Quaternary Structure of Proteins PDF
Document Details
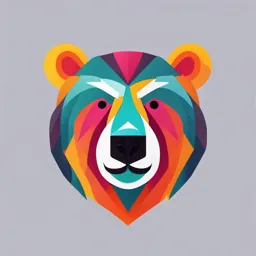
Uploaded by YouthfulGothicArt
Goodsell, D.S. and Olson, A.J.
Tags
Summary
This document explores the quaternary structure of proteins, a crucial aspect in biology. It explains the concepts of subunits, protomer, oligomers (monomer, dimer, trimer, etc.), and different types of symmetries involved (cyclic, dihedral, cubic). Examples and diagrams illustrate various types of protein assemblies and their interfaces, highlighting the importance of symmetry in protein function.
Full Transcript
Topic 04 Quaternary structure Quaternary structure Proteins that fold and operate as a single, isolated polypeptide chain are actually a minority Most proteins co-assemble with either multiple copies of the same protein type, or with other proteins, to form oligomers...
Topic 04 Quaternary structure Quaternary structure Proteins that fold and operate as a single, isolated polypeptide chain are actually a minority Most proteins co-assemble with either multiple copies of the same protein type, or with other proteins, to form oligomers This also allows modularity and flexibility – e.g. by having different paralogs or splice variants that occupy one position Terminology A single polypeptide subunit is known as a “protomer” A protein that operates as a single polypeptide chain is known as a “monomer” E.g. a “tetramer is comprised of four protomers” Examples: monomer (1), dimer (2), trimer (3), tetramer (4), pentamer (5), hexamer (6), octamer (8), dodecamer (12) Homooligomeric proteins are oligomers comprised of only one type of protein chain Heterooligomeric proteins are oligomers comprised of more than one type of protein chain (e.g. hemoglobin) The majority of proteins are oligomeric Survey of E. coli proteins homo- hetero- chains name oligomers oligomers percent Only ~20 % of E. coli 1 monomer 72 19.4 proteins are monomeric 2 dimer 115 27 38.2 3 trimer 15 5 5.4 Most proteins form small 4 tetramer 62 16 21 oligomers – dimers to 5 pentamer 1 1 0.1 6 hexamer 20 1 5.6 hexamers 7 heptamer 1 1 0.1 Even numbers of chains 8 octamer 3 6 2.4 9 nonamer 0 0 0 are much more common 10 decamer 1 0 0 than odd numbers 11 unadecamer 0 1 0 12 dodecamer 4 2 1.6 Note: eukaryotic proteins 13+ larger 8 2.2 ∞ polymers 2.7 are less homo-oligomeric Goodsell, D.S. and Olson, A.J. Annu. Rev.Biophys. Biomol. Struct. 29: 105-153 (2000) Examples of oligomeric proteins The protomer in each case is pink Note that oligomers are typically compact, with relatively little open space (but not always) Goodsell, D.S. and Olson, A.J. Annu. Rev.Biophys. Biomol. Struct. 29: 105-153 (2000) Building oligomeric interfaces To form an oligomer, proteins need to bind together with affinity significantly greater than their effective concentration This requires extended interaction surfaces that are complementary to one another Most oligomeric interfaces bury >1000 Å2, with 700 Å2 per interface being the bare minimum Typically, these surfaces co-evolve over extended time, and the oligomeric state of proteins tends to be fairly conserved The interfaces of transient oligomers (as seen in signaling) are typically only stable under certain contexts (e.g. when one partner is phosphorylated) The properties of oligomeric interfaces dUTPase 2hrm Interfaces are large and the shape of the interacting surfaces are complementary (so minimal holes, buried water) Oligomeric interactions are similar to interactions within a protein molecule, but are somewhat less hydrophobic Proteins may wrap around one another (as above), or have b- sheets that pair across the interface Typically, interaction surfaces are more conserved than typical solvent exposed protein surfaces Symmetry Symmetry is a property of an object such that that object can be transformed through space and will superimpose perfectly upon itself In effect, if you compare the object before and after a given shift, the shift is only a symmetry operation if the object is indistinguishable Symmetry operations have the property that if they can be applied once, then they can be applied over and over again (otherwise they would be distinguishable) Homo-oligomeric complexes are almost always symmetric Asymmetric homo-oligomers are exceptional, and generally only occur in functionally exceptional circumstances Rotational symmetry A rotation is a mapping that rotates each point around some fixed point in space If a pattern has rotational symmetry, it appears identical after some rotational shift Asymmetric unit The asymmetric unit is the smallest portion of a symmetric object which can be used to