CAIE Physics IGCSE Electricity and Magnetism Summary Notes PDF
Document Details
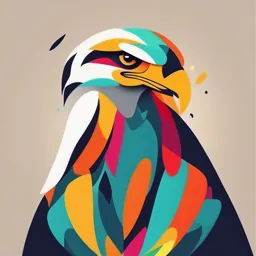
Uploaded by GodlikePentagon4392
CAIE
Tags
Summary
This document provides summary notes on electricity and magnetism for CAIE Physics IGCSE students. The notes cover topics like simple phenomena of magnetism, magnetic materials, and magnetic fields.
Full Transcript
CAIE Physics IGCSE Topic 4: Electricity and Magnetism Summary Notes Definitions in bold are for extended students only This work by PMT Education is licensed under https://bit.ly/pmt-cc https://bit.ly/pmt-edu-cc CC BY-NC-ND 4.0...
CAIE Physics IGCSE Topic 4: Electricity and Magnetism Summary Notes Definitions in bold are for extended students only This work by PMT Education is licensed under https://bit.ly/pmt-cc https://bit.ly/pmt-edu-cc CC BY-NC-ND 4.0 https://bit.ly/pmt-cc https://bit.ly/pmt-edu https://bit.ly/pmt-cc 4.1 Simple phenomena of magnetism A magnet is a material or object that produces a magnetic field. Magnetic forces arise from interactions between these magnetic fields. A magnet has a pole at either end: a north (N pole) and a south (S pole). The force between magnetic poles depends on which poles are facing one another: ○ Like poles experience repulsion. ○ Opposite poles experience attraction. Non-magnetic materials are materials that are not attracted to magnets. These include glass and plastic. There is no force between a magnet and a non-magnetic material. Magnetic materials are materials that are attracted to magnets. These include iron, steel, cobalt, and nickel. The force between a magnet and magnetic material is always attractive, regardless of the pole the material is placed at and whether it is unmagnetised or magnetised. Magnetic materials can be magnetised (given the properties of a magnet) by induced magnetism: The material is placed in a magnetic field using one of the following methods: ○ Stroking them with a magnet ○ Hammering them in a magnetic field ○ Placing them in a coil with a direct current through it One end of the material becomes a north pole, the other becomes a south pole. ○ Some become permanently magnetised (steel) from this process. ○ Others can only become temporary (induced) magnets (soft iron). EXAMPLE: When soft iron is placed within coiled wire with a direct current running through it, this makes a common temporary magnet known as an electromagnet or a transformer. https://bit.ly/pmt-cc https://bit.ly/pmt-edu https://bit.ly/pmt-cc There are differences in the properties of temporary magnets and permanent magnets: Temporary magnets lose magnetism when the magnetic field is removed. ○ Permanent magnets do not, because they make their own magnetic field. Therefore, temporary magnets can be turned on and off. ○ Permanent magnets cannot. Temporary magnets, such as electromagnets, are used for things we turn on and off, such as electric motors and moving scrap metal. ○ Permanent magnets are used for things that always need to be on, such as fridges and compasses. Magnets are surrounded by a magnetic field - a region in which a magnetic pole experiences the force from another magnet or magnetic material. A magnetic diagram can be drawn to show the strength and direction of a magnetic field. ○ Magnetic field lines are drawn around bar magnets. ○ The direction of the magnetic field at a point (the direction of the force on the north pole of a magnet placed in the magnetic field at that point) is shown using arrows on the field lines (that must point from the north to south pole). ○ Magnetic field strength is shown by how close field lines are to one another (closer together = stronger). ○ The magnetic field is stronger at the poles and decreases with distance away from them. There are two methods which allow you to visualise and plot magnetic field lines.: With a plotting compass: Place a magnet on top of paper. Draw around the magnet on the paper Put a plotting compass next to a point around the magnet’s edge, with its needle pointing towards this point Draw a dot at the other side of the compass needle. Move the compass so that it points towards the new dot, and draw a dot on the other side of the compass needle again Repeat to form a dotted line connecting one end of the magnet to the other. Linking the dots gives the magnetic field line. The direction of the field line is the same as the direction of the plotting compass Repeat this whole process, starting at a different point around