Pathophysiology of Peripheral Blood Circulation PDF
Document Details
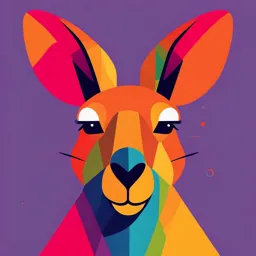
Uploaded by StrongerLogic195
Krishtal
Tags
Related
- Treatment and Diagnosis of Chemotherapy-Induced Peripheral Neuropathy (CIPN) PDF
- Treatment and Diagnosis of Chemotherapy-Induced Peripheral Neuropathy PDF
- Vascular Disorders Module 3C PDF
- Module 3C - Vascular Disorders PDF
- Pathomedicine Module 3.3 Blood Pressure Regulation PDF
- Peripheral Neuropathies BMS200 PDF
Summary
This document provides a detailed overview of the pathophysiology of peripheral blood circulation. It goes into the anatomical and functional classifications of vessels, mechanisms regulating vessel tone, and different types of hyperemia. It is a theoretical study of the topic and is for educational use.
Full Transcript
TYPICAL PATHOLOGICAL PROCESSES Chapter XIV PATHOPHYSIOLOGY OF PERIPHERAL BLOOD CIRCULATION Circulation at the site of the peripheral vascular bed includes the following elements: smal...
TYPICAL PATHOLOGICAL PROCESSES Chapter XIV PATHOPHYSIOLOGY OF PERIPHERAL BLOOD CIRCULATION Circulation at the site of the peripheral vascular bed includes the following elements: small arteries, arterioles, metarterioles, precapillary latching muscles, capillaries, postcapillary latching muscles, arteriolovenular anastomoses, venules, and small veins. Conceptionally, the peripheral circulation system is taken to have the microcirculatory bed consisting of terminal vessels, their diameter being under 100 microns: arterioles, metarterioles, capillaries, venules, arteriolovenular anastomoses. A separate group is formed by the vessels of the lymphatic system. However, in physiology and pathophysiology more commonly is used the functional classification of the vascular microcirculation bed that includes the three main groups of vessels: resistive, exchange, and capacitive. Compliance with the anatomical and functional classification is given in Table 8. Table 8. Anatomical and functional classification of the peripheral vessel bed Anatomical classification Functional classification Arterioles Precapillars Metarterioles Resistive vessels Precapillar latching muscles Capillars Exchange vessels Postcapillaries Postcapillary latching muscles Venules Capacitive vessels Small veins Arteriolovenular anastomoses Lacteal vessels Lymphatic vascular system Resorptive vessels According to the functional classification, the first vascular group primarily provides peripheral resistance, the capillaries have the function of exchange, and the third group of vessels is in charge of blood deposition and venous return regulation. The vessels of the lymphatic system possess the function of resorption – collection of the tissue fluid and its return into the bloodstream. The peripheral resistance depends on the resistive vessels and plays a key role in regulating both the systemic blood pressure and the blood flow in the microvasculature. A systemic change in the tone of the peripheral vessels often causes changes in the systemic blood pressure (a spasm effects a pressure increase and dilatation results in a pressure reduction), whereas a local tone change (in a certain organ or a tissue area) leads to disruption of the blood flow at the microcirculation level (Table 9). Table 9. Development of pathological states due to changes in the tone of the resistive vessels State of resistive Systemic reaction Local reaction vessels Vasoconstriction Hypertension Ischemia Vasodilatation Hypotension Arterial hyperemia The mechanisms regulating the regional circulation include, on the one hand, the effect of vasoconstrictor and vasodilatory innervations, on the other hand – the effect of nonspecific metabolites, inorganic ions, local biologically active substances (BAS) and hormones that come with the blood on the vascular wall. It is believed that with a decrease in the diameter of blood vessels the neural regulation values decrease and these of the metabolic regulation, on the contrary, increase. Various regulatory factors affect the tone of the resistive vessels (Table 10). Table 10. The mechanisms regulating the tone of the resistive vessels Vasoconstrictors Vasodilators The components of the vessel The contracting mechanism wall of non-striped muscle cells Neurogenic regulation Sympathetic Sympathetic vasodilators vasoconstrictors (the mediator: adrenalin – (the mediators: adrenalin, action upon (3- nor- adrenalin – action adrenoreceptors); upon a- adrenoreceptors) parasympathetic vasodilators (the mediator: acetylcholine) Humoral regulation via BAS Catecholamines; Kinins; histamine; and hormones angiotensin II; vasopressin; prostaglandins; endothelin; certain leukotrienes leukotrienes Humoral regulation by vaso- Carbon dioxide; local active products of tissue me- hypoxia; lactic acid; tabolism potassium and hydrogen ions; ATP (adenosine