Medical Physics Introduction to Nuclear Medicine PDF
Document Details
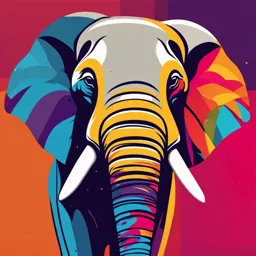
Uploaded by ImpressedBigfoot
Tehran University of Medical Sciences
Peyman Sheikhzadeh, PhD
Tags
Summary
This document is an introduction to nuclear medicine. It covers various medical imaging techniques, including X-rays, radiopharmaceuticals, and MRI and details the discovery and development of these technologies. It also introduces the concept of radioisotopes and radiopharmaceuticals, and describes the fundamental processes involved in Nuclear Medicine (NM) and Positron Emission Tomography (PET) methods.
Full Transcript
Medical Physics Introduction to Nuclear Medicine Peyman Sheikhzadeh, PhD Department of Nuclear Medicine Department of Medical Physics Tehran University of Medical Sciences, Tehran, Iran Medical imaging techniques Discovey of...
Medical Physics Introduction to Nuclear Medicine Peyman Sheikhzadeh, PhD Department of Nuclear Medicine Department of Medical Physics Tehran University of Medical Sciences, Tehran, Iran Medical imaging techniques Discovey of X-rays 1901: Wilhelm Roentgen received the Nobel Prize for recognition of the extraordinary services he has rendered by the discovery of the remarkable rays subsequently named after him Radiopharmaceuticals 1943: George de Hevesy received the Nobel Prize for his work on the use of isotopes as tracers in the study of chemical processes Development of X-ray CT 1979: Hounsfield & Cormack received the Nobel Prize for the development of X-ray computerized tomography (CT) Development of MRI 2003: Lauterbur & Mansfield received the Nobel Prize for their discoveries concerning development of Magnetic Resonance Imaging (MRI) Structural & Functional Imaging CT MRI STRUCTURE PET SPECT FUNCTION Nuclear Medicine is to physiology as Radiology is to anatomy WHAT DOES PROBE MEANS IN IMAGING ? MODALITY PROBE CHARACTERISTIC RADIOLOGY X-RAY ELECTRON DENSITY MAMMOGRAPHY X-RAY ELECTRON DENSITY CT X-RAY ELECTRON DENSITY ANGIOGRAPHY X-RAY ELECTRON DENSITY MRI RF PROTON DENSITY SPECT GAMMA RAY RADIONUCLIDE DIS. PET GAMMA RAY RADIONUCLIDE DIS. SONOGRAPHY ULTRASOUND ACOUSTIC IMPEDANCE HISTORY November 8, 1895 Wilhelm Conrad ROENTGEN, a German physicist, discovers accidentally unknown rays, able to pass through his hand and image the bones and his ring He called them…. “X-rays” He was awarded the first Nobel Prize for physics in 1901 One year later, Elihu Thomson demonstrates use of X-rays for diagnosis of fractures and location of foreign objects and Eddy C. Jerman starts the first school for Radiographers NM and PET Process N NH C NH2 H OH Radioisotopes and Radiopharmaceuticals Radioisotope is the radiation source (radioactive atom) Pharmaceutical is the vector molecule that targets the organ Radioisotope + pharmaceutical = radiopharmaceutical (radiotracer) CH2OH CH2OH O O + = OH OH 18F HO OH HO OH OH 18F Fluorine-18 + Glucose = 18F-FDG Why is NM different? Nuclear Medicine Other imaging modalities (X-ray, CT, MR, etc.) External emission source Emission source = Organ Detector Detector Functional imaging Anatomical imaging How does it work? How does it look? Nuclear Medicine acts as a complement to other modalities, not in replacement. NM Activity Poles Radiopharmacy (radiopharmaceuticals) Nuclear and biochemical industry Functional Imaging Clinical use Instrumentation (imaging) (camera, PET) Physicians Manufacturers/Physicist A Review Nuclear decay rules Based on conservation laws -decay: AXZ AYZ+1 + e- + ~ -decay: AXZ AYZ-1 + e+ + e-capture: AXZ + e- AYZ-1 + -decay and internal conversion: no changes for A & Z Radioactivity A (t): disintegration