Talaro's Foundations in Microbiology Chapter 7 PDF
Document Details
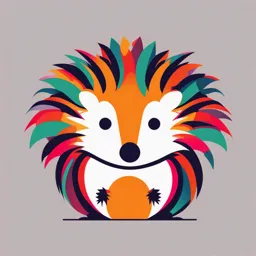
Uploaded by TrustedRetinalite4204
Barry Chess
Tags
Summary
This document, Chapter 7 of Talaro's Foundations in Microbiology, gives a detailed look into microbial nutrition. It covers bioelements, essential nutrients, macronutrients (in large quantities), and micronutrients (in small amounts) along with examples. The twelfth edition text is a valuable resource for microbiology students.
Full Transcript
Because learning changes everything. ® Chapter 7 Microbial Nutrition, Ecology and Growth Talaro’s Foundations in Microbiology Twelfth Edition Barry Chess © McGraw Hill LLC. All rights reserved. No reproduction or distribution without the prior written consent of...
Because learning changes everything. ® Chapter 7 Microbial Nutrition, Ecology and Growth Talaro’s Foundations in Microbiology Twelfth Edition Barry Chess © McGraw Hill LLC. All rights reserved. No reproduction or distribution without the prior written consent of McGraw Hill LLC. Microbial Nutrition Process by which chemical compounds (nutrients) are acquired from the environment to sustain life Bioelements – basic requirements for life (carbon, hydrogen, oxygen, phosphorus, potassium, nitrogen, sulfur, calcium, iron, sodium, chlorine, magnesium) Essential nutrients – substance (element or compound) an organism must get from a source outside its cells Macronutrients – required in large quantities; play principal roles in cell structure and metabolism (proteins, carbohydrates) Micronutrients or trace elements – required in small amounts; involved in enzyme function and maintenance of protein structure (manganese, zinc, nickel) © McGraw Hill LLC. 2 Energy Extraction of Microorganisms Source: Eric Erbe/Chris Pooley, USDA-ARS © McGraw Hill LLC. 3 Nutrients Organic nutrients – contain carbon and hydrogen atoms and are usually the products of living things Methane (CH4), carbohydrates, lipids, proteins, and nucleic acids Inorganic nutrients – atom or molecule that contains a combination of atoms other than carbon and hydrogen Metals and their salts (magnesium sulfate, ferric nitrate, sodium phosphate), gases (oxygen, carbon dioxide) and water © McGraw Hill LLC. 4 Chemical Analysis of Cell Contents 70% water 97% of dry cell weight is organic compounds: Proteins the most prevalent All these organic compounds are composed of 6 elements: Carbon Hydrogen Oxygen Phosphorous Sulfur Nitrogen © McGraw Hill LLC. 5 Essential Biological Nutrients 1 TABLE 7.1 Sources and Biological Functions of Essential Elements and Nutrients 1) Carbon Elements/Nutrients Forms Found in Nature: Sources/Reservoirs of Compounds: Air (0.036%*) Sediments/Soils Living Things © McGraw Hill LLC. 6 Essential Biological Nutrients 2 The “TABLE 7.1” continues on this slide. Significance to cells: CO2 is produced by respiration and used in photosynthesis; CO32− is found in cell walls and skeletons; organic compounds are essential to the structure and function of all organisms and viruses. 2) Nitrogen Elements/Nutrients Forms Found in Nature: N2 gas NO3- (nitrate) NO2- (nitrite) NH3 (ammonium) Organic nitrogen (proteins, nucleic acids) © McGraw Hill LLC. 7 Essential Biological Nutrients 3 The “TABLE 7.1” continues on this slide. Sources/Reservoirs of Compounds: Air (79%*) Soil and water Soil and water Soil and water Organisms © McGraw Hill LLC. 8 Essential Biological Nutrients 4 The “TABLE 7.1” continues on this slide. Significance to cells: Nitrogen gas is available only to certain microbes that fix it into other inorganic nitrogen compounds—nitrates, nitrites, and ammonium—the primary sources of nitrogen for algae, plants, and the majority of bacteria; animals and protozoa require organic nitrogen; all organisms use NH3 to synthesize amino acids and nucleic acids. © McGraw Hill LLC. 9 Essential Biological Nutrients 5 The “TABLE 7.1” continues on this slide. 3) Oxygen Elements/Nutrients Forms Found in Nature: Sources/Reservoirs of Compounds: Air (20%*), a major product of photosynthesis Soil © McGraw Hill LLC. 10 Essential Biological Nutrients 6 The “TABLE 7.1” continues on this slide. Significance to cells: Oxygen gas is necessary for the metabolism of nutrients by aerobes. Oxygen is a significant element in organic compounds and inorganic compounds (see water, sulfates, phosphates, nitrates, carbon dioxide in this table). © McGraw Hill LLC. 11 Essential Biological Nutrients 7 The “TABLE 7.1” continues on this slide. 4) Hydrogen Elements/Nutrients Forms Found in Nature: Sources/Reservoirs of Compounds: Waters, swamps, mud Volcanoes, vents Swamps organisms © McGraw Hill LLC. 12 Essential Biological Nutrients 8 The “TABLE 7.1” continues on this slide. Significance to cells: Water is the most abundant compound in cells and a solvent for metabolic reactions; gases are produced and used by bacteria and archaea; H+ ions are the basis for transfers of cellular energy and help maintain the pH of cells. 