triphosphate) hydrolysis products, especially ade- nosine; NO (nitric oxide) The exchange blood vessels (capillaries) provide, in addition to the exchange of blood, exchange of water, electrolytes, gases, essential nutrients and metabolites through the system blood – tissue – blood. Morphologically, the permeability of capillary vessels and venules is based on the endothelium and the basal membrane. Differences in the intensity and selective permeability of the capillaries and venules of different vascular areas are due to the peculiarities of their structure (the thicknesses of the endothelial cells and basal membrane, the presence or absence of ion and water channels, glucose and other transporters, pinocytic paths, pores and inter-endothelial gaps), and the functional state of these structures. The mechanism of transporting substances through the vascular wall can be active or passive. It is active in case it is operating through the plasma membranes of the endothelial cells against a concentration or electrochemical gradient (“upward” transport), which requires specific carriers or microvesicles whose work needs a certain amount of energy. The transport is called passive, if it is going on under an osmotic, concentration or electrochemical gradient without energy spent. It serves mainly for carrying water, dissolved gases and low-molecular substances, i.e. those substances that can penetrate through the walls of the exchange blood vessels directly or in presence of specific protein channels. The passive mechanism of transporting substances through the walls of microvessels can be classified into two types: ultrafiltration and diffusion. The process of fluid transition through the capillary membrane is termed as “filtering’, and that of the exchange of substances and gases dissolved in water is termed as “diffusion”. The transport of fat-soluble substances (oxygen, carbon dioxide, alcohol) through the lipid layer of cell membranes is termed as “simple diffusion”. The penetration of water and water-soluble substances can take place either through the intercellular spaces between the endotheliocytes that make 1/1000 of the total surface area of capillaries, or through specific protein channels embedded in the lipid layer of cell membranes (in the absence of these channels, the membranes are impermeable to water). The latter form of transport is called “facilitated diffusion" or “facilitatedfiltering" (see Chapters VII “Pathophysiology of Carbohydrate Metabolism”, XI “Pathophysiology of Water-Electrolyte Balance”, XVI “Inflammation”, XXX “Pathophysiology of the Kidneys”). Thus, the exchange function of capillaries is provided by a combination of three processes: two of them are passive – filtration and diffusion, and one is active – transport of substances through special conveyors or microvesicles. With the development of functional, structural or pathological changes in the organs or tissues, local circulatory disorders may appear, the most common forms of which include hyperemia – growth of blood supply in the organs and tissues, which is classified into arterial and venous, and ischemia, stasis, thrombosis and embolism. ARTERIAL HYPEREMIA Arterial hyperemia is growth of blood supply as a Bl result of excessive blood flow in the arterial vessels. oo An increased blood flow in the d pr microvasculature is caused by local expansion of the ess resistive vessels; therefore arterial hyperemia is often ur e, called active. Its development is characterized by a kP number of functional changes and clinical a Arteries Arterioles Capillaries Veins manifestations. Fig. 22. Blood pressure dynamics in different parts The main features include diffused redness, of the bloodstream and at arterial hyperemia expansion of small arteries, arterioles and capillaries, ripple of small arteries and capillaries, increase of the number of functional capillaries, local temperature rise, increase of the volume of hyperemic areas, increased tissue turgor, increased pressure in the arterioles, capillaries and veins (Fig. 22), acceleration of the blood circulation, increased metabolism and enhanced functioning of the organ. Arterial hyperemia may be caused by various environmental factors, including biological, physical, chemical, as well as by increased pressure on the organ or tissue section and psychogenic effects. Since a certain portion of these factors consists of common physiological stimuli (increased load on the body, psychogenic effects, dilatation of arterioles in the skin as a component of thermoregulation), the arterial hyperemia that occurs under their influence should be considered physiological. The main varieties of physiological arterial hyperemia include the working (or functional) and reactive hyperemia. Working hyperemia is the blood flow acceleration in the organ, which results from enhancement