Study Guide Exam 2 PDF
Document Details
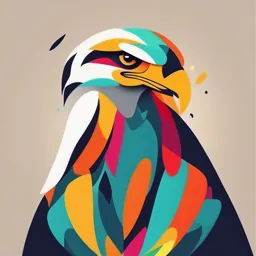
Uploaded by TroubleFreeVolcano6636
Los Medanos College
Tags
Related
Summary
This study guide covers metabolism, including anabolism and catabolism, and the three ways ATP is generated. It also explains the function of metabolic pathways and the role of ATP as an intermediate between catabolism and anabolism. The guide further explores the importance of glucose as an energy source. It also includes sections on aerobic and anaerobic respiration, and fermentation.
Full Transcript
CHAPTER 5 METABOLISM: 1. Define metabolism, and describe the fundamental differences between anabolism and catabolism Metabolism the buildup and breakdown of nutrients within a cell. These reactions provide energy and create substances that sustain life. These reacti...
CHAPTER 5 METABOLISM: 1. Define metabolism, and describe the fundamental differences between anabolism and catabolism Metabolism the buildup and breakdown of nutrients within a cell. These reactions provide energy and create substances that sustain life. These reactions are divided into two categories: Catabolism – breaking down complex molecules into “simpler” molecules. ▪ Provides energy for ADP phosphorylation (ADP to ATP) and building blocks for anabolism ▪ Exergonic – reactions release energy. ▪ Provides energy needed for cellular activities Anabolism – using energy and building complex molecules from simpler ones. ▪ Endergonic – reactions require energy ▪ Essential for growth, repair, and maintenance of cellular structures. 2. Outline the three ways that ATP is generated 1) Substrate-level phosphorylation – enzyme acts as catalyst. ▪ This happens in processes like glycolysis and the Krebs cycle. 2) Oxidative phosphorylation – energy generated from electron transport chain (ETC) used. ▪ Electrons are passed along a series of proteins creating a flow of protons (H+) across a membrane. ▪ Occurs during cellular respiration in mitochondria or the plasma membrane of prokaryotes. 3) Photophosphorylation – light energy used to add phosphate group to ADP. ▪ Creation of ATP via ETC and ATP synthase in the chloroplasts. 3. Explain the overall function of metabolic pathways Metabolic pathways are series of enzymatically catalyzed reactions in a cell. ▪ Are determined and executed by enzymes These pathways either: Break down molecules to release energy (catabolic pathways) Building up molecules by using energy (anabolic pathways) The function of metabolic pathways is to: Extract energy from nutrients (like glucose) or light (photosynthetic organisms) Convert that energy into forms that can be stored (ATP). Produce building blocks for the cell only produces what it needs, conserving energy and resources. 4. How is ATP an intermediate between catabolism and catabolism? ATP links the energy-releasing processes of catabolism with energy-consuming processes of anabolism making it intermediary in cellular metabolism ▪ In catabolism, energy is released from breaking down molecules and this energy is stored in ATP. ▪ In anabolism, ATP provides the energy needed to drive the synthesis of complex molecules from simpler ones. 5. Why is glucose such an important molecule for organism? Glucose is a key molecule for several reasons: ▪ It is a primary source of energy. ▪ Cellular respiration – glucose is broken down and its stored energy is used to produce ATP. ▪ Glucose is a universal fuel. Most cells can metabolize glucose to produce energy. ▪ Serves as starting point metabolic pathways. Example: Glycolysis – glucose is broken down to pyruvate which can enter the Krebs cycle or be used in fermentation. AEROBIC AND ANAEROBIC RESPIRATION; FERMINTATION: 1. Describe aerobic respiration. Include what it does, the reaction equation, where it occurs in