the magnets edge to draw another field line. With iron filings: Place paper on top of a magnet Sprinkle iron filings onto the paper Tap the paper and the filings will settle on the field lines https://bit.ly/pmt-cc https://bit.ly/pmt-edu https://bit.ly/pmt-cc 4.2 Electrical quantities 4.2.1 Electric charge Electric charge is measured in coulombs and can be positive or negative. Opposite charges experience an attractive force. Like charges experience a repulsive force. An electric field is the region in which an electric charge experiences these attractive/repulsive forces. Conductors are materials which allow electric charge to flow through them because they have charged particles that are free to move. Examples include: metals (which have free electrons to act as charge carriers) and molten ionic substances (which have ions that can act as charge carriers). Insulators are materials which impede the flow of electric charge because they don’t have charged particles that are free to move. Examples include: non-metals, such as rubber, plastic, glass, and wood. When charged, insulators gain electrostatic charge (electric charge at rest on their surface). Objects can be given a charge (charged). This involves the transfer of negative charge alone (electrons) by their addition or removal. Insulators can be electrostatically charged by friction using a simple experiment: Two insulators are rubbed together (a cloth used to rub one end of a rod). Electrons move from one to the other (electrons are transferred from the rod onto the cloth) Each become oppositely charged (the rod’s end becomes positively charged; the cloth becomes negatively charged). The electrostatic charges produced can then be detected by extending this simple experiment: Charge a second rod of insulating material using friction. Hold the charged ends of the second and first rod together. If a force is experienced, it shows both rods were electrostatically charged. ○ If they repel one another, they have the same electrostatic charge ○ If they attract one another, they have opposite electrostatic charges. Conductors and insulators can be distinguished between using a Gold-leaf electroscope (GLE): Charge the GLE plate. https://bit.ly/pmt-cc https://bit.ly/pmt-edu https://bit.ly/pmt-cc This gives the plate, rod and gold leaf the same charge so they repel, and the leaf sticks out to the side. Touch the material being investigated to the plate Record the observations ○ If the leaf falls: material is a good conductor. ○ If the leaf remains in place: object is a good insulator. The electric fields around all charged objects can be drawn using field lines, similarly to magnetic fields. The direction of the electric field at a point (the direction of the force on a positive charge placed in the magnetic field at that point) is shown using arrows on the field lines (that must point away from positive charges and towards negative charges). Electric field strength is shown by how close field lines are to one another (closer together = stronger). ○ Electric field strength is stronger closer to the charged object and decreasess with distance away from it. To draw the electric field around a point charge: The field lines for a positive charge point radially outwards. The field lines for a negative charge point radially inwards. (This naturally leaves lines closer to one another, nearer the point charge). https://bit.ly/pmt-cc https://bit.ly/pmt-edu https://bit.ly/pmt-cc To draw the electric field around a charged conducting sphere: It can be drawn like that of a point charge, as if the charge is concentrated as a point charge at the centre of the sphere. To draw the electric field between two oppositely charged conducting plates: Straight lines from the positive plate to the negative plate. Equally spaced apart. 