of the organ’s functions (the pancreas functioning during digestion, constriction of a skeletal muscle, the coronary blood flow increase at intensive work of the heart, the blood inflow to the brain due to mental stress). Reactive hyperemia occurs after a short restriction of the blood flow. Usually it develops in the kidneys, brain, skin, intestines and muscles. The maximum response is observed within a few seconds after the restoration of perfusion. Its duration is determined by the duration of occlusion. The reactive hyperemia eliminates the blood flow “debt” that occurred during occlusion. Pathological arterial hyperemia develops under unusual (pathological) stimuli (chemicals, toxins, mechanical factors, products of disturbed metabolic exchange, mediators that appear during inflammation, bums and some other states). Facial flushing in many infectious diseases (measles, typhus, scarlet fever), vasomotor disturbances in case of systemic lupus erythematosus, redness of the skin of the limbs due to damage of certain nerve plexuses, redness of a half of the face due to neuralgia caused by irritation of the trigeminal nerve are the clinical signs of pathological arterial hyperemia. Depending on the factors causing it, inflammatory hyperemia, heat hyperemia, ultraviolet erythema etc. can be distinguished. As for pathogenesis, two kinds of the pathological arterial hyperemia are distinguished – neurogenic (the neurotonic type and the neuroparalytic type) and caused by the action of local BAS and metabolic factors. Neurogenic arterial hyperemia develops due to changes in the tone of different divisions of the autonomic nervous system. Activation of the sympathetic nervous system is known to be accompanied by a spasm of the resistive vessels of the skin (involving a- blockers), while activation of the parasympathetic department is accompanied by expansion of these vessels. In this regard, for hyperemia to emerge, there must be an increase of the tone of the parasympathetic division of the nervous system (neurotonic hyperemia) or a reduction of the tone of the sympathetic division of it (neuroparalytic hyperemia). Arterial hyperemia of the neurotonic type can develop reflexly due to stimulation of exteroreceptors and interoreceptors, and also of vasodilatory nerves and centers. The stimuli can be mental, mechanical, thermal (heat effect), chemical (turpentine, mustard oil, etc.) and biological. A typical example of neurogenic arterial hyperemia is redness of the face and neck at pathological processes in the internal organs (ovaries, heart, liver, lungs). Claude Bernard first reproduced neurogenic arterial hyperemia by irritating chorda tympani – the branch of n. facialis, which consists of parasympathetic vasodilatory fibers, in experiments with animals (rabbits and dogs). In response to stimulation, hyperemia and increased secretion of the submandibular salivary gland occurred. In some cases, neurotonic hyperemia can be caused by activation of the sympathetic nervous system (cholinergic, histaminergic, and p-adrenergic) presented at the periphery by the respective fibers, neurotransmitters and receptors (H2-receptors of histamine, p2-adrenergic receptors of adrenaline, muscarinic receptors of acetylcholine). The sympathetic cholinergic nerves expand small arteries and arterioles of the skeletal muscles, muscles of the face, cheek mucosa, intestines. They too have acetylcholine as a mediator. Arterial hyperemia of the neuroparalytic type can be seen in the clinic and animal ex- periments with cutting ά-adrenergic sympathetic fibers. In 1842, Alexander Walter first noticed vasodilatation in a frog’s leg after cutting the sympathetic fibers of the sciatic nerve. Later (1851) Claude Bernard observed redness and temperature rise of a rabbit’s scalp on the side where the cervical sympathetic trunk node was removed. This is especially expressed in the area of the ear (Fig. 23). Similar effects are also seen at violation of the integrity of mixed nerves, which include ά- adrenergic vasoconstrictor fibers. The sympathetic vasoconstriction nerves are tonicly active, and under normal circumstances they are constantly carrying pulses of central origin (1–3 pulses per second at rest), which indicates that there is a neurogenic (vasomotor) component of the vascular tone. These nerves’ mediator is noradrenaline. In humans and animals, tonic impulsation is characteristic of the sympathetic nerves leading to the blood vessels of the skin of the upper limbs, ears, skeletal muscles, digestive tract etc. Cutting these nerves in each of these organs causes an increase of the blood flow in the arteries. This effect is a basis for applying periarterial and ganglionic sympath- ectomy at endarteritis (which is accompanied by prolonged vasospasms). Neuroparalytic type hyperemia can be caused by Fig. 23. The experiment