5) Phosphorus Elements/Nutrients Forms Found in Nature: © McGraw Hill LLC. 13 Essential Biological Nutrients 9 The “TABLE 7.1” continues on this slide. Sources/Reservoirs of Compounds: Rocks, mineral deposits Soil Significance to cells: Phosphate, a key component of DNA and RNA, is critical to the genetic makeup of cells and viruses; also found in ATP and NAD, where it takes part in numerous metabolic reactions; its presence in phospholipids provides stability to cell membranes. © McGraw Hill LLC. 14 Essential Biological Nutrients 10 The “TABLE 7.1” continues on this slide. 6) Sulfur Elements/Nutrients Forms Found in Nature: S SO42- (sulfate) SH (sulfhydryl) Sources/Reservoirs of Compounds: Mineral deposits volcanic sediments Soil © McGraw Hill LLC. 15 Essential Biological Nutrients 11 The “TABLE 7.1” continues on this slide. Significance to cells: Elemental sulfur (S) is oxidized by some bacteria as an energy source; sulfur is found in vitamin B1; sulfhydryl groups are part of certain amino acids, where they form disulfide bonds that shape and stabilize proteins. 7) Potassium Elements/Nutrients Forms Found in Nature: Sources/Reservoirs of Compounds: Mineral deposits, ocean water, soil Significance to cells: Plays a role in protein synthesis and membrane transport © McGraw Hill LLC. 16 Essential Biological Nutrients 12 The “TABLE 7.1” continues on this slide. 8) Sodium Elements/Nutrients Forms Found in Nature: Sources/Reservoirs of Compounds: Same as potassium Significance to cells: Major participant in membrane actions; maintains osmotic pressure in cells. 9) Calcium Elements/Nutrients Forms Found in Nature: © McGraw Hill LLC. 17 Essential Biological Nutrients 13 The “TABLE 7.1” continues on this slide. Sources/Reservoirs of Compounds: Oceanic sediments, rocks, and soil Significance to cells: A component of protozoan shells (as CaCO3); stabilizes cell walls; adds resistance to bacterial endospores 10)Magnesium Elements/Nutrients Forms Found in Nature: Sources/Reservoirs of Compounds: Geologic sediments, rocks, and soil © McGraw Hill LLC. 18 Essential Biological Nutrients 14 The “TABLE 7.1” continues on this slide. Significance to cells: A central atom in the chlorophyll molecule; required for function of membranes, ribosomes, and some enzymes 11)Chloride Elements/Nutrients Forms Found in Nature: Sources/Reservoirs of Compounds: Ocean water, salt lakes Significance to cells: May function in membrane transport; required by obligate halophiles to regulate osmotic pressure © McGraw Hill LLC. 19 Essential Biological Nutrients 15 The “TABLE 7.1” continues on this slide. 12)Zinc Elements/Nutrients Forms Found in Nature: Sources/Reservoirs of Compounds: Rocks, Soil Significance to cells: An enzyme cofactor, regulates eukaryotic genetics 13)Iron Elements/Nutrients Forms Found in Nature: © McGraw Hill LLC. 20 Essential Biological Nutrients 16 The “TABLE 7.1” continues on this slide. Sources/Reservoirs of Compounds: Rocks, Soil Significance to cells: Essential element for the structure of respiratory proteins (cytochromes) 14) Micronutrients Elements/Nutrients Forms Found in Nature: copper, cobalt, nickel, molybdenum, manganese, iodine Sources/Reservoirs of Compounds: Geologic sediments, soil © McGraw Hill LLC. 21 Essential Biological Nutrients 17 The “TABLE 7.1” continues on this slide. Significance to cells: Required in tiny amounts to serve as cofactors in specialized enzyme systems of some microbes but not all * As a portion of the earth’s atmosphere. © McGraw Hill LLC. 22 Sources of Essential Nutrients: Carbon-Based Sources of the element carbon defines two basic nutritional types: Heterotroph – must obtain carbon in an organic form such as proteins, carbohydrates, lipids, and nucleic acids made by other living organisms Autotroph – an organism that uses CO2, an inorganic gas, as its carbon source Not nutritionally dependent on other living things © McGraw Hill LLC. 23 Growth Factors: Essential Organic Nutrients Organic compounds that cannot be synthesized by an organism because they lack the genetic and metabolic mechanisms to synthesize them Growth factors must be provided as a nutrient for survival Essential amino acids, vitamins © McGraw Hill LLC. 24 Classification of Nutritional Types Main determinants of nutritional type are: Carbon source Heterotroph – from other organisms Autotroph – uses CO2 as source Energy source Chemotroph – gain energy from chemical compounds Phototrophs – gain energy through photosynthesis © McGraw Hill LLC. 25 Nutritional Categories TABLE 7.2 Nutritional Categories of Microbes by Energy and Carbon Source Category / Carbon Energy Source Example Source Autotroph/CO2 Nonliving Environment Photoautotroph Sunlight Photosynthetic organisms, such as algae, plants, cyanobacteria Chemoautotroph Simple inorganic chemicals Only certain bacteria and archaea, such as methanogens and deep-sea vent bacteria Heterotroph/ Organic Other Organisms Carbon or Sunlight