prokaryotic and eukaryotic cells. Aerobic respiration – break down of glucose, lipids, or proteins to produce ATP – requires O2 Reaction equation: C6H12O6 + 6O2 → 6CO2 + 6H2O + ATP ▪ C6H12O6 = glucose (used for energy) ▪ O2 = oxygen (use to break down glucose) ▪ CO2 = carbon dioxide (produced as a waste product) ▪ H2O = water (produced as a waste product) ▪ ATP = adenosine triphosphate (energy) Where it occurs: ▪ In eukaryotic cells it primarily occurs in the mitochondria. ▪ In prokaryotic cells it occurs in the cytoplasm glycolysis and the Krebs cycle) and across the plasma membrane (ETC). 2. Describe glycolysis Glycolysis is the first stage of cellular respiration. ▪ Breakdown of 6C glucose (C6H12O6) into two pyruvate 3C (C3H6O3) ▪ Does not require O2 (anaerobic) ▪ Produces net gain of +2 ATP molecules and +2NADH molecules ▪ Produces 2 pyruvate molecules ▪ Glycolysis: glucose → pyruvate + 2ATP and + 2 NADH It occurs in the cytoplasm of both prokaryotic and eukaryotic cells 3. What happens during the preparatory reaction to prepare for the citric acid cycle? Preparatory reaction (pyruvate oxidation) – occurs after glycolysis and before the Krebs cycle. Each pyruvate molecules are converted into acetyl-CoA ▪ Does not require O2 ▪ 1 carbon atom is released from pyruvate (3C) as CO 2 (first CO2 released in cellular respiration) ▪ + 2NADH “hydrogen transport” molecules ▪ The remaining 2C molecules (acetate) binds to Coenzyme A to form acetyl-CoA which enters the Krebs cycle. ▪ Preparatory reaction: pyruvate → acetyl-CoA +2 NADH 4. Describe and explain the products of the Krebs cycle. The Krebs cycle turns twice per glucose molecule since glycolysis produces 2 pyruvate molecules, each of which is converted to acetyl-CoA It occurs in the mitochondrial matrix in eukaryotes and the cytoplasm of prokaryotes For each acetyl-CoA entering the cycle, the products are: ▪ 3 NADH (electron carrier) ▪ 1 FADH2 (electron carrier) ▪ 1 ATP ▪ 2 CO2 (released as a waste product) 5. Describe what happens in the ETC ETC is located in the inner mitochondrial membrane in eukaryotes and the plasma membrane in prokaryotes It is the final step in aerobic respiration Transport of hydrogen as NADH to ETC membrane system Where most ATP is produced: ▪ Electrons from NADH and FADH2 are passed through protein complexes creating a proton (H+) gradient across the membrane ▪ These gradient drives enzyme ATP synthase which synthesizes ATP from ADP and Pi ▪ O2 serves as the final electron acceptor combining with protons and electrons to form water. Generates 32-34 ATP per glucose 6. How do NAD+ molecules function in the ETC? NAD+ is reduced to NADH during glycolysis, the preparatory reaction, and the Krebs cycle. NADH then donates electrons to the first complex of the ETC. As electrons are passed down the chain, protons (H+) are pumped across the membrane contributing to the proton gradient that drives ATP synthesis. 7. Compare and contrast aerobic and anaerobic respiration Aerobic respiration Anaerobic respiration Requires O2 Do not require O2 Produces 36-38 ATP per glucose Produces less than 30 ATP per glucose Uses O2 as the final electron acceptor Uses another electron acceptor like Sulfate, nitrate, or CO2 Occurs in mitochondria (eukaryotes) or across Occurs in organisms that live in environments the plasma membrane (prokaryotes) without O2 8. Describe the chemical reactions of and list some of products of fermentation. ▪ Fermentation – is an anaerobic process that occurs after glycolysis when oxygen is not available. It regenerates NAD+ by transferring electrons from NADH to pyruvate or its derivatives. ▪ Does not require oxygen ▪ Does not use Krebs cycle or ETC ▪ It occurs in the cytoplasm ▪ Produces small amounts of ATP ▪ Two types of fermentation: ▪ Lactic acid fermentation – pyruvate is reduced to lactic lactic acid. Occurs in muscle cells and some bacteria (yogurt production) Yields only 2 ATP Glucose → pyruvate → lactate ▪ Alcohol fermentation – pyruvate is converted to ethanol and CO2. This occurs in yeast and some bacteria (brewing and baking) Yields only 2 ATP Glucose → pyruvate → ethanol + CO2 9. List four compounds that can be produced from pyruvic acid by an organism that uses fermentation. 