generate the whole object by symmetry operations For a protein oligomer, the asymmetric unit is at least one protein chain, but might be several chains (e.g. in a symmetric heterooligomer) Here, the asymmetric unit is 1/3th of one trimer, or one protein chain The complete object (here a trimer) can be generated by applying the symmetry operations to the asymmetric unit Rotational symmetry - cyclic Cyclic symmetries are rotational symmetries generated around a single axis They are designated Cn where n is the order of the symmetry E.g. C3 implies that three molecules are related by a rotation of 120º (360º/3) between each pair The larger the cyclic group the rarer Cyclic groups C2, C3 and C4 are common in proteins Proteins with cyclic symmetry Proteins can adopt a wide variety of cyclic symmetries Low order symmetries (C2 – C4) are much more common than higher order symmetries Larger rings are rare and generally only seen where the larger ring is needed for a certain function – e.g. Trap, which RNA will wrap around Rotational symmetry - dihedral Objects with dihedral symmetry are generated by pasting two Cn rings back-to-back There are two-fold symmetry axis between the rings E.g. D4 objects are built from two stacked C4 rings D symmetries are common and explain the preference for even numbers of subunits in oligomers As in C symmetries, smaller rings are more common Rotational symmetry - cubic Symmetries arise from multiple 2, asymmetric unit 3, 4 and 5-fold rotation axes intersecting at a common central point E.g. the icosahedron has – 5-fold symmetry axes passing through each vertex – 3-fold symmetry axis passing though the center of each facet – 2-fold symmetry axis passing through the middle of each edge An icosahedron is built from 60 copies of the asymmetric unit Icosahedron has 20 facets, each 3-fold symmetric For proteins, you need at least one protomer per a.u. Rotational symmetry - cubic T O I Name tetrahedron octahedron icosahedron Minimum numbers 12 24 60 of protomers Note – these are minimal numbers of protomers. In complex viruses, the asymmetric unit can be built from hundreds of protein chains Rotational C D I symmetry: Translational symmetry Translation is a mapping that shifts each point by some x, y and z If a pattern has translational symmetry, it appears identical after some translational shift Translational symmetry can be 1-, 2- or 3- dimensional This symmetry is strictly true only for infinite objects (e.g. crystals) For proteins, strict translational symmetry is rare. It most commonly manifests as local 2D symmetry in viral facets Screw/helical symmetry screw axis A screw operation combines a rotation around an axis, and a translation along the same axis Screw symmetry again produces objects which are (in principle) infinite in the direction of the screw axis Relatively few proteins use translational or screw symmetries Symmetry and symmetry breaking The norm in homo-oligomers is that they are symmetric Most oligomers break symmetry in trivial ways – different side chain conformers, small changes in the backbone These reflect the flexibility inherent to the protein – averaged over time, all protomers have the same mean conformation More rarely, symmetry can be significantly broken for functional reasons One example is half site reactivity, where different subunits of an enzyme take turns to react, with the other subunit(s) forced to be in a non-productive state Pseudosymmetry Pseudosymmetry refers to use of two or more structurally similar chains with distinct sequences within a given oligomer Hemoglobin, with a- and b-chains, is a classic example Some proteins are found as head to tail duplications of the same chain, where the two halves remain approximately symmetric Translational/helical pseudosymmetry with multiple fused domains Large proteins are sometimes built using multiple copies of a domain E.g. bacterial adhesins can have >100 identical copies of a small b-domain These bind each other head to tail, with helical symmetry Ca2+ stabilizes these interactions, allowing the protein to be secreted as a flexible chain, then lock This protein allows the bacteria to reach out and bind target surfaces (ice) Quasi-symmetry Quasi-symmetry occurs where chemically identical chains have distinct conformations. This concept is important in viruses, where a given protein can adopt different conformations in different structural contexts ATP synthase, where different protomers are in different structural states, is another example growth Quasi symmetry in hormone receptors hormone Human growth hormone is a small monomeric protein that circulates