus Main Gate, Golf Course Lane, Building 07D, Filton, Bristol, UK BS34 7QQ Chapter 2 Page 3 of 7 Reactance Air 0% IACS Titanium 3.3% IACS Aluminium Alloy 35% IACS Copper 100% IACS Resistance Diagram 2: The A – Y Curve 2.2. Change of frequency If the frequency that the probe is operating at is altered, the positions on the A-Y curve of the different conductivities move around the curve. However, as the operating frequency changes so would the size of the A-Y curve. To enable direct comparisons to be made the curves are normalised, that is the values of the impedances are divided by the value for the probe in air at that frequency. An increase in frequency moves all the points clockwise around the normalised curve, and also moves them closer together (Diagram 3). Air 0% IACS Normalised Increase in Inductive frequency Reactance Titanium 3.3% IACS Aluminium Alloy 35% IACS Copper 100% IACS Normalised Resistance Diagram 3: Effect of increasing frequency Document Number: TESTIA - UK - TRA – ET – 003B - EN - Issue 2 Registered in England and Wales Testia Limited Company No. 08360678 Airbus Main Gate, Golf Course Lane, Building 07D, Filton, Bristol, UK BS34 7QQ Chapter 2 Page 4 of 7 2.3. Changes in lift off If A-Y curves are plotted for a fixed frequency but with different values of lift off, a set of A-Y curves are produced (Diagram 4). Increasing lift off takes each value of conductivity on to another curve and towards the probe-in-air point. Air 0% IACS Normalised Titanium 3.3% IACS Inductive Reactance Aluminium Alloy 35% IACS Copper 100% IACS Normalised Resistance Diagram 4: Effect of changes in lift off 3. Impedance plane analysis Consider point x on a typical A-Y curve where the probe is in contact with a serviceable piece of aluminium alloy (Diagram 5). If the probe is moved over a crack, the eddy currents in the material reduce as if there was effectively a reduction in conductivity. If the probe is lifted off, the impedance value changes towards the probe in air point. It is the convention to rotate the image on the screen of an impedance plane instrument so that the lift off direction is horizontal. Also the serviceable impedance value is positioned in the centre. Now it is possible to differentiate between cracks and lift off by the direction of the spot movement on the screen. Edge effect moves the impedance value in a direction between crack and lift off. Mass effect moves it to the right in the opposite direction from lift off whilst ferrous effect moves the spot along a line just to the right of a tight fatigue crack. The example shown is for a tight fatigue crack, but wider cracks produce angles of deflection towards the lift off direction so therefore any large indication should be investigated further. Document Number: TESTIA - UK - TRA – ET – 003B - EN - Issue 2 Registered in England and Wales Testia Limited Company No. 08360678 Airbus Main Gate, Golf Course Lane, Building 07D, Filton, Bristol, UK BS34 7QQ Chapter 2 Page 5 of 7 Crack Ferrous Effect Air 0% IACS Edge Effect Normalised Inductive Mass Lift off Effect Reactance Lift off X Crack X Aluminium Alloy 35% IACS Normalised Resistance Diagram 5: Typical screen presentation 3.1. Improving separation The separation between crack and lift off indications will depend upon the position on the A-Y curve, of the impedance of the probe when in contact with good material. This point will depend on the frequency of operation (Diagram 6) which is selected to obtain the best fault signal separation while maintaining suitable depth of penetration to identify the required faults. Air 0% IACS LOWER FREQUENCY Crack REDUCED SEPARATION Lift off GREATER PENETRATION Normalised Lift off Crack Inductive Reactance HIGHER FREQUENCY GREATER SEPARATION REDUCED PENETRATION Normalised Resistance Diagram 6: Separation through changing frequency Document Number: TESTIA - UK - TRA – ET – 003B - EN - Issue 2 Registered in England and Wales Testia Limited Company No. 08360678 Airbus Main Gate, Golf Course Lane, Building 07D, Filton, Bristol, UK BS34 7QQ Chapter 2 Page 6 of 7 As the spot’s position on the screen is determined by being deflected horizontally (X direction) and vertically (Y direction), further separation can be achieved by having more amplification in the Y direction than in the X. This is referred to as Y-axis spread. Consider the improved indication when a crack indication has its angle from lift off increased (Diagram 7). Crack indication with Y axis Crack indication with X and Y receiving more amplification axis receiving the same gain than the X axis Lift off indication is set horizontally and is unaffected by increases in Y axis gain Diagram 7: Screen representation of the effect of Y axis spread 3.2. Rotating probe impedance plane analysis For rapid inspection of holes, it may be necessary to pass though several different layers and not have indications from the edges of each layer. The indications from this type of probe are displayed on Impedance Plane Analysis test equipment, albeit in a different manner to that already described. The change in impedance of the probe coils is displayed in the X and Y directions as before. However, as the coil arrangement is differential the spot is in the centre of the screen whenever the two parts of the probe are affected equally. If the probe passes over a fault, first one pickup coil is affected and the spot moves on the screen, then when the fault is equally between the coils the spot returns to the centre. As the second pickup coil passes over the spot moves in the opposite direction, finally returning to its original starting position as the coil moves away from the fault. As the probe is rotating in the hole at approximately 2,700 RPM a continuous image is shown on the screen of this double loop as the probe scans repeatedly over the fault. The difference in direction between tight fatigue cracks and mechanical damage is about 30 degrees. It is conventional to set fatigue cracks