of C. Bernard chemically blocking the transmission of nerve impulses in (1851): removal of the cervical sympathetic trunk node on the right the area of the sympathetic nodes (using ganglioplegics) or, side at the level of the sympathetic nerve endings, via sympatholytic drugs or ά-adrenoblockers. Under these circumstances, the potential-dependent slow calcium channels are blocked, the delivery of extracellular Ca2+ under an electrochemical gradient to the smooth muscles and Ca2+ release from the sarcoplasmic grid are disrupted. As a result, the cells of the smooth muscle tissue relax. The neuroparalytic mechanism of arterial hyperemia is partly a basis for inflammatory hyperemia, ultraviolet erythema etc. The concept of arterial hyperemia (physiological and pathological) caused by local metabolic (chemical) factors and BAS, formed on site or coming from the blood, is based on the fact that these factors cause vasodilation by acting directly on the elements of smooth muscles and their walls, regardless of innervation. This notion is also confirmed by the fact that complete denervation does not prevent either working or reactive, or inflammatory arterial hyperemia. Expansion of vessels results from a decrease of the oxygen pressure (pO2) in the blood and the vessel wall tissue. The mechanism of the vasodilating influence of hypoxia is that a decrease of pO2 causes a decrease in the production of ATP. This leads to opening of the ATP-dependent potassium channels in the membranes of smooth- muscle cells. The release of potassium from the cells causes hyperpolarization of the cell membranes, which closes electrically-controlled calcium channels. As a result, the level of free calcium in the cytosol decreases, which causes relaxation of smooth-muscle tissues and vasodilation. Arterial hyperemia also occurs due to an increase of pCO2, excess of non-specific metabolites and inorganic ions (lactic acid, organic acids of the Krebs cycle, ADP, AMP, adenosine, potassium ions), local BAS (bradykinin, kalidyn, histamine, PGE, PGA, PHI 2, non- mediator acetylcholine, substance P, GABA) and hormones that come to the organs with blood (PNP, adrenomedulin, vasoactive intestinal peptide – VIP). The significance of each of these factors in the development of arterial hyperemia of different origins varies. In the event of reactive and working arterial hyperemia as a consequence of hypoxia, particularly important is adenosine formed as a result of ecto-5'-nucleotidase action on AMP. The source of adenosine is smooth-muscle and endothelial vascular cells, cells of the parenchyma of the organ, from which some adenosine is transported to the extracellular space, causing vasodilatation. Adenosine deaminase ensures destruction of adenosine, thus maintaining its balance inside the cell and outside it. Adenosine, histamine and PNP affect smooth-muscle cells via H2 receptors. Another physiologically important, highly active and versatile factor of vasorelaxation is NO that forms in the cells intact endothelium with L arginine in presence of NADPH and oxygen. The synthesis of nitric oxide can occur in several ways. In humans, this process is regulated by NO- synthases (NOS). NOS-enzymes are members of the hemo-containing enzyme superfamily known as monooxygenases. Depending on the structure and functions of NOS, they can be divided into three groups: endothelial (eNOS), neuronal (nNOS), and inducible (iNOS). Although all NOS isoforms catalyze the formation of NO, they are products of different genes; each has certain characteristics in the mechanisms of action and localizations, as well as in the biological significance to the organism. Thus, these isoforms are considered as divided into constitutive (ingredient) NOS (cNOS) and iNOS. The difference is that the constitutive enzyme is synthesized continuously, and the inducible (adaptive) form – only among the relevant factors. It has been established that NO is a labile molecule that lives a short time (a few seconds). In interaction with oxygen and water, NO is rapidly converted to nitrates and nitrites. Small vessels synthesize it in a larger quantity than large ones do. cNOS reacts with increased production and release of NO within minutes in response to the increase of Ca2+ level in endotheliocytes under effect of vasodilators (acetylcholine, bradykinin, kallidin, adrenomedullin, substance P, VIP), hypoxia, estrogens and increased shear blood stress relative to the endothelial cells. Diffusing from the endothelial cells to smooth-muscle ones, NO stimulates the guanylate cyclase in them with formation of cGMP, which activates cGMP-dependent protein kinase and calcium ATPases. As a result, in the smooth-muscle cells the Ca2+concentration reduces, these cells have their relaxation and vasodilatation. Furthermore, this effect is caused by the depressive influence of NO