in the bloodstream It is detected by binding to the extracellular domain of the growth extracellular hormone receptor receptor domains Crucially, two receptors bind the hormone on opposite faces using different interactions membrane This promotes asymmetric dimerization of the intracellular domains, allowing one kinase to act intracellular as the substrate, the other as the signaling enzyme domains signaling events Domain-swapping as a route to facile oligomerization Domain-swapping occurs when a sub-domain from one protein structurally substitutes for the sub-domain from another copy of the same protein Since most interactions are exactly those in the monomer, the stability of the complex is similar to that of the monomer Domain swapping often involves the N- or C-termini It generally results in dimers, occasionally larger oligomers Domain swapping in wzt dimers b In wzt domain the last b-strand swaps to form a dimer The loop connecting this strand is a little too short to allow interaction within the domain Most of the interactions between monomers occurs through this b-strand, and the preceding loop Domain swapping is essential to the function of this protein (complex sugar export), and is conserved in distant homologs Immunoglobulin fold Complex cellular machines are often assembled as many chain hetero-oligomers Yeast RNA polymerase I has 14 unrelated subunits, each encoded by a separate gene Total MW: 590 kDa Building the protein this way allows it to be modular, with minor components being incorporated or swapped out as needed for specific steps or tasks Many complex cellular machines are built in this way, especially in Yeast RNA pol I (4c3h) eukaryotes Protein N-glycosyltransferase Protein N-glycosyltransferases co-translationally glycosylates select Asn residues in secreted eukaryotic proteins It is comprised of up to 9 ER lumen distinct protein chains A three protein sub-module can be swapped out for an alternative module that uses ER membrane homologous proteins The resulting complexes select different target sites to modify Oligomeric state is often tied to function Advantages of being monomeric Monomers remain stable at very low concentrations where oligomers would tend to dissociate - important e.g. if the protein is secreted Monomers have a higher amount of unique surface area available for binding Monomers can bind to any oligomeric complex without risking aggregation For the above two reasons, molecules which function in signaling by binding other proteins tend to be monomeric Monomers are smaller and diffuse faster Small, fast-diffusing carrier proteins are monomeric Monomers are more compact and therefore preferred for roles where fast diffusion is required e.g. plastocyanin has a redox copper ion It carries electrons from photosystem I to plastocyanin photosystem II Acyl carrier protein is covalently attached (via a phosphopanteinyl group) to a growing fatty acid molecule This solubilizes the hydrophobic cargo It carries its cargo from enzyme to enzyme, where the fatty acid inserts into acyl carrier protein the active site to allow reaction Advantages of being oligomeric Oligomers allow allosteric regulation mechanisms that utilize shifts in the oligomeric interface (e.g. hemoglobin, ATCase) Oligomeric proteins can present multimeric binding surfaces allowing extreme avidity Large oligomers can span large spaces (e.g. fibers) or enclose large volumes (virus shells, clathrin) Associating successive enzymes in a pathway can help promote efficient coupling of reactions Hetero-oligomeric interactions allow the assembly of large multi- protein complexes, and is the preferred strategy for building complex cellular machines These can also allow mix and match strategies for generating many variations of a complex with different properties (e.g. transcription regulation complexes) Avidity Affinity measures the binding energy released when a single binding site engages a single ligand If a protein has multiple binding sites, and they can all engage a given polymeric ligand simultaneously, then binding energies are added For this to work, the individual ligand targets need to be physically linked together This results in a binding avidity that is much more powerful than the affinity of the individual components Oligomeric proteins can therefore bind very tightly to polymeric epitopes Oligomerization can build filaments Cells build a variety of long filaments These provide pathways for trafficking, give the cell shape and motility, allow cell division etc. Filaments are built from small protein subunits that assemble via helical symmetry Using several