Chemoheterotroph Metabolic conversion of the nutrients Protozoa, fungi, many bacteria, animals from other organisms 1. Saprobe Metabolize the organic matter Fungi, bacteria, some protists (decomposers) from dead organisms 2. Symbiotic microbes Obtain organic matter from living Parasites, commensals, mutualistic organisms microbes Photoheterotroph Sunlight or organic matter Purple and green photosynthetic bacteria © McGraw Hill LLC. 26 Autotrophs and Their Energy Sources 1 Photoautotrophs Oxygenic photosynthesis (plants, algae, and cyanobacteria) Produce oxygen and use chlorophyll as the primary pigment Anoxygenic photosynthesis (purple and green sulfur bacteria) no oxygen but sulfur production, use bacteriochlorophyll as pigment © McGraw Hill LLC. 27 Autotrophs and Their Energy Sources 2 Chemoautotrophs (lithoautotrophs) survive totally on inorganic substances Methanogens, a kind of chemoautotroph, produce methane gas under anaerobic conditions (a): Dr. Adrian Hetzer © McGraw Hill LLC. 28 Autotrophs and Their Energy Sources 3 (b): Electron Microscope Lab, UC Berkeley © McGraw Hill LLC. 29 Heterotrophs and Their Energy Sources 1 Majority are chemoorganotrophs: Derive carbon and energy from organic compounds, that is, aerobic respiration Two categories: Saprobes: free-living microorganisms that feed on organic detritus from dead organisms Opportunistic pathogen Facultative parasite Symbionts: derive nutrients from host Can obtain nutrients from living or nonliving source Some are obligate parasites © McGraw Hill LLC. 30 Heterotrophs and Their Energy Sources 2 The figure of “Heterotrophs and Their Energy Sources.” a) Cell wall acts as a barrier. b) Enzymes are transported outside the wall. Access the text alternative for slide images. © McGraw Hill LLC. 31 Heterotrophs and Their Energy Sources 3 The figure of “Heterotrophs and Their Energy Sources” continues on this slide. c) Enzymes hydrolyze the bonds on nutrients. d) Smaller molecules are transported across the wall and cell membrane into the cytoplasm. Access the text alternative for slide images. © McGraw Hill LLC. 32 Concept Check: (1) If an organism is degrading large organic molecules to get both carbon and energy, it would be best described as a: A. Photoheterotroph B. Photoautotroph C. Chemoorganotroph D. Chemoautotroph © McGraw Hill LLC. 33 Concept Check: (2) If an organism is degrading large organic molecules to get both carbon and energy, it would be best described as a: A. Photoheterotroph B. Photoautotroph C. Chemoorganotroph D. Chemoautotroph Answer: C © McGraw Hill LLC. 34 Transport: Movement of Substances Across the Cell Membrane 1 Passive transport – does not require energy; substances exist in a gradient and move from areas of higher concentration toward areas of lower concentration Diffusion Osmosis – diffusion of water Facilitated diffusion – solutes that require a carrier © McGraw Hill LLC. 35 Transport: Movement of Substances Across the Cell Membrane 2 Active transport – requires energy and carrier proteins; gradient independent Carrier-mediated active transport Group translocation – transported molecule chemically altered Bulk transport – endocytosis, exocytosis, pinocytosis © McGraw Hill LLC. 36 Diffusion and Molecular Motion Net Movement of molecules down their concentration gradient by random thermal movement (passive transport) © McGraw Hill LLC. 37 Diffusion of Water: Osmosis 1 Living membranes generally block the entrance and exit of larger molecules and permit free diffusion of water (passive transport) When concentrations of the solutions differ, one side will experience a net loss of water and the other a net gain of water until equilibrium is reached © McGraw Hill LLC. 38 Diffusion of Water: Osmosis 2 The figure of “Osmosis.” a) Inset shows a close-up of the osmotic process. The gradient goes from the outer container (higher concentration of H2O) to the sac (lower concentration of H2O). Some water will diffuse in the opposite direction but the net gradient favors osmosis into the sac. Access the text alternative for slide images. © McGraw Hill LLC. 39 Diffusion of Water: Osmosis 3 The figure of “Osmosis” continues on this slide. b) As the H2O diffuses into the sac, the volume increases and forces the excess solution into the tube, which will rise continually. Access the text alternative for slide images. © McGraw Hill LLC. 40 Diffusion of Water: Osmosis 4 The figure of “Osmosis” continues on this slide. c) Even as the solution becomes diluted, there will still be osmosis into the sac. Equilibrium will not occur because the solutions can never become equal. (Why?) Access the text alternative for slide images. © McGraw Hill LLC. 41 Effect of Differing Osmotic Content Solutions 1 The figure of “Tonicity.” Cells with cell walls Isotonic Solution: Water concentration is equal inside and outside the cell, thus rates of diffusion are equal in both directions. Access the text alternative for slide images. © McGraw Hill