1. Lactic acid (cheese, yogurt) 2. Ethanol (beer, wine) 3. Acetone (pharmaceutical, industrial use) 4. Citric acid (flavoring) 10. Compare the energy yield (ATP) of aerobic respiration, anaerobic respiration, and fermentation. Aerobic respiration – 36-38 ATP per glucose Anaerobic respiration – less than 30 ATP per glucose Fermentation – 2 ATP per glucose 11. Where do lipids and proteins enter the respiration process? Lipids: ▪ Fats are broken down into glycerol and fatty acids ▪ Glycerol enters glycolysis ▪ Fatty acids are broken down into smaller units (acetyl-CoA) in process called beta- oxidation. The acetyl-CoA then enters Krebs cycle to produce ATP Proteins: ▪ Broken down into amino acids ▪ Amino acids get their nitrogen removed and then converted into molecules that can enter at different respiration like: - Glycolysis - Preparatory reaction - The Krebs cycle 12. What “reaction step” is common between all three processes (aerobic, anaerobic, fermentation)? The common step in all three processes is glycolysis. In all cases, glycolysis is converted into pyruvate generating small amount of ATP and NADH. 13. Respiration comparison table: Characteristics Aerobic Anaerobic Fermentation respiration respiration O2 requirement Requires O2 Does not require Does not require O2 O2 Final electron Oxygen Nitrate, sulfate, Organic acceptor or other molecules (ex. compounds Pyruvate) ATP yield per 36-38 ATP Less than 30 2 ATP glucose ATP Location in Mitochondria Cytoplasm Cytoplasm eukaryotes Location in Cytoplasm and Cytoplasm and Cytoplasm prokaryotes plasma plasma membrane membrane End products CO2, H2O CO2, Nitrate, or Lactic acid, Sulfide ethanol, CO2 Process involved Glycolysis, Glycolysis, Glycolysis Krebs cycle, Krebs cycle, ETC ETC 14. Summarized respiration processes: Aerobic respiration: Glycolysis → 𝐾𝑟𝑒𝑏𝑠 𝑐𝑦𝑐𝑙𝑒 → 𝐸𝑇𝐶 (𝑢𝑠𝑖𝑛𝑔 𝑜𝑥𝑦𝑔𝑒𝑛 𝑎𝑠 𝑡ℎ𝑒 𝑓𝑖𝑛𝑎𝑙 𝑒𝑙𝑒𝑐𝑡𝑟𝑜𝑛 𝑎𝑐𝑐𝑒𝑝𝑡𝑜𝑟) Anaerobic respiration: Glycolysis → 𝐾𝑟𝑒𝑏𝑠𝑐𝑦𝑐𝑙𝑒 → 𝐸𝑇𝐶(𝑢𝑠𝑖𝑛𝑔 𝑛𝑜𝑛 − 𝑜𝑥𝑦𝑔𝑒𝑛 𝑎𝑐𝑐𝑒𝑝𝑡𝑜𝑟) Fermentation: Glycolysis → 𝑓𝑒𝑟𝑚𝑖𝑛𝑡𝑎𝑡𝑖𝑜𝑛(𝑐𝑜𝑛𝑣𝑒𝑟𝑠𝑖𝑜𝑛 𝑜𝑓 𝑝𝑦𝑟𝑢𝑣𝑎𝑡𝑒 𝑡𝑜 𝑙𝑎𝑐𝑡𝑖𝑐𝑎 𝑐𝑖𝑑, 𝑒𝑡ℎ𝑎𝑛𝑜𝑙 𝑒𝑡𝑐. ) CHAPTER 6 MICROBIAL GROWTH: 1. Differentiate between linear and exponential growth Linear growth – constant growth rate adding the same number of cells or bacteria per unit of time. Exponential growth – occurs by multiplication not addition, meaning the population doubles at regular intervals. ▪ Rapid increase, population size doubles with each round of division ▪ Growth rate changes in proportion to the prior size 2. Explain generation time, theoretical growth population calculation. Generation time – time it takes for a bacterial population to double in number. ▪ Can vary between different bacteria. ▪ Most bacteria have generation time of 1-2 hours ▪ Times can range from 20 minutes to 24 hours. Theoretical growth calculation – to calculate the theoretical growth of a bacterial population. ▪ Formula: Nt = N0 x 2n - Nt the number of cells after certain time - N0 first number of cells - n the number of generations (calculated by dividing the total time by the generation time) 3. Bacterial reproduction: compare and contrast binary fission vs budding Binary fission Budding Most common method of bacterial reproduction Less common in bacteria but seen in some species Parent cell duplicates its DNA and divides into Small outgrowth, or bud forms on the parent cell. two identical daughters The bud grows until it detaches becoming a new smaller daughter cell Division is symmetrical meaning the two Division is asymmetrical meaning the daughter daughter cells are the same size cell is smaller than the parent cell 4. Describe the bacterial growth cycle Consist of distinct phases that represent changes in the growth rate of population over time. ▪ Happens in a closed system, like lab culture, where nutrients are limited. 