at vertical, with the mechanical damage direction at 30 degrees anticlockwise (Diagram 8). This display is called the X-Y or Spot display. No axis spread is used when carrying out rotating eddy current inspections. Rotating eddy current inspections on aluminium alloy are usually carried out at a frequency of 500 kHz. Document Number: TESTIA - UK - TRA – ET – 003B - EN - Issue 2 Registered in England and Wales Testia Limited Company No. 08360678 Airbus Main Gate, Golf Course Lane, Building 07D, Filton, Bristol, UK BS34 7QQ Chapter 2 Page 7 of 7 Mechanical damage Crack 30° Diagram 8: The X – Y (Spot) display 3.3. Rotating probe – Fault location In addition to the spot display, which is showing the impedance plane, there is another display mode provided which plots the vertical component of the spot display against the probe’s angular position in the hole. This is called the Y-T or Timebase display. To achieve this, the spot is deflected across the screen left to right and synchronised with the rotation of the probe, so that one sweep across the screen represents one revolution, 360°. If the probe gun is held so that the switch box (taken as the reference) aligns with the fault, the indication will appear in the centre of the screen, any indication whose position in the hole is displaced by an angle from the gun’s reference will appear on the timebase at a position proportional to this angle (Diagram 9). Fault A crack Fault B Mechanical damage Fault A crack Fault B 180° 90° 0° 90° 180° Mechanical damage Anticlockwise Clockwise Diagram 9: Typical Y – T (timebase) display Document Number: TESTIA - UK - TRA – ET – 003B - EN - Issue 2 Registered in England and Wales Testia Limited Company No. 08360678 Airbus Main Gate, Golf Course Lane, Building 07D, Filton, Bristol, UK BS34 7QQ Chapter 3 Page 1 of 12 EDDY CURRENT TESTING CHAPTER 3 COILS AND PROBES CONTENTS Paragraphs 1. Introduction.................................................................................................................................... 3 2. Absolute coils.................................................................................................................................. 3 2.1. Single absolute coil................................................................................................................. 4 2.2. Double absolute coil............................................................................................................... 4 3. Differential coils.............................................................................................................................. 5 3.1. Single differential self comparative coils................................................................................ 5 3.2. Double differential self comparative coils.............................................................................. 6 3.3. Double differential external comparative coils...................................................................... 6 4. Hand-held probes........................................................................................................................... 7 4.1. Probes for special purposes.................................................................................................... 8 4.2. Probe coils............................................................................................................................... 9 4.3. Probe cores............................................................................................................................. 9 4.4. Shielded probes...................................................................................................................... 9 4.5. Differential probes................................................................................................................ 10 4.6. Rotating probes..................................................................................................................... 11 5. Probe care..................................................................................................................................... 12 Document Number: TESTIA - UK - TRA - ET – 003C - EN - Issue 3 Registered in England and Wales Testia Limited Company No. 08360678 Airbus Main Gate, Golf Course Lane, Building 07D, Filton, Bristol, UK BS34 7QQ Chapter 3 Page 2 of 12 Diagrams Diagram 1: An encircling coil................................................................................................................... 3 Diagram 2: Single absolute coil arrangement......................................................................................... 4 Diagram 3: Double Absolute Coil............................................................................................................ 4 Diagram 4: Single differential self comparative coil arrangement......................................................... 5 Diagram 5: Double differential self comparative coil arrangement....................................................... 6 Diagram 6: Double differential external comparative coil arrangement............................................... 7 Diagram 7: Common eddy current probes............................................................................................. 8 Diagram 8: An example of special probes............................................................................................... 8 Diagram 9: Air cored and Ferrite cored coils.......................................................................................... 9 Diagram 10: Cross section through shielded probe.............................................................................. 10 Diagram 11: Variable frequency differential probe coil arrangement................................................. 10 Diagram 12: Rotating probe coil arrangement..................................................................................... 