LLC. 42 Effect of Differing Osmotic Content Solutions 2 The figure of “Tonicity” continues on this slide. Hypotonic Solution: Net diffusion of water is into the cell; this swells the protoplast and pushes it tightly against the wall. Wall usually prevents cell from bursting. Access the text alternative for slide images. © McGraw Hill LLC. 43 Effect of Differing Osmotic Content Solutions 3 The figure of “Tonicity” continues on this slide. Hypertonic Solution: Water diffuses out of the cell and shrinks the cell membrane away from the cell wall; process is known as plasmolysis. Access the text alternative for slide images. © McGraw Hill LLC. 44 Effect of Differing Osmotic Content Solutions 4 The figure of “Tonicity” continues on this slide. Cells lacking walls Isotonic Solution: Rates of diffusion are equal In both directions. Access the text alternative for slide images. © McGraw Hill LLC. 45 Effect of Differing Osmotic Content Solutions 5 The figure of “Tonicity” continues on this slide. Hypotonic Solution: Diffusion of water into the cell causes it to swell, and may burst it if no mechanism exists to remove the water. Access the text alternative for slide images. © McGraw Hill LLC. 46 Effect of Differing Osmotic Content Solutions 6 The figure of “Tonicity” continues on this slide. Hypertonic Solution: Water diffusing out of the cell causes it to shrink and become distorted. Access the text alternative for slide images. © McGraw Hill LLC. 47 Movement of Solutes: Facilitated Diffusion 1 Passive transport mechanism that utilizes a carrier protein in the membrane that will bind a specific substance Carrier proteins exhibit specificity (they bind and transport only a single type of molecule) Facilitated diffusion exhibits saturation (rate of transport limited by the number of binding sites on the transport proteins) © McGraw Hill LLC. 48 Movement of Solutes: Facilitated Diffusion 2 Access the text alternative for slide images. © McGraw Hill LLC. 49 Carrier Mediated Active Transport 1 Permeases and pumps: Specific membrane–bound transporter proteins that interact with nearby solute-binding proteins that carry essential solutes (sodium, iron, sugars) Once a solute-binding protein attaches to a specific site in the transporter protein, an ATP is activated and generates energy to pump the solute into the cell’s interior through a special channel. © McGraw Hill LLC. 50 Carrier Mediated Active Transport 2 The figure of “Carrier Mediated Active Transport.” Access the text alternative for slide images. © McGraw Hill LLC. 51 Carrier Mediated Active Transport 3 The figure of “Carrier Mediated Active Transport” continues on this slide. a) Carrier-mediated active transport. (1) Membrane- bound transporter proteins (permeases) interact with nearby solute-binding proteins that carry essential solutes (sodium, iron, sugars). (2) Once a binding protein attaches to a specific site, an ATP is activated and generates energy to pump the solute into the cell's interior through a special channel in the permease. © McGraw Hill LLC. 52 In Group Translocation 1 Another type of active transport in which energy is conserved by coupling transport with synthesis A specific molecule is actively captured, but it is chemically altered or activated for use in the cell on its passage through the membrane protein carrier © McGraw Hill LLC. 53 In Group Translocation 2 b) In group translocation, (1) a specific molecule is actively captured, but on its passage through the membrane protein carrier (2) it is chemically altered or activated for use in the cell. By coupling transport with synthesis, the cell conserves energy. Access the text alternative for slide images. © McGraw Hill LLC. 54 Endocytosis: Eating and Drinking by Cells 1 Endocytosis: bringing substances into the cell through a vesicle or phagosome Phagocytosis ingests substances or cells (pseudopods) Pinocytosis ingests fluids and/or dissolved substances (microvilli) © McGraw Hill LLC. 55 Endocytosis: Eating and Drinking by Cells 2 c) Endocytosis. With phagocytosis, solid particles are engulfed by flexible cell extensions or pseudopods (1-4) (1,000x). (5) With pinocytosis, fluids and/or dissolved substances are enclosed in vesicles by very fine protrusions called microvilli (3.000x). Oil droplets fuse with the membrane and are released directly into the cell. Access the text alternative for slide images. © McGraw Hill LLC. 56 TABLE 7.3 Summary of Transport Processes in Cells 1 General Nature of Transport Examples Description Qualities Process Energy expenditure by the cell is not required. Nonspecific Brownian Substances exist in a A fundamental property movement gradient and move from Diffusion and of atoms and Movement of small Passive areas of higher osmosis molecules that exist uncharged concentration toward in a state of random molecules across areas of lower motion membranes concentration in the gradient. Molecule binds to a Molecule specific; Facilitated carrier protein