rate at time t (decays/sec) N(t): number of nuclei at time t : decay constant with units of 1/sec or 1/hr = ln2/T1/2 = 0.693/T1/2 half life: T1/2 = ln2/ = 0.693/ Radioactivity unit in SI: 1 Bq = 1 disintegrations per second (Becquerel) traditional unit: 1 Ci = 3.7×1010 dps (1g of Ra-226, extracted first by Mme. Curie) 1 mCi = 37 MBq NM imaging: ~ 1 to 30 mCi (30 – 1100 MBq) Physical Half-life (Tp) Tp = time required for the number of radioactive atoms to reduce by one half Basic equations: Nt = N0e-t or At = A0e-t Tp = 0.693 / = 0.693 / Tp N0 = Initial number of radioactive atoms Nt = number of radioactive atoms at time t Effective half life Te = Time to reduce radiopharmaceutical in the body by one half due to functional clearance and radioactive decay 1 1 Te = + 𝑇𝑝 𝑇𝑏 𝑇𝑝𝑇𝑏 Te = 𝑇𝑝+𝑇𝑏 if Tp >> Tb, Te ≈ Tb if Tp 99.95% of s counted stopped Typical efficiency of a LEHR collimator ~ 0.02 % Scatter Major source of image degradation in NM Increases image noise and reduces lesion contrast Windowing the photopeak allows suppression of scatter events (but not complete elimination) Scatter in patient Photopeak scatter Image degradation Septal penetration Counted Image degradation Simultaneous detections detected detected Image degradation Scatter detected System spatial resolution Rsys = R 2int + R col 2 system resolution Rsys intrinsic (detector) resolution Rint collimator resolution Rcol Rint typically 2.9mm to 4.5mm Rcol typically 7.4mm to 13.2mm Rsys typically 1cm Collimator Resolution Spatial resolution degrades with increasing pt – collimator distance. Data acquisition collimator: match the radioisotope energy window: match the radioisotope pixel size: 1/3 ~ 1/2 of spatial resolution detector size matrix size = pixel size usually, 64×64, 128×128 or 256×256 2 bytes in pixel depth count rate < 20000/sec patient close to the detector Effect of matrix size 64×64 128×128 Planar NM Imaging Uniformity a collimator defect a bad PMT shift of energy peak Bar phantom made of lead stripes with different orientations and spacing in 4 quadrants to measure extrinsic and intrinsic linearity and spatial resolution extrinsic: place a Co-57 sheet source with the bar phantom on the top of the collimator intrinsic: take collimator off and place a Tc-99m pt source point source 5 × detector size away ray bar detector Tomographic NM imaging (SPECT) Single Photon Emission Computed Tomography Tomographic imaging (SPECT) Produce tomographic images by acquiring conventional gamma camera projection data at several angles around the patient Similar to CT SPECT Provide 3-D images to eliminate overlaying and underlying activity of a slice better contrast more accurate lesion localization more demanding technically and longer data acquisition more severe image noise SPECT data acquisition Generally, two detectors mounted at 180 or 90 on a rotation gantry SPECT data acquisition A sequence of 2-D static images at different angular positions (views) detector rotation range 180º with 2 perpendicular detectors or 360º with 2 opposite detectors SPECT data acquisition Circular or elliptical orbit, which is better? closer to the patient better spatial resolution SPECT Image Acquisition (Improves spatial resolution) Data Collection: Configuration Non Cardiac Cardiac SPECT Image Acquisition Typically 2 camera heads, rotating around patient Projection images every 3 – 6 degrees ~ 30 s / projection, ~ 15 minutes total Matrix: 64 x 64 or 128 x 128 Data Collection: Angular Stops 3 to 6 degrees is common a lesser number causes streaking a larger number does not improve image quality Step-shoot or continuous acquisition, which is better?????? Step & Shoot Characteristics Some loss of time Less Blur View number for 360º SPECT 128 views 64 views number of views = matrix size 43 views 32 views An image with 128 x 128 matrix Contains 128 projections Each projection has 128 data points Equivalent to 128 slice CT (i.e., 128 tomographic slices per rotation) Sinogram (for one of many slices) Back Projection Leads to blurring in image (streaks and star-like artifacts) Filtered Backprojection Suppress blurring through filtering the projections A high-pass filter (ramp filter) can be used to suppress blurring Filtered Back Projection (of noiseless data) Filter Applied filter is the product of: Ramp User selected/characterized filter Shepp-Logan Hahn Butterworth Weiner Hamming Hanning (MCG: Philips, generally turned off) Selection of Filters for SPECT Filters trade noise for resolution No standard way to optimize filter choice Patient to patient variation Physician preferences Vendor recommendation Iterative Reconstruction (IR) Filtered Back Projection has some limits Various corrections needed Attenuation Compton Scatter Ordered Subsets Expectation Maximization (OSEM) is a common iterative reconstruction algorithm Iterative Reconstruction Slow compared to filtered back projection Commonly used for PET Being used increasingly in SPECT Image recon - Iterative Common IR recon is the OSEM For OSEM, # iterations (I) and # subsets (S) affect image quality # (I/S) noise, but sharper images Iterative reconstruction algorithms © Physics in Nuclear Medicine: Cherry, Sorenson and Phelps 85 Brain Phantom IR FBP OSEM Non-filter Noise Factors Collimator Matrix 64 x 64 128 x128 Slice thickness Time per stop/ Number of Stops Administered Dose Data Collection: Counts Determination of Number of Image Counts Activity in patient Time per stop Number of Stops Attenuation Correction Like all radionuclide imaging there is a problem due to attenuation. Correction can be important for judging the activity of lesions Uniform phantom with evenly distributed 99mTc Low counts Chang in center method Proper AC SPECT/CT Patient Studies Advantages No overlapping structures 3 dimensional lesion locations Fusion with high resolution images (CT, MRI) Disadvantages Time consuming (motion) Images are noisy Introduction to the physics of Positron Emission Tomography Positron is an ‘Antimatter’ particle E = 1.02 MeV Note the diametrically opposed nature of the annihilation coincidence detection PET image formation t1 t = t1 – t2 t < 5 (to 12) ns ? Yes Register as a “coincident” event t2 Lines of response (LOR) Positional information is gained LOR is assigned by electronic coincidence circuitry + emitters used in PET Proton-rich nuclei: positron emission p n + e+ + 18F 18O + e+ + T1/2 = 110 min 9 8 15O 8 15N7 + e+ + T1/2 = 2 min 13N 7 13C6 + e+ + T1/2 = 10 min 11C 6 11B5 + e+ + T1/2 = 20 min 103 Ultimate spatial resolution in PET The uncertainties in annihilation (location & residual particle momentum) determine the ultimate spatial resolution (~ 2 mm) 104 Types of coincidences (correct LOR assigned) (incorrect LOR assigned) True Scatter Random True coincidences form a “true” distribution of radioactivity Scatter & random coincidences distort the distribution of radioactivity, add to image noise, degrade image quality No collimators in a PET scanner Photon direction determined by LOR no collimators Absence of lead improves: detection efficiency (count rate) spatial resolution 106 Detector materials BGO (Bi4Ge3O12) used by GE LSO (Lu2SiO5) used by Siemens GSO (Gd2SiO5 ) used by Philips LYSO (Lu2YSiO5, 9(L):1(Y)) used by all 107 Advantages of PET imaging No collimators higher