11 Document Number: TESTIA - UK - TRA - ET – 003C - EN - Issue 3 Registered in England and Wales Testia Limited Company No. 08360678 Airbus Main Gate, Golf Course Lane, Building 07D, Filton, Bristol, UK BS34 7QQ Chapter 3 Page 3 of 12 1. Introduction There are many different types of probes, each designed to be used for a specific purpose. To help understand why we use certain probes for certain tasks, we need to understand the coil arrangements within those probes. To explain the operation of the different types of coil arrangements, it is easier to consider encircling coils. Encircling coils (Diagram 1) are wound so that components, usually items such as wire, tubes, and cylinders of uniform cross section, can be inspected by passing them through the centre of the coil. Their principle of operation can then be read across to the hand-help probes with which we are more familiar. Diagram 1: An encircling coil 2. Absolute coils Absolute coils can be used for flaw detection, conductivity measurements, lift-off measurements and thickness measurements. They are widely used due to their versatility. Since absolute probes are sensitive to things such as conductivity, permeability lift-off and temperature, steps must be taken to minimize these variables when they are not important to the inspection being performed. Document Number: TESTIA - UK - TRA - ET – 003C - EN - Issue 3 Registered in England and Wales Testia Limited Company No. 08360678 Airbus Main Gate, Golf Course Lane, Building 07D, Filton, Bristol, UK BS34 7QQ Chapter 3 Page 4 of 12 2.1. Single absolute coil With the single absolute coil, only one coil or winding is used (Diagram 2). The eddy currents induced into the component affect the impedance of the coil. Any changes in impedance are detected and displayed on the test set. The change in impedance is an absolute measurement, given without comparison to any references. Single absolute coils can be small, cheap to produce and fairly sensitive in the hands of an expert but lack the ability to be truly optimised for smaller faults. COMPONENT PASSING THROUGH ENCIRCLING COIL Diagram 2: Single absolute coil arrangement 2.2. Double absolute coil Here, two coils are used (Diagram 3). A primary driver coil which induces the eddy currents in the component under test, and a secondary pickup coil which gives an output dependent on the resulting eddy currents in the component. These are sometimes known as Reflection probes or driver/pickup probes. Again the output is an absolute value that is not compared with any reference. Diagram 3: Double Absolute Coil The advantage of reflection probes is that the driver and pickup coils can be separately optimized for their intended purpose. The driver coil can be made so as to produce a strong and uniform flux field in the vicinity of the pickup coil, while the pickup coil can be made very small so that it will be sensitive to very small defects. They are however more expensive and require a more complicated set up. Document Number: TESTIA - UK - TRA - ET – 003C - EN - Issue 3 Registered in England and Wales Testia Limited Company No. 08360678 Airbus Main Gate, Golf Course Lane, Building 07D, Filton, Bristol, UK BS34 7QQ Chapter 3 Page 5 of 12 3. Differential coils Differential coil arrangements can be divided into two types, single differential and double differential. These can be further sub-divided into self-comparative and external comparative types. 3.1. Single differential self-comparative coils Here, a coil is wound in two parts to cover two different areas of the component (Diagram 4). The two halves of the coil are wound in opposition. The impedances of the two halves of the coil are compared to each other, and on a serviceable component would cancel each other out giving a no- fault indication. Faults are detected by one winding being over a defective area of the component and one winding being over a good area. If a discontinuity was present a difference of impedance between the two coils halves would exist and this would be displayed by the equipment. Impedance of coil Compared with impedance over this area of coil over this area Diagram 4: Single differential self-comparative coil arrangement Document Number: TESTIA - UK - TRA - ET – 003C - EN - Issue 3 Registered in England and Wales Testia Limited Company No. 08360678 Airbus Main Gate, Golf Course Lane, Building 07D, Filton, Bristol, UK BS34 7QQ Chapter 3 Page 6 of 12 3.2. Double differential self-comparative coils Here a single driver coil, covering both areas to be compared, is wound over a pick-up coil (Diagram 5). One half of the pick-up coil is wound in the same direction as the driver, the other half of the pick-up coil is wound in the opposite direction to the driver. If both areas of the component are identical then the outputs from each half of the pick-up coil will be equal in amplitude, but opposite in phase and will cancel each other out. Only if the areas being inspected are not identical will a difference in impedance be detected resulting in an indication. Driver coil Impedance of coil Pick up coil Compared with impedance over this area of coil over this area Diagram 5: Double differential self comparative coil arrangement 3.3. Double differential external comparative coils A double differential external comparative coil arrangement compares the part of the component being inspected with part of a reference component which is known to be serviceable (Diagram 6). The method by which changes of impedance are compared and displayed are identical to the explanation given at Para 3.2. Document Number: TESTIA - UK - TRA - ET – 003C - EN - Issue 3 Registered in England and Wales Testia Limited Company No. 08360678 Airbus Main Gate, Golf Course Lane, Building 07D, Filton, Bristol, UK BS34 7QQ Chapter 3 Page 7 of 12 Component Pickup coil Driver coil Comparator Diagram 6: Double differential external comparative coil arrangement All differential probes have the advantage of being very sensitive to defects yet relatively insensitive to slowly varying properties such as gradual dimensional or temperature variations. Probe wobble signals are also reduced with this probe type. However, the signals may be difficult to interpret i.e. if a flaw is longer than the spacing between the two coils, only the leading and trailing edges will be detected due to signal cancellation when both coils sense the flaw equally. 