in transports both diffusion membrane and is ways carried across to Transports sugars, other side. amino acids, water Energy expenditure is Atoms or molecules are required. Molecules need Carrier- pumped into or out of Transports simple not exist in a gradient. mediated the cell by specialized sugars, amino Active Rate of transport is active receptors; driven by acids, inorganic increased. transport ATP or other high- ions (Na+, K+) Transport may occur against energy molecules a concentration gradient. © McGraw Hill LLC. 57 TABLE 7.3 Summary of Transport Processes in Cells 2 General Nature of Transport Examples Description Qualities Process Molecule is moved across membrane Alternate system for Group and simultaneously transporting translocation converted to a nutrients (sugars, metabolically useful amino acids) substance. Mass transport of large Process is particles, cells, and endocytosis; Bulk transport liquids by examples are engulfment and phagocytosis and vesicle formation pinocytosis © McGraw Hill LLC. 58 TABLE 7.3 Summary of Transport Processes in Cells 3 General Process: Passive Active © McGraw Hill LLC. 59 Concept Check: (3) If a cell is in a concentrated glucose solution and the glucose is moving into the cell through a carrier protein, this would be an example of A. Diffusion B. Facilitated Diffusion C. Osmosis D. Endocytosis E. Pinocytosis © McGraw Hill LLC. 60 Concept Check: (4) If a cell is in a concentrated glucose solution and the glucose is moving into the cell through a carrier protein, this would be an example of A. Diffusion B. Facilitated Diffusion C. Osmosis D. Endocytosis E. Pinocytosis Answer: B © McGraw Hill LLC. 61 Environmental Factors That Influence Microbes Niche: totality of adaptations organisms make to their habitat Environmental factors affect the function of metabolic enzymes Factors include: Temperature Oxygen requirements pH Osmotic pressure Barometric pressure © McGraw Hill LLC. 62 Adaptations to Temperature The range of temperatures for microbial growth can be expressed as three cardinal temperatures Minimum temperature – lowest temperature that permits a microbe’s growth and metabolism Maximum temperature – highest temperature that permits a microbe’s growth and metabolism Optimum temperature – promotes the fastest rate of growth and metabolism © McGraw Hill LLC. 63 Temperature Adaptation Groups Psychrophiles – optimum temperature below 15°C; capable of growth at 0°C Mesophiles – optimum temperature 20°-40°C; most human pathogens Thermophiles – optimum temperature greater than 45°C Access the text alternative for slide images. © McGraw Hill LLC. 64 Gas Requirements: Oxygen As oxygen is utilized it is transformed into several toxic products: Singlet oxygen (1O2), superoxide ion (O2−), peroxide (H2O2), and hydroxyl radicals ( OH) Most cells have developed enzymes that neutralize these chemicals: Superoxide dismutase, catalase If a microbe is not capable of dealing with toxic oxygen, it is forced to live in oxygen free habitats © McGraw Hill LLC. 65 Adaptations to Oxygen Requirement 1 Aerobe – utilizes oxygen and can detoxify it Obligate aerobe – cannot grow without oxygen Facultative anaerobe – utilizes oxygen but can also grow in its absence Microaerophile – requires only a small amount of oxygen © McGraw Hill LLC. 66 Adaptations to Oxygen Requirement 2 Anaerobe – does not utilize oxygen Obligate anaerobe – lacks the enzymes to detoxify oxygen so cannot survive in an oxygen environment Aerotolerant anaerobes – do not utilize oxygen but can survive and grow in its presence © McGraw Hill LLC. 67 Oxygen Requirement Determination Cultures with reducing media that contain an O2-removing chemical, such as thioglycollate, can help determine the O2 requirements of a microbe The relative position of growth of bacteria that differ in O2 requirements in such culture media provides some indication of their adaptations to O2 use Terese M. Barta, Ph.D. © McGraw Hill LLC. 68 Anaerobic Culture Techniques Growing strictly anaerobic bacteria usually requires special media, methods of incubation, and handling chambers that exclude O2 Most microbes require some CO2 in their metabolism Capnophile – grows best at higher CO2 tensions than normally present in the atmosphere © McGraw Hill LLC. 69 Effects of pH Majority of microorganisms grow at a pH between 6 and 8 Neutrophiles– grow within range of pH 5.5 to 8 Acidophiles – grow at extreme acid pH Alkalinophiles – grow at extreme alkaline pH © McGraw Hill LLC. 70 Osmotic Pressure Most microbes exist under hypotonic or isotonic conditions Osmophiles – require a high concentration of salt (halophile) Obligate halophiles grow optimally in solutions of 25% NaCl but require at least 9% NaCl (salt lakes, ponds…) Halobacterium, Halococcus Osmotolerant – do not require high concentration of solute Facultative halophiles remarkably resistant to salt Staphylococcus aureus © McGraw Hill LLC. 71 Other Environmental Factors Pressure Barophiles – can survive under extreme pressure and will rupture if exposed to normal atmospheric pressure Water Only dormant, dehydrated cell stages (i.e. spores and cysts) tolerate extreme drying because of the inactivity of their enzymes © McGraw Hill LLC. 72 Concept Check: (5) Chlamydomonas nivalis grows on Alaskan glaciers and it’s photosynthetic pigments give the snow a red crust. This organism would be best described as a A. Psychrophile B. Alkalinophile C. Microaerophile D. Osmotolerant E. Barophile (a and b): Image courtesy of Nozomu Takeuchi Access the text alternative for slide images. © McGraw Hill LLC. 73 Concept Check: (6) Chlamydomonas nivalis grows on Alaskan glaciers and it’s photosynthetic pigments give the snow a red crust. This organism would be best described as a A. Psychrophile B. Alkalinophile C. Microaerophile D. Osmotolerant E. Barophile Answer: A © McGraw Hill LLC. 74 Ecological Associations Among Microorganisms 1 Microbial Associations: Symbiotic Nonsymbiotic Symbiotic: Organisms live in close nutritional relationships; required by one or both members Mutualism: May or may not be obligatory, dependent; both members benefit Commensalism: The commensal benefits; other member not harmed Parasitism: Parasite is dependent and benefits; host harmed © McGraw Hill LLC. 75 Ecological Associations Among Microorganisms 2 Nonsymbiotic: Organisms are free-living; relationships not required for survival Syntrophy: Members cooperate and share nutrients Amensalism: Some members are inhibited or destroyed by others © McGraw Hill LLC. 76 Ecological Associations 1 Symbiosis Two organisms live together in a close partnership Mutualism –both members benefit Obligate Mutualism require each other to survive Termites rely on endosymbiotic Trychonympha for survival Nonobligate Mutualism can be separated and live apart Ciliophoran Euplotes and unicellular green algae © McGraw Hill LLC. 77 Ecological Associations 2 Commensalism – commensal benefits, other member neither harmed nor benefited Haemophilus and Staphylococcus Parasitism – parasite dependent and benefits; host is harmed Rickettsia and Chlamydia bacteria © McGraw Hill LLC. 78 Ecological Associations 3 The figure of “Symbiosis.” Obligate Mutualism : Organisms are so intimately associated that they require each other to survive. Root nodules (a1) have nitrogen-fixing endosymbiotic bacteria that supply the plant with usable nitrogen and provide a nurturing habitat for the bacteria (a2 inset). Termites (b1) rely on endosymbiotic Trichonympha (b2 inset) for survival. © McGraw Hill LLC. 79 Ecological Associations 4 The figure of “Symbiosis” continues on this slide. (a1): Source: Scott Bauer/USDA; (a2): Louisa Howard - Dartmouth Electron Microscope Facility (a1) Root nodules on a legume; (a2) Bradyhizobium bacteria inside a nodule (3,000x) Access the text alternative for slide images. © McGraw Hill LLC. 80 Ecological Associations 5 The figure of “Symbiosis” continues on this slide. (b1): jeridu/Getty Images; (b2): Science History Images/Alamy Stock Photo (b1) Termites would be able to digest wood if not for a variety of endosymbiotic protists (b2), like Trichonympha (6,500x). Access the text alternative for slide images. © McGraw Hill LLC. 81 Ecological Associations 6 The figure of “Symbiosis” continues on this slide. Nonobligate Mutualism: Organisms interact at the cellular level for mutual benefit, but they can be separated and live apart. The protozoan in (c) engulfs the algae but absorbs the nutrients they release and shelters them. (d) The plant supplies nutrients to the fungus and the fungus protects the plants against drying and insects. © McGraw Hill LLC. 82 Ecological Associations 7 The figure of “Symbiosis” continues on this slide. (c): Courtesy of Dr. Ralf Wagner (c) The ciliophoran Euplotes (800x) harbors unicellular green algae. Access the text alternative for slide images. © McGraw Hill LLC. 83 Ecological Associations 8 The figure of “Symbiosis” continues on this slide. (d): Source: Nick Hill/USDA (d) Fungal hyphae (blue filaments— 500x) growing in close contact with the cells of a grass leaf. Access the text alternative for slide images. © McGraw Hill LLC. 84 Ecological Associations 9 The figure of “Symbiosis” continues on this slide. Commensalism: The members have an unequal relationship. One partner is favored by the association and the other is not harmed or helped. (e) Tiny colonies of Haemophilus absorb required growth factors given off by Staphylococcus. (f1 and 2) Human commensals associated with the epidermis make a living off flakes and excretions, generally with neutral effects. © McGraw Hill LLC. 85 Ecological Associations 10 The figure of “Symbiosis” continues on this slide. (e): Kathy Park Talaro (e) Satellite Haemophilus colonies clustered around Staphylococcus (white line of growth). Access the text alternative for slide images. © McGraw Hill LLC. 86 Ecological Associations 11 The figure of “Symbiosis” continues on