distinct, relatively weak oligomeric interactions allows the overall filament to be very stable, but sub-units can readily be dissociated from the ends as needed Filament assembly and disassembly is often controlled by hydrolyzation of bound nucleotides, and a diverse set of fiber/subunit binding accessory proteins E.g. Actin is a major filament forming protein that is found in all domains of life Actin organization of the protomer (-) end of strand cleft Four domains (blue, yellow, orange, green) ATP Mg2+ groove Actin is related to (+) end of strand hexokinase! The actin filament (-) Actin protomers each interact with four 13 protomers, 36 nm helical repeat other subunits Each successive subunit is rotated green -> magenta 166° rotation almost 180o, and 28 Å translation shifted up half a protomer length The resulting filament has a double-helical appearance with a left-handed twist (+) F-Actin filament appears to be a double helix but can be described by simple helical symmetry a Tryptophan synthase Tryptophan synthase catalyses the last two steps of tryptophan synthesis b It was originally thought that Trp synthase catalyzes a simple, one step reaction Trp synthase is an a2b2 heterotetramer The a-enzyme cleaves indole from b indole-3-glycerol phosphate, releasing D- glyceraldehyde-3-phosphate The b-enzyme adds the indole to serine to make tryptophan, and has a very high a Km for indole 2TRS Substrate channeling Substrate channeling involves the passing of substrate molecules from one active substrate site to another without release into enters solution In Trp synthase, the a and b active sites are connected by a 25 Å tunnel a enzyme product active site The intermediate, indole is transferred exits directly between the catalytic sites 25 Å without release into solvent connecting tunnel This prevents the escape of indole, greatly increases its effective concentration of at b enzyme the second site active site Pathways with highly reactive intermediates (e.g. aldehydes) can also use channeling to prevent intermediates from reacting with inappropriate molecules C6 hexamer of Large rings can create open spaces spheres leaves hole the size of one sphere Proteins are typically globular Unless the protomers are wedge shaped, rings tend to HslV protomers are leave open spaces in their arranged to center maximize the interior open The more protomers, the space larger the hole tends to be The size of the space can be controlled by the shape of the CcmK protomers protomers, and the way they are wedge interact shaped, and arranged to make a small pore Protected interior spaces A ring of molecules can be organized so as to contain a central hollow space that is then protected from the larger environment Some chaperone proteins (e.g. GroEL/ES) envelop their cargo to protect it from other proteins as it refolds Most cytoplasmic proteases form large double rings with the catalytic sites lining an interior lumen Target proteins are unfolded by a chaperone helper, and threaded into the lumen, where they are degraded This organization allows proteins to be completely degraded, if required, without constantly accidentally damaging needed proteins Proteasome Gate – opens the entrance, and receives unfolded proteins through a small pore; there are three distinct complexes that do this, this one is hetero- trimeric 7-membered a-subunit rings form a vestibule Back-to-back 7-membered b-subunit rings form the proteolytic chamber Sadre-Bazzar et al 2010, Mol Cell The proteasome is the main intracellular protease in eukaryotes a- and b- subunits are each made of seven distinct paralogs and exhibit pseudosymmetry repeat unit DNA sliding clamp The DNA sliding clamp envelops dsDNA, allowing movement along DNA but unable to let go until unloaded by specialized proteins E coli DNA clamp with DNA bound – 3BEP The clamp keeps DNA polymerase clamped onto DNA and is essential for precessive DNA replication The E. coli clamp is dimeric Each protomer has three copies of a basic repeat (pseudosymmetry) The human protein is trimeric, with 2 pseudo-equivalent copies H sapiens DNA clamp with DNA polymerase fragment– 2ZVM Encapsulation Encapsulation is the enclosing of some molecular content in a protein shell Encapsulin is a 31 kDa archaeal protein with structural similarity to virus capsid proteins 60 copies assemble into an icosahedral structure 240 nm in diameter An enzyme can be recruited to the lumen via a C-terminal sequence that is recognized by encapsulin. One such targeted enzyme is ferritin; this can reduce iron which can then be stored in the large interior space Sutter et al Nature Stuct