5. Describe the 4 stages of bacterial population growth curve – what is happening in each stage? 1. Lag phase: ▪ “flat” period of adjustment, adapting to their environment. ▪ Cells are metabolically active preparing for division. ▪ Little growth 2. Log (exponential) phase: ▪ Period of maximum growth will continue as long as cells have adequate nutrients and a favorable environment. ▪ Population doubles at regular intervals and resources are abundant. 3. Stationary phase: ▪ Rate of cell growth is equals rate of cell death caused by depleted nutrients, O2, excretion of organic acids and pollutants ▪ Growth slows down as nutrients become depleted and waste products accumulate. ▪ The number of new cells produced equals the number of cells dying so the population size remains stable. 4. Death (decline) phase: ▪ As limiting factors intensify, cell death will start to occur. ▪ Cells begin to die at an exponential rate because of the complete depletion of nutrients and the accumulation of toxic waste products. PHYSICAL GROWTH REQUIREMENTS: 1. Classify microbes into four groups based on preferred temperature range: Temperature adaptation classifications: ▪ Psychrophiles – cold-loving - Grows between -20°𝐶 and -10°𝐶 - Found in artic and Antarctic regions ▪ Mesophiles – moderate-temperature-loving - Grows between 20°𝐶 𝑎𝑛𝑑 40°𝐶 - These include many human pathogens, as body temperature is around 37°𝐶 ▪ Thermophiles – heat-loving - Optimum growth >45°𝐶 - Found in hot springs and compost heaps ▪ Hyperthermophiles – thrive at extremely high temperatures - Optimum growth temperature >70°𝐶 - Found in environments like hydrothermal vents 2. Classify microbes by pH, osmotic, and atmospheric pressure conditions: pH adaptations classifications: ▪ acidophiles – grow in acidic environments (pH 8) ▪ neutrophiles – grow best at neutral pH (pH 6.5 to 7.5). Most bacteria fall into this category. Osmotic adaptation classifications: ▪ Halophiles – live in salty environments ▪ Extreme (obligate) halophiles – require extremely high levels of salt for growth (high osmotic pressure) ▪ Halotolerant (facultative) – can tolerate high salt but do not require high salt to grow Osmotic pressure: difference in solute concentration inside vs outside cell ▪ Hypertonic environment ▪ Higher in solutes than inside the cell ▪ Plasmolysis due to loss of water ▪ Bacterial plasmolysis – H2O leaves cell – growth inhibited Atmospheric pressure: Barometric adaptation classification ▪ Barophiles – can survive in environments under extreme pressure such as deep-sea organisms - Will rupture if exposed to normal atmospheric pressure 3. Identify how and why the pH of culture media is controlled: The pH of culture media is controlled using buffers to maintain a stable environment for microbial growth. Microbes produce acidic or basic metabolic byproducts which can alter the pH of the media, inhibiting growth. Buffers help to neutralize these byproducts, ensuring the pH remains suitable for the microbe being cultured. 