this slide. (f1): Source: Image courtesy of Dr. CSBR Prasad, Vindhya Clinic and Diagnostic Lab, India/ CDC-DPDx; (f2): Source: Janice Carr/CDC (f1) Demodex mite lives in or around human hair follicles. (100x) and (f2) Micrococcus luteus bacteria live on the skin surface (2,000x) Access the text alternative for slide images. © McGraw Hill LLC. 87 Ecological Associations 12 The figure of “Symbiosis” continues on this slide. Parasitism: A microbe invades the sterile regions of a host and occupies its tissues and cells, causing some degree of damage. (g) All viruses are parasites that invade cells and take over their function. (h) Malaria shows multilevel parasitism. Mosquitoes (h1) are blood-sucking ectoparasites of humans that carry their own parasites (h2) that can also infect humans. © McGraw Hill LLC. 88 Ecological Associations 13 The figure of “Symbiosis” continues on this slide. (g): Source: Cynthia Goldsmith/CDC (g) Sin Nombre hantavirus, a human pathogen carried in the waste of mice (5,000x). Access the text alternative for slide images. © McGraw Hill LLC. 89 Ecological Associations 14 The figure of “Symbiosis” continues on this slide. (h1): Source: James Gathany/CDC; (h2): Source: Blaine A. Mathison/CDC-DPDX (h1) The malaria vector, a female Anopheles mosquito; (h2) malaria parasites (Plasmodium, 1,000x) from blood Access the text alternative for slide images. © McGraw Hill LLC. 90 Ecological Associations 15 Non-symbiotic When organisms are free-living in a shared habitat Syntrophy (or cross-feeding)– Microbes sharing a habitat feed off substances released by other organism Azotobacter and Cellulomonas Amensalism – One member of an association produces a substance that harms or kills another (antagonism, competition) Antibiosis © McGraw Hill LLC. 91 Ecological Associations 16 The figure of “Non-symbiotic.” Syntrophy: Microbes sharing a habitat feed off substances released by other organisms, (i) Azotobacter releases NH4 that feeds Cellulomonas, and Cellulomonas degrades cellulose that feeds Azotobacter. (j) A dust mite lives in human settings and feeds off dead skin flakes. © McGraw Hill LLC. 92 Ecological Associations 17 The figure of “Non-symbiotic” continues on this slide. (i) Cycle of cross-feeding in two soil bacteria Access the text alternative for slide images. © McGraw Hill LLC. 93 Ecological Associations 18 The figure of “Non-symbiotic” continues on this slide. (j): MedicalRF.com (j) Dermatophagoides mite (100x) Access the text alternative for slide images. © McGraw Hill LLC. 94 Ecological Associations 19 The figure of “Non-symbiotic” continues on this slide. Amensalism: One member of an association produces a substance that harms or kills another. (k) Ants have complex symbiotic relationships that involve mutualism and amensalism with fungi and bacteria. (l1 and l2) In the amensal phase of their ecology, the ants cultivate actinomycetes to protect their habitat from microbial pests. © McGraw Hill LLC. 95 Ecological Associations 20 The figure of “Non-symbiotic” continues on this slide. (k): Paul Bertner/Getty Images (k) Leaf cutting ants gathering food for a fungus garden that is their source of food. Access the text alternative for slide images. © McGraw Hill LLC. 96 Ecological Associations 21 The figure of “Non-symbiotic” continues on this slide. (l1): Source: Jorg Barke, Ryan F Seipke, Sabine Grüschow, Darren Heavens, Nizar Drou, Mervyn J Bibb, Rebecca JM Goss, Douglas W Yu, and Matthew I Hutchings; (l2): Microfield Scientific Ltd/Science Source (l1) Antibodies by an actinomycete against a pathogenic fungus;(l2) micrograph of actinomycete (800x) Access the text alternative for slide images. © McGraw Hill LLC. 97 Other Microbial Relationships: Biofilms Biofilms result when organisms attach to a substrate by some form of extracellular matrix that binds them together in complex organized layers Dominate the structure of most natural environments on earth Communicate and cooperate in the formation and function of biofilms – quorum sensing © McGraw Hill LLC. 98 Biofilm Formation and Quorum Sensing 1 The figure of “Biofilm Formation.” Access the text alternative for slide images. © McGraw Hill LLC. 99 Biofilm Formation and Quorum Sensing 2 The figure of “Biofilm Formation” continues on this slide. 1. Free-swimming cells lose their motility and settle down onto a surface or substrate. 2. Cells synthesize an adhesive matrix that holds them tightly to the substrate. 3. When biofilm grows to a certain density (quorum), the cells release inducer molecules that can coordinate a response. 4. Enlargement of one cell to show genetic induction. Inducer molecule stimulates expression of a particular gene and synthesis of a protein product, such as an enzyme. 