4.2.2 Electric current An electric current is related to the flow of charge. It is the charge passing a point in the circuit per unit time: 𝑐ℎ𝑎𝑟𝑔𝑒 𝑄 𝐶𝑢𝑟𝑟𝑒𝑛𝑡 = 𝑡𝑖𝑚𝑒 𝐼 = 𝑡 𝑢𝑛𝑖𝑡𝑠 = 𝑎𝑚𝑝𝑠 (A) Conventional current is defined as the rate of flow of positive charge (from a positive to a negative terminal). Electric currents are measured with a digital or analogue ammeter. The ammeter is connected in series (placed one after the other in a continuous line) with the part of the circuit you are measuring the current through. They must be set to zero before use. Different ammeters measure current over a different range. ○ Analogue ammeters typically measure currents over a larger range (0.1 - 5 amps). ○ Digital ammeters can measure currents over a much smaller range (milliamps or microamps), so are more precise. https://bit.ly/pmt-cc https://bit.ly/pmt-edu https://bit.ly/pmt-cc ○ The ammeter used for a specific circuit should result in a reading in the middle of its range, to avoid its damage.. Metals can have an electric current flow through them because their electrons are free to carry charge through the material (electrical conduction). A metal’s current is the opposite of a conventional current because negative charges (free electrons) flow from negative to positive. An electric current flows either as a direct current (d.c.) or an alternating current (a.c.). d.c. electrons flow in one direction, from the negative terminal to the positive terminal. a.c. electrons change direction of flow regularly (so do not have a positive and negative terminal). https://bit.ly/pmt-cc https://bit.ly/pmt-edu https://bit.ly/pmt-cc 4.2.3 Electromotive force and potential difference To move charge around an electric circuit, electrical energy is transferred to the charged particles (e.g. electrons) each time they pass through the energy source (e.g. battery). The electromotive force (e.m.f) of an electrical energy source is the electrical work done by a source in moving a unit charge around a complete circuit. E.m.f. is measured in volts (V). E.m.f. can be found using the equation: 𝑐ℎ𝑎𝑟𝑔𝑒 𝑊 𝑒. 𝑚. 𝑓 = 𝑡𝑖𝑚𝑒 𝐸 = 𝑄 𝑢𝑛𝑖𝑡𝑠 = volts When the charged particles pass through a circuit component, work is done to transfer some of their electrical energy to another form (e.g. light energy is the component is a bulb). Potential difference (p.d.) shows the work done by a unit charge passing through a component (by measuring the difference in energy across the component). P.d. is also measured in volts (V) because the p.d. of an electrical energy source is its e.m.f. P.d. can be found using the equation: 𝑐ℎ𝑎𝑟𝑔𝑒 𝑊 𝑝. 𝑑. = 𝑡𝑖𝑚𝑒 𝑉 = 𝑄 𝑢𝑛𝑖𝑡𝑠 = 𝑣𝑜𝑙𝑡𝑠 P.d. is measured with a digital or analogue voltmeter. The voltmeter is placed in parallel with the component being investigated (on a parallel branch to the component, connected either side of it). The higher the voltage, the higher the p.d. and the greater the current. Digital and analogue voltmeters differ in the same way as ammeters: ○ Analogue voltmeters measure p.d. over a larger range (0 - 5.0 V). ○ Digital voltmeters measure p.d. over a smaller range (millivolts or microvolts), so are more precise. https://bit.ly/pmt-cc https://bit.ly/pmt-edu https://bit.ly/pmt-cc 4.2.4 Resistance The resistance of a circuit component shows its opposition to the current flowing through it. The greater the resistance, the harder it is for current to flow through. Resistance is related to potential difference, so is found using the equation: 𝑝𝑜𝑡𝑒𝑛𝑡𝑖𝑎𝑙 𝑑𝑖𝑓𝑓𝑒𝑟𝑒𝑛𝑐𝑒 𝑉 𝑅𝑒𝑠𝑖𝑠𝑡𝑎𝑛𝑐𝑒 = 𝑐𝑢𝑟𝑟𝑒𝑛𝑡 𝑅 = 𝐼 Current-voltage graphs show how current and voltage are related: For a resistor with constant resistance - the current is directly proportional to the voltage. For a resistor with varying resistance (e.g. a filament lamp) - voltage and current are not directly proportional. As the current increases through a filament lamp, so does the temperature. This means electrons and ions vibrate more and collide more, increasing resistance. For a diode - current only flows in one direction and after a particular voltage has been reached. An experiment can be done to find the resistance of a circuit component: Connect a length of wire in a circuit with a power supply, a voltmeter in parallel, and an ammeter in series with the component being investigated. Record the current on the ammeter and voltage on the voltmeter. Use the current and voltage to calculate the resistance with the equation: 𝑅=𝑉/𝐼. Repeat three times, remove anomalies, and calculate a mean. Repeat for resistors with different properties to investigate the factors affecting resistance (e.g. by varying length of the wire using crocodile clips). The resistance of the metal wire in a circuit changes as its properties change: As its length increases, the resistance increases. ○ Resistance is directly proportional to the resistor’s length. As its cross-sectional area increases, resistance decreases. ○ Resistance is inversely proportional to the cross-sectional area. https://bit.ly/pmt-cc https://bit.ly/pmt-edu https://bit.ly/pmt-cc 4.2.5 Electrical energy and electrical power In an electric circuit, the electrical energy source (electrical