detection efficiency and better spatial resolution Ring detectors higher detection efficiency Block detectors higher detection efficiency and better spatial resolution 108 PET Data Corrections Attenuation CT based Normalization Correction for variation in performance of ~20,000 individual detectors Random coincidences Delayed coincidence time window (~64 ns) Scattered radiation Modeling from transmission & emission data Extrapolation from tails of projections Dead time Empirical models 109 CT number: Hounsfield Units CT number (x,y) = 1000 ((x,y) – water) / water SPECT vs PET SPECT PET (Step-and-shoot acquisition) (Simultaneous acquisition) SPECT vs PET imaging Attribute SPECT PET Detection Single s Coincident s Radionuclides 99mTc, 67Ga, 111In 18F, 82Rb, 13N, E 70 – 300 keV 511 keV Spatial res. ~ 10 – 12 mm ~ 5 - 6 mm Atten.Correction No / Yes* Yes * Possible with SPECT/CT or transmission source systems PET systems Multiple rings ~5 rings ~8 slices/ring Ring diameter ~ 80 to 92 cm Transverse FOV ~ 60 cm Axial FOV ~ 15 - 20 cm Attenuation correction X-ray CT 2015 Nuclear Medicine Physics for Radiology Residents Sameer Tipnis, PhD, DABR Detector materials BGO (Bi4Ge3O12) used by GE LSO (Lu2SiO5) used by Siemens GSO (Gd2SiO5 ) used by Philips LYSO (Lu2YSiO5, 9(L):1(Y)) used by all 115 Stopping power (attenuation coefficients) 511 keV 140 keV NaI 0.32/cm 2.44/cm BGO 0.86/cm 11.76/cm LSO 0.81/cm 9.26/cm GSO 0.67/cm 6.37/cm LYSO 0.50/cm _____________________________________________________________________________ N(x) = N e-x For x = 3 cm, NBGO = 7.5% (for a 3 cm thick BGO detector, 92.5% of 511 keV photons absorbed) 116 Scintillation decay time If time interval between detecting 2 ’s too short pile-up effect reduced count rate and artifacts NaI BGO LSO GSO LYSO 230 ns 300 ns 40 ns 60 ns 53 ns 117 Energy resolution Depending on both fluctuation of blue photon number and light output NaI BGO LSO GSO LYSO 10% 17% 12-18% 10% 12-18% 118 Detector blocks PET 20 – 30 mm PET spatial resolution is primarily dictated by dimensions of individual detector elements (width ~ 4 – 6 mm) Each detector element optically isolated by reflective material in crystal cuts PMT array can determine which detector element(s) absorbed 511 keV photon Detector blocks Each detection element: 4 mm (T) × 4 mm (A) × 30 (R) mm Small tangential and axial sizes good spatial resolution Large radial size high stopping power high count rate All blocks acquire data simultaneously significantly increasing count rate 120 Advantages of PET imaging No collimators higher detection efficiency and better spatial resolution Ring detectors higher detection efficiency Block detectors higher detection efficiency and better spatial resolution 121 2014 PET image of the ACR phantom 7.9 mm 122 2014 SPECT image of the ACR phantom as 9.5 mm 31.8 mm 15.9 mm Sphere diameters: 9.5, 12.7, 15.9, 19.1, 25.4, and 31.8 123 mm Time-of-flight PET ToF is used to improve SNR. The improved SNR is used either for better image quality or for shorter scan time. No additional hardware is needed except more CPU due to the heavy computation. 