4. Hand-held probes Most aerospace eddy current inspections are carried out using hand-held probes. These probes usually have a single absolute coil mounted in the tip of the probe, the body of which forms the handle. The probe is shaped to give comfortable handling and can be supplied with different tip/coil arrangements, handle lengths and geometries to form a range of probes which should give complete and easy access to various parts of the component under test. The most common are the spade, pencil and bolthole probes (Diagram 7). Document Number: TESTIA - UK - TRA - ET – 003C - EN - Issue 3 Registered in England and Wales Testia Limited Company No. 08360678 Airbus Main Gate, Golf Course Lane, Building 07D, Filton, Bristol, UK BS34 7QQ Chapter 3 Page 8 of 12 COIL AXIS SPADE PROBE COIL AXIS PENCIL PROBE SPLIT END TO ADJUSTABLE ENSURE GOOD COLLAR TO SET CONTACT SCAN DEPTH COIL AXIS BOLTHOLE PROBE Diagram 7: Common eddy current probes 4.1. Probes for special purposes Where problems regarding the shape of the item being inspected or access to the scan area occur, special bespoke probes can be manufactured to suit (Diagram 8). Diagram 8: An example of special probes Document Number: TESTIA - UK - TRA - ET – 003C - EN - Issue 3 Registered in England and Wales Testia Limited Company No. 08360678 Airbus Main Gate, Golf Course Lane, Building 07D, Filton, Bristol, UK BS34 7QQ Chapter 3 Page 9 of 12 4.2. Probe coils The type of coil used in a probe has a significant effect on the sensitivity of an eddy current test. In general, to obtain high sensitivity, a small eddy current field is required because a small discontinuity will have a greater effect on a small field than on a large one. Therefore, for high sensitivity, small coils are used. 4.3. Probe cores The core of a probe coil may be air or a material, such as plastic which has magnetic and electrical properties similar to air, they are called air cored coils. To concentrate the magnetic field of a coil, and hence make it more sensitive to small discontinuities, a ferrite core can be used. Ferrite is a ceramic ferro-magnetic compound which has low electrical conductivity and high permeability (Diagram 9). Diagram 9: Air cored and Ferrite cored coils 4.4. Shielded probes Shielded probes are constructed with a ferrite core and shield (Diagram 10). The purpose of the ferrite shield is to provide a low reluctance path for the flux, which prevents the field spreading. This helps to avoid ferrous effect when scanning near fasteners and, mass and edge effect when scanning in difficult geometries. Document Number: TESTIA - UK - TRA - ET – 003C - EN - Issue 3 Registered in England and Wales Testia Limited Company No. 08360678 Airbus Main Gate, Golf Course Lane, Building 07D, Filton, Bristol, UK BS34 7QQ Chapter 3 Page 10 of 12 Ferrite shield Ferrite shield Ferrite core Magnetic lines or flux Diagram 10: Cross section through shielded probe 4.5. Differential probes Consider a differential probe of the type used with variable frequency equipment (Diagram 11). This arrangement gives zero output from the pickup coil when held in air; this improves its sensitivity when in contact with the material under inspection. A variation on this arrangement has a transparent Perspex core referred to as an air core. This is known as a ring probe or doughnut probe. It is used for detecting cracks from holes that have their fasteners fitted. The transparent core allows centering over the fastener. Driver coil Pickup coil Diagram 11: Variable frequency differential probe coil arrangement Document Number: TESTIA - UK - TRA - ET – 003C - EN - Issue 3 Registered in England and Wales Testia Limited Company No. 08360678 Airbus Main Gate, Golf Course Lane, Building 07D, Filton, Bristol, UK BS34 7QQ Chapter 3 Page 11 of 12 4.6. Rotating probes For the rapid inspection of the bores of holes, rotating probes are used. These probes are held in a special gun which rotates the probe at approximately 2,700 RPM. The probe is pushed slowly through the hole and so gives a tight helical scan path. To enable the probe to scan right up to the ends of the bore and to pass from one layer to another e.g., skin to spar, the probe is only sensitive to discontinuities that are orientated in the direction of the hole axis. The probe is a double differential self-comparative coil arrangement. The two halves of the pickup coil are aligned so that as the probe rotates, they both pass over the same scan path with one leading the other. Any discontinuity which is in line with the direction of travel of the coil, such as the edge at the end of a bore, will affect both halves simultaneously and produce no output. Any discontinuity running in the direction of the hole axis (Diagram 12), will first change the impedance of one half of the coil giving a differential output, then the discontinuity will pass under the trailing half of the coil and give a second differential output. Diagram 12: Rotating probe coil arrangement Document Number: TESTIA - UK - TRA - ET – 003C - EN - Issue 3 Registered in England and Wales Testia Limited Company No. 08360678 Airbus Main Gate, Golf Course Lane, Building 07D, Filton, Bristol, UK BS34 7QQ Chapter 3 Page 12 of 12 5. Probe care Although the surface probe is normally manufactured with a wear resistant tip, it is subject to wear as it is rubbed over the surface of the component to be inspected, especially if the surface is rough which also makes smooth scanning difficult. The probe tip may also scratch the surface of the item under test so to