Mol Biol, 2008; 3DKT Bacterial microcompartments Found in diverse bacteria (~20 %) with 15+ different varieties Icosahedral particles built from three different protein families (with multiple paralogs of each contributing) This example is 40 nm; up to 600 nM Encapsulate multiple enzymes within Volatile or toxic reaction intermediates generated by one enzyme, then fixed by another without escaping Important for cyanobacterial carbon fixation, and ethanolamine, choline, fucose, propanediol breakdown Sutter, Science, 2017 Vault nucleoprotein complex The liver vault is a large nucleoprotein complex found in the liver Its function is presently unclear; seems to play a role in viral immunity It is built primarily from a 99 kDa major protein (shown) The protein is comprised of – 9 head to tail fusions of a repeated domain – a shoulder domain (green) – a long helical cap domain (blue) – a cap ring domain (dark red) Vault Use of repeated domains in the monomer, in many copies, allows the building of a highly unusual vertically elongated, cylindrically symmetric structure The vault is built as two 39 member rings binding back-to-back (D39 symmetry) The interior is almost entirely closed off from outside vRNA and two additional proteins are present, but not modeled Gas vesicles – modified helical symmetry Gas vesicles are large (~250 nm long) protein bodies made by diverse bacteria They are built as multi-protein complexes with a thin protein wall and a hollow interior They vary in size, but are all organized as cylinders with conical end caps Gas vesicle Gas vesicles are made from many copies of a small protein GvpA with 2 b-strands and 2 structure terminal a-helices The b-sheets pair with identical copies to form an extended ribbon exterior face The ribbons spirals around, forming a cylindrical body and conical end cap Each ribbon interacts with the adjacent layer via the helices and N-extension interior face Two such assemblies dimerize to form the vesicle Huber et al, Cell, 2023 Hydrophobicity in action – gas vesicles The GsvA amino acids that line the interior are strongly hydrophobic These interact unfavourably with water Gasses can enter via small pores between Serratia sp. the helices, and are concentrated by the hydrophobic effect Gas vesicles self-inflate after assembling, becoming buoyant Their buoyancy allows the microscopic host organism to migrate 10s of meters vertically Light shade arises from absence of electron scattering water within the vesicle through the water column with minimal effort Symmetry allows viruses to encode their shell The viral shell needs to encapsulate the DNA (or RNA) that encodes all viral proteins, including the shell proteins One shell protein can only enclose a limited amount of DNA It takes significantly more DNA to encode a shell protein than that shell protein could cover as a monomer (if only because nucleotides are bigger than amino acids) Therefore, the only way to make a protein shell that can enclose its encoding DNA is to use multiple copies of one or a few proteins to assemble a highly symmetric shell This argument was first pointed out by Watson and Crick in 1956, before any virus structures were known (1956. Nature 177,473-475) Helical viruses - Tobacco mosaic virus One way to build an ~40 Å encapsulating shell is as a hollow helical tube Tobacco mosaic virus is a helical virus Made from a single 158 a.a. 4- helix bundle coat protein ~3000 Å Helix has 161/3 subunits/turn Rises 1.4 Å per subunit Net result is a long thin hollow tube that envelops the TMV RNA genome (red) ~180 Å http://www.rcsb.org/pdb/static.do?p=education_discussion/molecule_of_the_month/pdb109_1.html Icosahedral viruses An icosahedral shell can also be used to encapsulate DNA/RNA Spherical viruses generally adopt icosahedral symmetry The shell is often made from more than one type of protein, and can be multi-layered Often specialized structures are built at the 5-fold symmetric vertices David Goodsell – pdb molecule of the month pentamers at Tiling in viral facets 5-fold axis Many viruses have distinct proteins forming the vertices and the facets In some viruses, the facet is formed (pseudo-) from multiple copies of a hexameric hexamers tile to form facets protein tiled in 2D Adenovirus In adenovirus, each facet is made from 12 pseudo-hexamers 240 copies of this protein per virus Positions 1-4 are in non-equivalent environments, and interact with their neighbours in different ways (and are therefore quasi-symmetric) Higher and lower tiling numbers also Reddy et al Science 2010 occur Icosahedral virus diversity among the 200 structures in the PDB