on the calcium sensitivity of contractile proteins. Under pathological conditions (bums, trauma, or harmful effects of ultraviolet radiation, the impact of infectious pathogens, leading to development of inflammation – septic or aseptic – with involvement of the immune mechanisms of damage or without it), an important role in the development of arterial hyperemia is played by iNOS. Its synthesis is carried out by expression of the corresponding gene (located in chromosome 7), under the influence of pro-inflammatory and immunogenic factors (endotoxins, interferons, IL 1, TNF α etc.). In this situation, the appropriate cells (endothelial cells, neutrophils, fibroblasts, but primarily monocytes and macrophages) are producing and secreting large amounts of NO for long hours. Here, an excess of NO creates not only a strong and prolonged arterial congestion but also cytolysis of the cells. An important role in strengthening the blood flow at local vascular reactions is played by changes of pH tissues: a shift of the reaction in the relevant zone toward acidosis promotes vasodilatation due to increased sensitivity of the endotheliocytes to vasodilatation factors and that of the smooth-muscle cells – to NO. At arterial hyperemia, the processes of filtration and diffusion in the microvasculature are changing. With the blood flow increase, the hydrostatic pressure level is increasing in both the arterial and venous sections of the capillaries, its increase by 10 mm Hg increases the filtration pressure in the arterial section up to 20 mm Hg, hence the fluid moves out of the vessels into the tissues more actively. In this situation, in the venous section the hydrostatic and oncotic pressures get equalized and the fluid resorption does not occur; however, a significant fluid accumulation in the tissues is not observed. This is due to a more intense return of fluid into the lymphatic system whose resorptive activity is significantly increased. As a result, the drainage of tissues is enhancing, the intensity of transport of nutrients to them and excretion of metabolites is increasing. Along with the increase of drainage of tissues, the diffusion processes become activated. At arterial hyperemia, the inflow of oxygen-saturated arterial blood to tissues increases, which significantly improves their oxygenation. However, the rate of linear and volumetric blood flow can be increased so that the tissue will fail to utilize all the oxygen, then oxygenation of the venous blood takes place (pO2 can reach 60 to 70 mm Hg). Here, the carbon dioxide is completely derived from tissues, which can be considered as a positive effect of arterial hyperemia; the microvesicular transport is not significantly changed. The arterial hyperemia can effect both positive (adaptive) and negative (pathological) changes; the positive effects prevail, being associated with enhancement of metabolism and body functions. However, there may be adverse effects too. For example, at atherosclerosis, a dramatic expansion of the vessel may be accompanied by rupture of its wall and tissue hemorrhage. Such situations are especially dangerous in the brain. VENOUS HYPEREMIA Venous hyperemia is the result of increasing the blood supply to the organ or tissue area due to complicated outflow of blood through veins. Violation of the outflow of the blood microcirculation causes stagnation of blood, thus venous hyperemia is also called passive hyperemia. It can be caused by clogging the vein with a thrombus or embolus, compression due to a tumor, scars, enlarged uterus. Thin-walled veins can also be compressed if swelling of tissues develops. In certain cases, the factor that leads to venous hyperemia is a constitutional weakness of the elastic apparatus of veins. Such a tendency often has a family character. Professions requiring long (for hours) daily staying upright imply risk factors that contribute to the development of venous hyperemia in the distal areas of the lower extremities in patients with constitutionally conditioned deficiency of the elastic and smooth-muscle elements in the vein walls. Also, venous hyperemia develops if there is weakening of the right ventricle function of the heart and reduction of the suction action of the chest (pleural effusion, hemothorax). In this pathology, stagnation of blood develops in the venous system of the greater circulation, and here systemic venous hyperemia can be considered. Slowdown of the blood flow in the pulmonary circulation at fibrosis and emphysema, weakening of the left ventricle function leads to stagnation of blood in the veins of the pulmonary circulation. Venous hyperemia is clinically manifested by an enlargement of the organ or tissue site, cyanosis, a decrease of the local temperature, swelling, increased pressure in the veins and capillaries of the stagnant area, slowdown of the blood