5. Cells secrete their enzymes in unison to digest food particles. © McGraw Hill LLC. 100 Interrelationships Between Microbes and Humans Human body is a rich habitat for symbiotic bacteria, fungi, and a few protozoa - normal microbial flora or microbiota Commensal, parasitic, and synergistic relationships Affect our physiology, immunity, and genetics © McGraw Hill LLC. 101 The Study of Microbial Growth Microbial growth occurs at two levels: growth at a cellular level with increase in size, and increase in population Division of bacterial cells occurs mainly through binary (or transverse) fission Parent cell enlarges, duplicates its chromosome, and forms a central transverse septum dividing the cell into two daughter cells © McGraw Hill LLC. 102 Binary Fission 1 The figure of “Binary Fission.” 1. A parent cell at the beginning of the cell cycle: What cannot be seen is the synthesis and activity gearing up for cell division. 2. Chromosome replication and cell enlargement: The parent cell duplicates the chromosome and synthesizes new structures that enlarge the cell in preparation for the daughter cells. 3. Chromosome division and septation: The chromosomes affix to the cytoskeleton and are separated into the forming cells. The cell lays down a septum that begins to wall off the new cells. Other components (ribosomes) are equally distributed to the developing cells. © McGraw Hill LLC. 103 Binary Fission 2 The figure of “Binary Fission” continues on this slide. 4. Completion of cell compartments: The septum is synthesized completely through the center, and the cell membrane patches itself so that there are two separate cell chambers. 5. End of cell division cycle: Daughter cells are now independent units. Some species will separate completely as shown here, while others will remain attached, forming chains or pairs, for example. © McGraw Hill LLC. 104 Binary Fission 3 The figure of “Binary Fission” continues on this slide. Access the text alternative for slide images. © McGraw Hill LLC. 105 Rate of Population Growth 1 Time required for a complete fission cycle is called the generation, or doubling time Each new fission cycle increases the population by a factor of 2 – exponential growth Generation times vary from minutes to days © McGraw Hill LLC. 106 Rate of Population Growth 2 Access the text alternative for slide images. © McGraw Hill LLC. 107 Rate of Population Growth 3 *Note that the left scale is logarithmic and the right scale is arithmetic Access the text alternative for slide images. © McGraw Hill LLC. 108 Rate of Population Growth 4 Equation for calculating population size over time: Nf = (Ni)2n Nƒ is total number of cells in the population Ni is starting number of cells Exponent n denotes generation time © McGraw Hill LLC. 109 Concept Check: (7) Escherichia coli has a doubling time of 20 minutes. If there are 5 cells at the beginning of the experiment, how many will there be in 3 hours? A. 215 B. 6,000 C. 712 D. 2560 © McGraw Hill LLC. 110 Concept Check: (8) Escherichia coli has a doubling time of 20 minutes. If there are 5 cells at the beginning of the experiment, how many will there be in 3 hours? A. 215 B. 6,000 C. 712 D. 2560 Answer: D © McGraw Hill LLC. 111 Determinants of Growth: Viable Plate Count * The culture is probably in lag period with no few cells added. Access the text alternative for slide images. © McGraw Hill LLC. 112 Stages in the Population Growth Curve 1 In laboratory studies, populations typically display a predictable pattern over time – growth curve Stages in the normal growth curve: 1. Lag phase – “flat” period of adjustment, enlargement; little growth 2. Exponential growth phase – a period of maximum growth when cells have adequate nutrients and a favorable environment 3. Stationary phase – rate of cell growth equals rate of cell death caused by depleted nutrients and O2, excretion of organic acids and pollutants 4. Death phase – as limiting factors intensify, cells die exponentially © McGraw Hill LLC. 113 Stages in the Population Growth Curve 2 Access the text alternative for slide images. © McGraw Hill LLC. 114 Methods of Analyzing Population Growth 1 Turbidometry – most simple Degree of cloudiness, turbidity, of the nutrient culture media reflects the relative population size (a): Kathy Park Talaro Access the text alternative for slide images. © McGraw Hill LLC. 115 Methods of Analyzing Population Growth 2 Enumeration of bacteria: Viable colony count Direct cell count – (manually or automated) counting the number of cells in a sample microscopically Access the text alternative for slide images. © McGraw Hill LLC. 116 Methods of Analyzing Population Growth 3 Counting cells in direct count can be automated by sensitive devices: Coulter counter Flow cytometer © McGraw Hill LLC. 117 Methods of Analyzing Population Growth 4 Access the text alternative for slide images. © McGraw Hill LLC. 118 Methods of Analyzing Population Growth 5 Access the text alternative for slide images. © McGraw Hill LLC. 119 End of main content Because learning changes everything. ® www.mheducation.com © McGraw Hill LLC. All rights reserved. No reproduction or distribution without the prior written consent of McGraw Hill LLC.