cell or mains supply) transfers its energy to electrical energy. This electrical energy is used by the circuit components to do work. The remaining energy is released to the surroundings (usually as heat / sound energy). The electrical power of a component is the rate at which it transfers electrical energy. It can be calculated using the equation: 𝑃𝑜𝑤𝑒𝑟 = 𝑐𝑢𝑟𝑟𝑒𝑛𝑡 𝑥 𝑣𝑜𝑙𝑡𝑎𝑔𝑒 𝑃 = 𝐼𝑉 The electrical energy transferred to an appliance depends on its power and the length of time it is switched on. It can be calculated by multiplying electrical power by time: 𝐸𝑙𝑒𝑐𝑡𝑟𝑖𝑐𝑎𝑙 𝑒𝑛𝑒𝑟𝑔𝑦 = 𝑝𝑜𝑤𝑒𝑟 𝑥 𝑡𝑖𝑚𝑒 = 𝑐𝑢𝑟𝑟𝑒𝑛𝑡 𝑥 𝑣𝑜𝑙𝑡𝑎𝑔𝑒 𝑥 𝑡𝑖𝑚𝑒 𝐸 = 𝐼𝑉𝑡 𝑢𝑛𝑖𝑡 = 𝑘𝑊ℎ A kilowatt-hour (kWh) is a unit of electrical energy. ○ It shows the electrical energy converted by a 1 kW appliance used for 1 hour. ○ This measure is used to calculate the cost of using electrical appliances. 𝐶𝑜𝑠𝑡 = 𝑒𝑙𝑒𝑐𝑡𝑟𝑖𝑐𝑎𝑙 𝑒𝑛𝑒𝑟𝑔𝑦 𝑢𝑠𝑒𝑑 (𝑘𝑊ℎ) 𝑥 𝑐𝑜𝑠𝑡 𝑝𝑒𝑟 𝑘𝑊ℎ https://bit.ly/pmt-cc https://bit.ly/pmt-edu https://bit.ly/pmt-cc 4.3 Electric circuits 4.3.1 Circuit diagrams and circuit components Circuit diagrams are a drawn representation of an electric circuit. When drawn, there should be no breaks in the complete circuit. Cables and wires are drawn as straight lines that do not cross over. Different symbols are used to show different components: https://bit.ly/pmt-cc https://bit.ly/pmt-edu https://bit.ly/pmt-cc ○ Cells / batteries (multiple cells connected) - the circuit’s source of electrical energy. ○ Generators - electrical energy source creating electrical energy from mechanical. ○ Power supplies - convert electric energy from a source, to a current with the right voltage. ○ Potential dividers - divide the source voltage into smaller parts to vary the voltage, using resistors. ○ Switches - used to turn a circuit on (closed) and off (open). ○ Relays - an electromagnetically operated switch, used to switch on a circuit with a high current by using a circuit with a small current. ○ Resistors - restrict or limit the flow of electrical current. A fixed resistor’s resistance does not change, a variable’s one does. ○ NTC thermistors - resistors whose resistance depends on temperature (resistance decreases as temperature increases). ○ Light-dependent resistors (LDRs) - resistors whose resistance depends on light intensity (resistance decreases as light intensity increases). ○ Diodes and light-emitting diodes (LEDs) - allow current to flow in only one direction by having high resistance to flow in the opposite direction (can convert a.c. into d.c.). ○ Lamps - convert the electric current into light using a filament, which is heated by the current. ○ Heaters - convert the electric current into heat. ○ Motors - convert electrical energy from the circuit into mechanical energy using the magnetic effect of a current. ○ Ammeters - placed in series and measure the electric current in amps. ○ Voltmeters - placed in parallel and measure the potential difference across a circuit component. ○ Magnetising coils - store energy in a magnetic field when current flows through them. ○ Transformers - change the magnitude of an alternating voltage. ○ Fuses - safety devices that cut off the power supply if there is a surge in current. https://bit.ly/pmt-cc https://bit.ly/pmt-edu https://bit.ly/pmt-cc 4.3.2 Series and parallel circuits Series: Components are connected end to end in one continuous loop The current at every point in a series circuit is the same. The potential difference of the power supply is shared across each component according to their resistances (i.e. the total p.d. across the components in a series circuit is equal to the sum of the individual p.d.s across each component). The combined resistance is the sum of the resistances of each component in series. RT = R1 + R2 The combined e.m.f. of several sources in series is the sum of the individual e.m.f.s. Parallel: Components are connected to the power supply in separate branches that current can flow through independently. The current is shared between each branch (i.e. the sum of the currents in the separate branches is equal to the current through the source) so the current in the source is larger than the current in each branch. ○ The sum of the currents entering a junction in a parallel circuit is equal to the sum of the currents that leave the junction. The potential difference is the same across every branch in the circuit and is equal to the potential difference of the supply. The combined resistance of two resistors in parallel