flow, diapedesis of erythrocytes. At the final stage of hyperemia, pendulous motion of the blood and the blood stasis can be observed. Prolonged venous hyperemia causes pronounced changes in the functional elements of the vascular wall, their atrophy and death. Also, in the venous hyperemia area substitutional proliferation of the connective tissue is observed. A classic example is the liver cirrhosis caused by venous hyperemia, when the cardiac function is insufficient. At venous hyperemia, fluid filtration and drainage of tissues worsen substantially. Violation of the outflow from the microcirculation is accompanied by increased hydrostatic pressure in the venous capillary section, and its increase just by 10 mm Hg leads to equalization of the hydrostatic pressure and the oncotic one in this section. In the arterial area, the fluid continues to be filtered in the tissue, and in the venous area there is no resorption; the fluid is accumulating in the tissues, which can cause pronounced swelling. This is because in case of violation of the venous outflow a reflex blocking of the lymph drainage occurs and simultaneously venous and lymphatic stagnation develops. Another key factor that leads to local changes at venous hyperemia is oxygen deprivation (hypoxia) of the tissue, its development is linked to violation of diffusion. Hypoxia at venous hyperemia leads to disruption of tissue metabolism, atrophic and degenerative changes, excessive proliferation of the connective tissue. Along with local changes at venous hyperemia, general hemodynamic disorders of very serious consequences are possible. Most often they arise due to clogging of large venous collectors – the portal vein and the inferior vena cava. The accumulation of blood in these vascular reservoirs (about 90 % of the whole blood amount) is accompanied by a sharp decrease of the blood pressure and disorders in the feeding of vital organs. ISCHEMIA Violation of the peripheral circulation caused by a slowdown or complete cessation of the arterial blood delivery is called ischemia or local anemia. Ischemia is characterized by the following features: pallor ischemic areas of the organ, local temperature reduction, damaged sensitivity expressed in form of paresthesia (numbness, tingling, goosebumps), pain, reduced blood flow velocity and reduced size of the organ, lowered blood pressure in the artery section below the obstacle, reduction of O2 in the ischemic area of the organ or tissue, decreasing formation of interstitial fluid and reduced tissue turgor, dysfunction of the organ or tissue and degenerative changes in it. The factors causing ischemia vary: compression of the artery, obstruction of the artery opening, affected neuro-muscular system of the arterial wall. Accordingly, compression, obstructive and angiospastic types of ischemia are distinguished. Compressive ischemia is caused by compression of the drive artery, a scar, a tumor, a foreign body, etc. Obstructive ischemia is the result of a partial narrowing or complete closure of the artery opening with a thrombus or an embolus. Productive-infiltrative and inflammatory changes in the artery wall caused by atherosclerosis, obliterative endarteriit, nodular polyarteritis also effect weakening of the local blood flow along the obstructive ischemia pattern. Angiospastic ischemia is caused by irritation of the vasoconstrictive mechanism of the vessels and their reflex spasm caused by an emotional impact (fear, pain, anger), physical (cold, trauma, mechanical stimulation) and chemical factors, biological stimuli (bacterial toxins) etc. Under pathological conditions, angiospasm is characterized by long duration and significant severity, which can cause a sharp slowdown of the blood flow, to the point of its complete termination. Most often angiospasm develops in arteries of a relatively larger diameter inside the organ, following the pattern of vascular unconditioned reflexes from relevant interoreceptors. These reflexes are featured with expressed inertia and autonomy. An example of this type of vascular reactions may be spasm of coronary arteries during stimulation of the receptors of the internal organs (the intestines, bile ducts, ureter, bladder, lungs, uterus), reflex vasospasm of a paired organ (a kidney, a limb) located at stimulation of the opposite paired organ. Angiospastic ischemia can also be of the conditioned reflex type. Finally, direct stimulation of the vascular center located in the medulla oblongata with toxic substances contained in the blood that washes this center, mechanical stimulation of the subcortical structures regulating the vascular tone (in case of a tumoral process in the brain or bleeding in it, an increased intracranial pressure), presence of pathological, in particular inflammatory, processes in a certain area of the intermediate brain often lead to