4. Preferred pH for most bacterial species vs fungal species: Standard pH growth ranges: ▪ Most bacteria grow between pH 6.5 and 7.5 ▪ Molds and yeast grow between pH 5 and 6 5. Explain the importance of osmotic pressure to microbial growth: Osmotic pressure refers to the balance of water between the inside of a cell and its external environment. ▪ Hypertonic — water will leave the cell causing it to shrink (plasmolysis) which can inhibit growth ▪ Hypotonic — water enters the cell which can cause the cell to swell and potentially burst. Maintaining proper osmotic pressure is essential for cells to prevent water loss or gain, which affects their ability to grow and survive. CHEMICAL GROWTH REQUIREMENTS: 1. Four main elements needed in large amounts for microbial growth: a. Carbon — structural backbone of all organic molecules required for all life forms. ▪ Heterotrophs – use organic molecules as energy (sugar, protein, lipids). ▪ Autotrophs – use CO2 to make own organic molecules. b. Nitrogen — components of amino acids, DNA, RNA and ATP. ▪ Most bacteria decompose protein material for their nitrogen source. ▪ A few bacteria use N2 in nitrogen fixation ▪ Some bacteria use N2+ or NO3- from organic material. c. Sulfur — important for the synthesis of some acids (like cysteine) and vitamins. Required for protein structure and enzyme function. ▪ Some bacteria use SO42- or H2S ▪ Most bacteria decompose protein for their sulfur source. d. Phosphorus — essential for the formation of DNA, RNA, ATP, and phospholipids. ▪ Found in membranes – phospholipid bilayer. 2. How do we acquire each of the four elements? ▪ Carbon: ▪ Heterotrophs get carbon from organic compounds (like glucose). ▪ Autotrophs fix carbon from CO2 in the atmosphere. ▪ Nitrogen: ▪ Acquired from ammonia (NH3), nitrates (NO3-) or atmospheric nitrogen (N2) in nitrogen fixing bacteria. ▪ Sulfur: ▪ Obtained from sulfate (SO42-), hydrogen sulfide (H2S), or organic sulfur-containing compounds. ▪ Phosphorus: ▪ Taken up as phosphate ions (PO43-) from the environment. 3. Explain how microbes are classified on the basis of oxygen requirements Obligate aerobes Obligate anaerobe Facultative Aerotolerant Microaerophiles anaerobes anaerobe Require oxygen for Cannot survive in Can grow with or Tolerate oxygen Require oxygen growth and cannot the presence of without oxygen. but cannot use it lower than air survive without it oxygen, as it is toxic to them. Growth occurs only Growth occurs only Growth is best where Growth occurs Growth occurs where high where there is no most oxygen is evenly; oxygen has only where a low concentrations of oxygen present nut occurs no effect concentration of oxygen have throughout tube oxygen has diffused into the diffused into medium. medium Presence of Lacks enzymes to Presence of enzymes Presence of one Produce lethal enzymes catalase neutralize harmful catalase and SOD enzyme, SOD, amounts of toxic and SOD allows forms of oxygen; allows toxic forms of allows harmful forms of oxygen toxic forms of cannot tolerate oxygen to be forms of oxygen to if exposed to oxygen to be oxygen neutralized; can use be partially normal neutralized oxygen neutralized; atmospheric tolerates oxygen oxygen 4. Three enzymes that help aerobes avoid damage by toxic forms of oxygen: Oxygen can produce toxic byproducts such as superoxide radicals (O2-), hydrogen peroxide (H2O2), and hydroxyl radicals (OH∙). Aerobes have developed enzymes to neutralize these harmful compounds: ▪Superoxide dismutase (SOD) — this enzyme converts the toxic superoxide radicals (O2-), into hydrogen peroxide (H2O2) and oxygen (O2 ). o By converting SOD radicals into less harmful compounds, SOD prevents oxidative damage to cellular components. o Is found in all organisms that can grow in the presence of oxygen. ▪ Catalase — converts hydrogen peroxide (H2O2) which is still toxic to cells into water (H2O) and oxygen (O2). o This detoxifies the hydrogen peroxide and protects the cell from oxidative damage. ▪ Peroxidase (hydrogen peroxidase) — breaks down hydrogen peroxide (H2O2) but instead of producing oxygen it produces water by using reducing agents like NADH or glutathione. o This process helps aerobes avoid the buildup of toxic hydrogen peroxide without generating oxygen. 