is less than the resistance of either resistor by itself. This is because the charge is split; decreasing the charge to each component decreases its resistance. ○ Combined resistance is given by: 1 1 1 1 1 1 𝑐𝑜𝑚𝑏𝑖𝑛𝑒𝑑 𝑟𝑒𝑠𝑖𝑠𝑡𝑎𝑛𝑐𝑒 = 𝑟𝑒𝑠𝑖𝑠𝑡𝑎𝑛𝑐𝑒 𝑜𝑛𝑒 + 𝑟𝑒𝑠𝑖𝑠𝑡𝑎𝑛𝑐𝑒 2 𝑅𝑇 = 𝑅1 + 𝑅2 Connecting lamps in parallel is advantageous because if one breaks, current can still pass through the rest. 4.3.3 Action and use of circuit components When resistors are connected in a series, the potential difference of the power source is shared between them. A variable potential divider is a circuit that uses resistors to divide the source voltage into smaller parts to vary the voltage (e.g. to control temperature of an appliance). If the current is constant, then the potential difference across an electrical conductor increases as its resistance increases. ○ Therefore, the potential difference is shared between resistors in a ratio determined by their resistances. The resistor with the larger resistance has the larger potential difference: 𝑅1 𝑉1 𝑅2 = 𝑉2 https://bit.ly/pmt-cc https://bit.ly/pmt-edu https://bit.ly/pmt-cc 4.4 Electrical Safety Hazards of electric circuits: Damaged insulation – contact with the wire due to gaps in the insulation can cause an electric shock or pose a fire hazard by creating a short circuit. Overheating of cables – high currents passing through thin wire conductors cause the wires to heat up to very high temperatures which could melt the insulation so poses a fire hazard. Damp conditions – water can conduct currents, so wet equipment can cause an electric shock. Overloading plugs, extension leads, single and multiple sockets when using a mains supply – creates excess current which produces heat and so poses a fire hazard. A mains circuit is an electric circuit, connected to the power grid for its electrical supply. These circuits consist of: ○ A live wire (line wire) - the terminal of the circuit which delivers the current. ○ A neutral wire - the terminal which carries the current back to the power source. ○ An earth wire - protects from sudden voltage fluctuations. The mains circuits have a switch connected to the live wire, so that when it is switched off, the flow of current is switched off and the circuit is safe (if it were connected to the neutral wire, the current would still flow). The mains circuits also have safety devices which act to stop the flow of current if it becomes too high: A fuse - a thin piece of wire which overheats and melts if the current is too high, breaking the circuit, so stopping the flow of current. A trip switch - has the same function but is an automatically operated switch which flicks off when the current is too high (so can be reset and used again). Fuses have a rating and trip switches have a setting, which reflects the current they will withstand before breaking/switching off the circuit. ○ These should be only slightly higher than the current used by the device in the circuit. The most common fuse ratings are 3A, 5A and 13A. https://bit.ly/pmt-cc https://bit.ly/pmt-edu https://bit.ly/pmt-cc To reduce the risk of electrocution from wires in the mains circuit, they are covered in an outer casing, which can be either non-conducting (double-insulated) or earthed. Non-conducting casing is a layer of insulation around the wires which is non-metallic. Earthed casings are metal but connected to an earth wire. ○ Earth wires create a safe route (rather than through a person) for current to flow through in the case of a short circuit (when the wire is touched). ○ They have a very low resistance, so the current surges through them with more strength, which breaks the fuse and disconnects the appliance. A fuse without an earth wire protects the circuit and the cabling for a double-insulated appliance. 4.5 Electromagnetic effects 4.5.1 Electromagnetic induction Electromagnetic induction is the creation of an e.m.f. using a magnetic field either by: A wire moving across a magnetic field, creating current flow. ○ The induced e.m.f. 's magnitude can be increased by moving the wire more quickly, using a stronger magnetic field, or increasing the length of the wire. Changing the direction of a magnetic field through a coil, creating current flow. ○ The induced e.m.f. 