0.067 ns 0.585 ns t2 t2 t1 t1 124 Time of flight PET image of a big patient Discovery PET/CT 710 (GE) Biograph TruePoint PETCT (Siemens) Ingenuity TF PET/CT (Philips) Attenuation Correction in PET/CT Attenuation Correction Without AC With AC Photon attenuation in PET High uptake in lungs Low uptake in others High uptake in skin w/o attenuation attenuation compensation compensation 129 Typical PET / CT imaging (1) (2) 512 x512 128 x128 120 kVp 511 keV Role of FDG NOTE - FDG is NOT cancer specific and will accumulate in areas with high levels of metabolism and glycolysis increased uptake can be expected in sites of: (1) hyperactivity (muscular, nervous) (2) active inflammation (infection, sarcoid, arthritis, etc.) (3) tissue repair, etc. Hyperactivity High FDG uptake in pectoralis major after strenuous exercise 24 hours prior to study Inadequate fasting 45 min Overnight fasting fasting Semiquantitative PET: Standard Uptake Value (SUV) Defined as the ratio of activity concentrations SUV = conc. in vol. of tissue / conc. in whole body SUV = (MBq/kg) / (MBq/kg) Usually, SUV ~ 2.5 taken as cut-off between malignant and non-malignant pathology SUV in clinical studies Numerator: highest pixel value (SUVmax) from an ROI Or SUVmean Denominator: Activity administered/ body mass Or lean body mass Or body surface area SUV will depend on – physiologic condition, uptake time, fasting state, etc. Image noise, resolution, ROI definition Small changes in SUV need to be interpreted carefully Requirement for reproducible SUV 18FDG uptake period, scan length, scanning range, scanning direction (e.g. head to toe) Patient preparation: fasting, medication Reconstruction parameters: slice thickness, filters Region-of-interest definition (SUVmax / SUVmean/ body mass/ lean body mass/ body surface area) Consistency is the most important factor! Clinical Use of PET Oncology Cardiac & Neuro (~ 90%) (~ 10%) Typical oncology protocol Administered dose – 10 to 20 mCi FDG ~ 60 mins. in “quiet” room” to allow adequate uptake and trapping, clearance from blood Scanning – typically “eyes-to-thighs” 6 to 7 “bed” positions (each ~ 15 cm FOV) Total scan time is ~ 30 mins. (3 mins/bed) With time, SUVtumor & SUVbkg. Patient dose (FDG) Effective dose to pt. 10 mCi (370 MBq) injection ~ 7 mSv Organ of max. dose: bladder Equivalent dose 10 mCi (370 MBq) injection ~ 63 mGy CT (for AC) ~ 5 mSv CT (Diag. / contr.) ~ 15 – 18 mSv SPECT & PET SPECT – 2 views from opposite sides Res. collimator res., which degrades rapidly with increasing distance from collimator face PET – Simultaneous acquisition Res. detector width; is max in center of ring SPECT sensitivity ~ 0.02% Huge losses due to absorptive collimators PET sensitivity- 3D ~ 2% or higher High sensitivity due to coincidence detection (electronic collimation) Advantages of PET over SPECT Superior spatial resolution Higher sensitivity Attenuation Correction Limitation of Functional Imaging LIMITED SPATIAL RESOLUTION POOR SIGNAL TO NOISE RATIO POOR UPTAKE TO THE RADIOTRACER IN THE DISEASED CONDITION REGISTERATION WITH AN ANATOMICAL IMAGE CAN BE USEFUL…… Medical imaging techniques Anatomical Functional Hybrid Fusing Anatomy and Function FUSED BY H.N. Wagner IN 1968 History of dual-modality imaging SPECT/CT The first prototype SPECT/CT was built by B. Hassegawa in 1990 The first commercial SPECT/CT was installed in 1999 PET/CT The first prototype system was built by D. W. Townsend in 1998 The first commercial PET/CT was installed in 2000 Gamma Camera Components Stationary gantry Transmission Rotating gantry acquisition system Detectors Patient table Acquisition station Processing station PET Scanner Components Gantry Detector ring Acquisition and Patient table processing station PET/CT Scanner Gantry PET detector CT module Acquisition and Patient table processing station PET/CT Scanner PET CT The PET/CT Power..! CT: Is there a Lesion? PET : Where is the Lesion PET-CT : gives Located Exactly? the answers! Anatomic and Functional Imaging Anatomic Imaging CT Functional Imaging NM Complementation of modalities Fusion CT + NM Effective dose of NM procedures 152 Dose limits Occupational: ALARA 1 & ALARA 2 Embryo/fetus: 5 mSv total 153