severe angiospastic manifestations too. Thus, angiospasm develops primarily due to activation of neurogenic, α-adrenergic, H1- histaminenergic, serotoninergic, and dopaminergic mechanisms. The sources of biogenic amines of nonmediator origin are the adrenal glands (catecholamines) and cells of the diffuse neuroendocrine system (APUD – the system for capturing and decarboxylation of amine predecessors), which are localized in various organs and tissues that are capable of producing 5-hidroksytryptamin (serotonin), histamine and dopamine. In the loose connective tissue, the additional sources of histamine are tissue basophils, in the blood – basophils (histamine) and platelets (histamine, serotonin). In the development of angiospasm, prostaglandins (PGF2α and thromboxane A2) play a certain role. The latter is formed in platelets; its secretion grows particularly sharply in their adhesion and aggregation at the damaged vascular wall. Finally, angiospastic effects are produced by vasoactive peptides – vasopressin and angiotensin II. Of great importance in the development of angiospastic reactions is endothelin-1 produced by vascular endothelium. First endothelium produces prohormone – big endothelin-1 consisting of 39 amino acid residues. Under the influence of the endothelin- transforming enzyme, big endothelin-1 produces endothelin-1 that contains 21 amino acid residues. This is the most powerful of all known vasoconstriction factors, and its activity is above 10 times higher than that of angiotensin II. The main mechanism of the endothelin-1 action is the receptor-mediated opening of the potential-dependent calcium channels and release of Ca2+ from the intracellular stores into the cytosol of smooth-muscle cells, which causes their contraction and proliferation, with thickening of the vascular walls and reduction of the vessel diameters. The synthesis of endothelin-1 is enhanced by angiotensin II, catecholamines, thrombin, hypoxia, growth factors, endothelial damage with oxidized lipoproteins or high blood pressure. All these factors activate transcription of the endothelin-1 gene. NO, PGE2, PGI2, PNP, conversely, inhibit the activity of this gene and reduce the secretion of endothelin and endothelin-1. Endothelin- 1 is engaged in formation of a number of pathological processes – myocardial infarction, cardiac arrhythmia, pulmonary and systemic hypertension, atherosclerosis etc. The mechanism activating contractile proteins of smooth-muscle cells depends on the condition of specific receptors. In particular, catecholamines act upon a-adrenergic receptors. In their activation, probably the first change is the one that takes place in the electrical properties of the membranes of the smooth-muscle cells due to increased permeability of the cell membrane for Na+ and Ca2+. A special role is played by Ca2+ that enters the cell from the intercellular fluid and is released from the sarcoplasmic grid. The result is activation of contractile proteins and reduction of smooth-muscle cells. Using blockers of slow calcium channels (Ca2+ antagonists – verapamil, nifedipine, befidyl), it is possible to prevent contraction caused by noradrenaline (by 50 % on the average). Angiotensin II is one of the most powerful vasoconstrictor agents, it produces a direct effect on smooth-muscle cells by depolarizing them due to increasing conduction of the channels for Na+ and Ca2+. An important role in causing ischemia is played by changes in the angiospastic sensitivity of the muscle elements of the vascular wall to noradrenaline and vasoactive peptides. For example, the sodium ions, accumulating in the smooth-muscle cells, increase the sensitivity of vessels to pressor substances – catecholamines, vasopressin, and angiotensin II. Currently, endothelial participation in the normal contraction and relaxation of the vessel smooth-muscles is brought into focus. Damage to endothelium eliminates its ability to detect the relaxing factor (NO), which results in enhanced spastic reactions. They can occur even when there is the influence of acetylcholine and bradykinin that normally cause vasodilation. The nature of metabolic, functional and structural changes in the ischemic area of the tissue or organ is determined by the degree of hypoxia, the severity of which depends on the development rate and type of ischemia, its duration, location, the character of collateral circulation, the functional state of the organ or tissue. Ischemia occurring in the area of complete obstruction or compression of the arteries, ceteris paribus causes more significant changes than in the case of spasm. Fast-developing ischemia, as well as the prolonged one, is characterized by the course being harder than at ischemia that develops slowly or lasts short. Of particular importance in the development of ischemia is sudden obstruction of the arteries, as