's magnitude can be increased by changing the magnetic field more quickly. The current induced flows in such a direction that it opposes the change that produced it. The direction of the induced current is determined by Fleming’s right hand rule (induced current, magnetic field, and wire movement directions are at right angles to one another, along each axis). Electromagnetic induction can be demonstrated experimentally: By moving a magnet through a wire coil: ○ A wire coil is connected to a voltmeter. ○ A bar magnet is moved in and out of the coil. ○ An e.m.f can be read on the voltmeter ○ Length of the coil, speed of magnet movement, and magnet strength can be altered to investigate the effect of these factors. By changing the direction of the magnetic field a wire coil is in: ○ A wire is connected to a voltmeter and moved between two magnets. ○ The e.m.f. is read on the voltmeter. ○ Speed of the change in magnetic field can be altered to investigate this factor. https://bit.ly/pmt-cc https://bit.ly/pmt-edu https://bit.ly/pmt-cc Results for both should be zero when not moving, increasing with speed of movement, and a brief value with the opposite sign when moving out of the coil/ magnetic field. 4.5.2 The a.c. generator An a.c. generator uses electromagnetic induction to create an a.c. current from mechanical energy. It consists of a coil of wire between two permanent magnets, spun by a turbine (see Topic 6). This generates a.c. current because a slip ring is used. ○ As the coil rotates, the magnetic field through the coil changes, which induces an e.m.f.. ○ Slip rings keep a continuous connection with the wire to rotate it, allowing an alternating current to be produced because every half rotation the current changes direction (to flow from the south to north pole). ○ Carbon brushes connect the slip ring to the circuit, transmitting the current. The e.m.f. can be plotted against time and each point in the graph relates to a positioning of the wire coil: The magnitude of the e.m.f. is at its maximum when the coil is horizontal (at 90° and 270° perpendicular to the direction of the magnetic field) as the field lines are cut the fastest. The magnitude of the e.m.f. is zero when the coil is vertical (at 0° and 180°, in line with the magnetic field) so no field lines are being cut. https://bit.ly/pmt-cc https://bit.ly/pmt-edu https://bit.ly/pmt-cc 4.5.3 Magnetic effect of a current When a charged particle moves it causes a magnetic effect. Therefore, a magnetic field is produced by an electrical current travelling in a straight or solenoid wire (a wire wrapped into the shape of a coil). The pattern and direction of a wire’s magnetic field: The magnetic field produced by a straight wire forms a concentric circle pattern around the wire (so has no poles). The direction of the magnetic field produced by a straight wire can be found using the right hand grip rule. ○ The thumb shows the direction of current. ○ The fingers show the magnetic field direction. The magnetic field produced by a solenoid forms concentric circles around either side of the coil, like the field produced by a bar magnet. The solenoid has poles (south pole where current is travelling clockwise and vice-versa) so the magnetic field’s direction is from the north to the south pole. The magnetic field of a solenoid is stronger, closer to the ends of the coil (the poles). https://bit.ly/pmt-cc https://bit.ly/pmt-edu https://bit.ly/pmt-cc The magnetic field is stronger in a solenoid: With more turns (each coil’s magnetic field combines to create the overall magnetic field). ○ So solenoid’s have stronger magnetic fields than straight wires. When closer to the wire itself. With a larger current. By adding an iron core (field lines travel more easily through metal than air). Placing a soft iron core in the solenoid makes an electromagnet: The iron core becomes a temporary (induced) magnet when the current is flowing (see Topic 4.1). The magnetic field of electromagnets can be switched off and on, because the magnet is temporary. Electromagnets are used in relays: Relays use one circuit (containing the electromagnet) to turn off/on another circuit by attracting a switch open/closed with the magnetic field produced. ○ They are often used to move scrap-metal (on when picking up, off when dropping) and in electric bells (when on, the magnetic field is produced and attracts an iron armature, hitting the bell and at the same time breaking the circuit to reset the system). Loud speakers also use the magnetic effect, but use permanent magnets to convert electrical signals into sound. The current run through a solenoid around a permanent magnet, is a.c.. The magnetic field’s direction