5. Describe biofilms and their potential for causing infection: Biofilms— are communities of microorganisms (bacteria, fungi, or other microbes) that are embedded in a self-produced matrix of extracellular polymeric substances (EPS), such as proteins, medical devices, tissues, or industrial equipment. o Form slime or hydrogels that adhere to surfaces - Bacteria communicate cell-to-cell via quorum sensing o Share nutrients o Shelter bacteria from harmful environmental factors ▪ Formation: 1. Microbes attach to a surface 2. They secrete EPS which protects them and allows them to stick together. 3. As the biofilms grows, different layers of microbes form and the biofilm become highly structured. ▪ Potential for causing infection: 1. Biofilms protect bacteria from environmental threats including antibiotics and the immune system, making them much harder to eradicate. 2. They can form on medical devices like catheters, heart valves, joint implants, and other surfaces inside the human body leading to chronic infections. 3. Bacteria within biofilms are up to 1,000 times more resistant to antibiotics compared to free-floating bacteria. 6. Why are biofilms of special concern in the medical industry? Antibiotic resistance — it creates a protective environment for bacteria making them highly resistant to antibiotics. This complicates treatment, as higher doses or more potent drugs may be required but even then, they may fail to fully penetrate the biofilm. Chronic infections — can lead to persistent, chronic infections. They are difficult to eliminate and often cause infections to reoccur, such as in cases of UTI or chronic wounds. Medical devices — commonly form on medical devices. Once formed, they can seed infections into the bloodstream or surrounding tissues causing life-threatening infections like sepsis or endocarditis. It involved in ~70% of medical infections. Increased healthcare costs — biofilm-related infections often require longer hospital stays, repeated surgeries, and additional treatments which raises the overall cost of care and increases the burden of healthcare systems. 7. Direct methods of measuring cell growth: counting microbial cells These methods measure the actual number of cells in a population or culture. 1. Plate count: ▪ Count colonies on plates that have 30 to 300 CFUs ▪ To ensure the right number of colonies, the original inoculum must be diluted via serial dilution. Two bacterial dilution techniques: A. Spread plate method – bacteria diluted in liquid broth and spread directly on surface of agar plate. B. Pour plate method – bacteria diluted into a soft agar and poured onto agar plate 2. Filtration: ▪ Solution passed through a filter that collects bacteria ▪ Filter is transferred to a Petri dish and grows as colonies on the surface 3. Most probable number (MPN) method ▪ Multiple tube: inoculate tubes with different sample volumes ▪ Count positive tubes ▪ Compare with a statistical table 4. Direct microscopic count ▪ Volume of a bacterial suspension placed on a slide with grip ▪ Average number of bacteria per viewing field is calculated ▪ Uses a special Petroff-Hausser cell counter 𝑛𝑢𝑚𝑏𝑒𝑟 𝑜𝑓 𝑐𝑒𝑙𝑙 𝑐𝑜𝑢𝑛𝑡𝑒𝑑 Number of bacteria/ml = 𝑣𝑜𝑙𝑢𝑚𝑒 𝑜𝑓 𝑎𝑟𝑒𝑎 𝑐𝑜𝑢𝑛𝑡𝑒𝑑 8. Indirect methods of measuring cell growth: These methods estimate cell growth by measuring factors related to cell activity rather than directly counting cells. Three common indirect methods: A. Turbidity – measurement of cloudiness with spectrophotometer B. Metabolic activity – amount of metabolic product (color change) is proportional to the number of bacteria C. Dry weight – bacteria are filtered, dry, and weighed used for filamentous organisms. CHAPTER 7 CONTROLLING MICROBIAL GROWTH: 