this may be accompanied by reflex spasm of the system of the artery branches. Ischemia of vital organs (brain, heart) has heavier consequences than ischemia of kidneys, spleen, lungs, and with this second group of organs ischemia is, in its turn, heavier than that of skeletal, muscle, bone or cartilage tissue. This is because the tissue sensitivity to hypoxia is in fact determined by two factors: the metabolic activity of the tissue and the intensity of collateral circulation. Tissues with higher metabolism and higher specific activity are more sensitive to oxygen starvation. Thus, neurons undergo irreversible destruction already in 3 to 5 minutes of complete ischemia, cardiomyocytes – within 20 to 30 minutes, while fibroblasts in the myocardium are able to remain viable for several hours. The intensity of the collateral circulation is another limiting factor for the tissue stability against ischemia. As for the development degree of collaterals, all tissues can be divided into three types: absolutely adequate, relatively sufficient, and completely inadequate. If the collateral vessels, in the functionality aspect, are absolutely or relatively unable to compensate for poor circulation, the tissue is more prone to ischemia. A classic example of absolutely insufficient collateral circulation is the tissues of the brain and the myocardium. The intestines can be considered as tissues with relatively sufficient development of collaterals, and absolutely sufficient collateral blood flow is observed in the muscle tissue and skin. Finally, for ischemia the previous functional state of the organ or tissue is highly important. Slowdown of the arterial blood delivery under conditions of high functional activity of the oigan or tissue is more dangerous than at rest. Especially serious is inadequacy of the organ functionality and the blood supply to this organ, if there are organic changes in the arteries. This is due to the fact that organic changes of the vascular wall, on the one hand, limit the vessel ability to expand with an increase of the load, on the other hand, they increase the vessel sensitivity to various vasoconstrictor factors. In addition, the ability to enhance collateral circulation in sclerotic-modified vessels is also very limited. It is noteworthy that at ischemia changes in the processes of filtration, diffusion, and microvesicula transport are observed. Reduction of the pressure in the microvasculature by 10 mm Hg leads to nearly complete cessation of drainage and filtration of tissues. Oxygen delivery to tissues sharply reduces or terminates, causing hypoxia and rebuilding metabolism into anaerobic glycolysis. Reduced production of ATP impairs the processes of active transport. At ischemia, changes in tissues can be classified into several successive stages. 1. Reduction of the Krebs cycle efficiency, increase of the intensity of glycolysis and pentose cycle, overall reduction of the intensity of energy metabolism. 2. Macroergic phosphate depletion is accompanied by an overall increase of the Ca2+ level in the cell, especially after long periods of ischemia (Scheme 5). Scheme 5. The sequence of changes in the tissues caused by hypoxia or ischemia (according to V.H. Nailer, M.J. Dane, 1988) Figure 7 specifies sources and mechanisms of the increase of cytoplasmic Ca2+ concentration and the sequence of events caused by its excess: activation of calcium-sensitive proteinases and phospholipases, irreversible changes in the structure and integrity of the cell membranes, loss of the intracellular adenyl bases – precursors of the ATP synthesis. These changes inflict violation of the specific cell functions (contraction, secretion, etc.) and work of ion pumps, and development of necrobiosis. 3. The stage of enhanced biosynthesis of the major biochemical components of connective tissue – collagen, acidic and neutral glycosaminoglycans – is the basis for further sclerosis of the ischemic areas in the tissue or organ. Increased synthesis of nucleic acids is a prerequisite for this process. Reperfusion Syndrome. Reperfusion tissue damage occurs after restoration of blood flow in the ischemic area of the tissue or organ. The lack of oxygen and nutrients for the period of ischemia in the tissues creates conditions under which the antioxidant systems become depleted and do not renew. Therefore, restoration of circulation leads to development of free-radical damage in the ischemia tissue area due to formation of active oxygen radicals from the oxygen that starts its inflow in absence of the antioxidant protection. If the damage site in the tissue is large (e.g., at the syndrome of prolonged compression), the reperfusion-caused inflow of a large number of BAS and metabolites from the ischemia focus into the general circulation can inflict systemic effects, to the point of shock development.