changes as the current direction changes. The changing magnetic field direction moves the coil (due to forces explained in the next section). The moving coil makes the air oscillate in sound waves. To show the pattern of a wire’s magnetic field experimentally: Attach a thick wire vertically through a hole in the middle of cardboard and clamp it in a stand (or loop the wire through holes in the cardboard to form the coil for solenoids). Attach the ends of the wire to a series circuit with a cell, ammeter, and variable resistor. Spread iron filings uniformly on the cardboard. Place a plotting compass on the cardboard. Run a current through the wire and tap the cardboard slightly. Iron filings will align along the magnetic field lines and the plotting compass will show the direction of the magnetic field. https://bit.ly/pmt-cc https://bit.ly/pmt-edu https://bit.ly/pmt-cc 4.5.4 Force on a current-carrying conductor When a current-carrying wire’s magnetic field interacts with an external magnetic field, the wire experiences a force, perpendicular to the direction of the magnetic field. Fleming’s left hand rule shows the relative directions of the force, field, and current. An experiment can demonstrate the existence of these forces: If a current-carrying wire is placed between two magnets which rest on a balance, when a current is passed through the wire it will move within the magnetic field. If the current is reversed or the magnetic field is reversed, the force will be reversed and the wire will move in the opposite direction. A force is also exerted on charged particles moving in a magnetic field (because moving charged particles are current and so cause a magnetic effect). When beams of charged particles enter a magnetic field, the force exerted on the charged particles is perpendicular to the velocity (direction) of the charge. This changes the velocity so the direction of the applied force changes and results in a circular motion of the particle beam (it is deflected). https://bit.ly/pmt-cc https://bit.ly/pmt-edu https://bit.ly/pmt-cc 4.5.5 The d.c. motor A current-carrying coil in a magnetic field can experience a turning effect due to the opposite direction of the forces exerted on either side of the coil. D.c. motors consist of a coil of wire in between two permanent magnets. Current flows through the wire and it experiences a turning effect. The turning effect can be increased by: ○ increasing the current. ○ using a stronger magnetic field. ○ increasing the number of turns on the coil. The motor would stop turning when the coil became parallel with the magnetic field, but a split ring commutator is used, which changes the current direction every half turn. Carbon brushes connect the split ring commutator to the circuit. ○ They disconnect from the commutator every half turn but the coil continues to spin from momentum. ○ When the split ring reconnects, the coil has been flipped and so current flows in the opposite direction. https://bit.ly/pmt-cc https://bit.ly/pmt-edu https://bit.ly/pmt-cc 4.5.6 The transformer A transformer consists of two coils wrapped around a soft iron core and is used to transform the voltage of alternating currents. Transforming voltages means reducing (step down) or increasing (step up) the voltage of an electric current. Transformers are used to step up the voltage in power lines to reduce power loss ○ Loss of power will be lower because higher voltage means a smaller current: 2 power = (current) x resistance P = I2R For a 100% efficient transformer, the power used is constant across the primary and secondary coil, P=IV so: IpVp=IsVs An alternating current in the primary coil creates a changing magnetic field. The iron core becomes magnetised, transmitting the changing magnetic field to the secondary coil and induces an alternating e.m.f. in it. If the secondary coil is part of a complete circuit, this results in a current flowing. A step up transformer has more turns on the secondary coil which means the voltage of the secondary is greater than that of the primary. A step down transformer has fewer turns on the secondary coil which means the voltage of the secondary is less than that of the primary. ○ The turns ratio of the coils is directly proportional to the voltage ratio across either coil: 𝑝𝑟𝑖𝑚𝑎𝑟𝑦 𝑐𝑜𝑖𝑙'𝑠 𝑣𝑜𝑙𝑡𝑎𝑔𝑒 𝑝𝑟𝑖𝑚𝑎𝑟𝑦'𝑠 𝑛𝑢𝑚𝑏𝑒𝑟 𝑜𝑓 𝑐𝑜𝑖𝑙𝑠 𝑉𝑃 𝑁𝑃 𝑠𝑒𝑐𝑜𝑛𝑑𝑎𝑟𝑦 𝑐𝑜𝑖𝑙'𝑠 𝑣𝑜𝑙𝑡𝑎𝑔𝑒 = 𝑠𝑒𝑐𝑜𝑛𝑑𝑎𝑟𝑦'𝑠 𝑛𝑢𝑚𝑏𝑒𝑟 𝑜𝑓 𝑐𝑜𝑖𝑙𝑠 𝑉𝑆 = 𝑁𝑆 https://bit.ly/pmt-cc https://bit.ly/pmt-edu https://bit.ly/pmt-cc