reduce wear and damage, extend the life of the probe, ease smooth probe movement and to keep a uniform ‘lift off’ distance between the probe and the items surface, we put a layer of PTFE tape over the tip. This tape must be checked and replaced regularly to ensure is does not wear through or get thin which would change the lift off settings and allow scratching damage to occur. Every time the tape is replaced, the ‘lift off’ settings should be checked. PTFE tape is not used on rotary probes. Document Number: TESTIA - UK - TRA - ET – 003C - EN - Issue 3 Registered in England and Wales Testia Limited Company No. 08360678 Airbus Main Gate, Golf Course Lane, Building 07D, Filton, Bristol, UK BS34 7QQ Chapter 4 Page 1 of 16 EDDY CURRENT TESTING CHAPTER 4 ELECTRICAL THEORY CONTENTS Paragraphs 1. Introduction and Magnetic Theory................................................................................................. 3 1.1. Current.................................................................................................................................... 3 1.2. Resistance............................................................................................................................... 3 1.3. Voltage.................................................................................................................................... 3 1.4. Ohm’s Law............................................................................................................................... 4 1.5. Power...................................................................................................................................... 4 1.6. Alternating Current (AC)......................................................................................................... 4 1.7. Frequency................................................................................................................................ 5 2. Measurement of AC values............................................................................................................. 5 3. Rectification.................................................................................................................................... 5 4. Resistors in series............................................................................................................................ 6 5. Resistors in parallel......................................................................................................................... 6 6. The potential divider....................................................................................................................... 6 7. The Wheatstone Bridge.................................................................................................................. 7 8. Alternating Current Theory............................................................................................................. 8 8.1. The Sine Wave......................................................................................................................... 8 9. Resistance and reactance............................................................................................................. 10 9.1. Resistance............................................................................................................................. 10 9.2. Reactance.............................................................................................................................. 11 9.2.1. Inductive Reactance...................................................................................................... 11 9.2.2. Capacitive Reactance.................................................................................................... 12 9.3. Circuits containing resistance and reactance....................................................................... 13 10. Impedance................................................................................................................................ 14 10.1. Circuits containing resistance and inductance................................................................. 14 10.2. Circuits containing resistance, and capacitive and inductive reactance.......................... 14 11. Resonance................................................................................................................................. 15 Document Number: TESTIA - UK - TRA - ET – 003D - EN - Issue 3 Registered in England and Wales Testia Limited Company No. 08360678 Airbus Main Gate, Golf Course Lane, Building 07D, Filton, Bristol, UK BS34 7QQ Chapter 4 Page 2 of 16 Diagrams Diagram 1: A simple electrical circuit...................................................................................................... 3 Diagram 2: Typical alternating current waveform.................................................................................. 4 Diagram 3: Half and full-wave rectification............................................................................................ 5 Diagram 4: Resistors in series................................................................................................................. 6 Diagram 5: Resistors in parallel.............................................................................................................. 6 Diagram 6: The Potential Divider............................................................................................................ 7 Diagram 7: The Wheastone Bridge......................................................................................................... 7 Diagram 8: Phasor representation of a sine wave.................................................................................. 8 Diagram 9: Angular representation of a sine wave................................................................................ 9 Diagram 10: A Vector diagram....................................