1. Definitions and differences of key terms related to microbial control: Sterilization – the process of completely removing or destroying all forms of microbial life, including bacterial spores and viruses. This is the highest level of microbial control. Disinfection – the process of eliminating or reducing harmful microorganisms on inanimate objects. It does not necessarily kill all microbes especially bacterial spores. Antisepsis – similar to disinfection but it is the process of eliminating microbes on living tissues (skin wounds). Antiseptics are used to reduce to risk of infection. Degerming – the mechanical removal of microbes from a limited area such as swabbing the skin with alcohol before injection. Sanitization – the process of lowering microbial counts to safe public health levels. It doesn’t eliminate all microbes but reduces them to acceptable levels. Biocide/germicide – a chemical or agent that kills microorganisms. “biocide” is a broad term, while “germicide” focuses on agents that specifically kill germs. Bacteriostatic – agents or conditions that inhibit bacterial growth without necessarily killing them. The bacteria remain viable but cannot grow or reproduce. Bactericidal – agents that kill bacteria outright. Unlike bacteriostatic agents, bactericidal ones lead to cell death. Asepsis – the absence of significant contamination. Aseptic techniques are practices that prevent microbial contamination, especially in medical or lab settings. 2. Four factors related to effective microbial treatment: 1. Number of microbes—the more microbes present, the longer it takes to kill them all. ▪ If rate of killing is the same – will take longer to kill larger population. 2. Environmental influences – factors like temperature, pH, and the presence of organic matter (blood pus), can affect the efficacy of treatments. ▪ Warmer temperatures and lower pH often increase the effectiveness of chemical treatments. 3. Time of exposure – the longer the microbial control agent is applied, the more effective it is at killing microbes. 4. Microbial characteristics – different microbes have varying levels of resistance. ▪ For example: spores and mycobacteria are more resistant to treatments than typical vegetative bacteria. 3. Exponential death rate of microbes: Microbes typically die at a constant rate when exposed to lethal agents. The death rate remains constant over time – the more microbes there are at the start, the longer it takes to kill them all. Example: 90% of population is killed every minute – is called exponential (logarithmic) death. 4. Patterns of microbial death caused by treatments with microbial control agents: Microbial death occurs in a pattern: ▪ Certain percentage of microbes die in a given time interval, rather than all dying at once. ▪ As treatment continues, the number of surviving microbes decrease exponentially. Why is a solution with more bacteria takes longer to sterilize? ▪ If a solution contains 1 million bacteria, each time interval will kill a specific percentage of the bacteria (example: 90% per minute). Therefore, a solution with more starting bacteria (1 million vs 500,000) will take longer to reduce the bacterial population to zero, even though the death rate per unit of time is constant. 5. Different modes of action for microbial control agents on cellular structures: Microbial control agents target specific cellular structures or functions to kill or inhibit microbes. A. Alteration of cell wall or plasma membrane permeability o Damage to sugars, lipids or proteins o Causes cell content to leak out o Agents like alcohol or detergents can damage the peptidoglycan layer of bacterial walls causing the cell to lyse B. Damage to proteins (enzymes) o Denaturing proteins – lose “folding” structure, thus function o Enzymes often targeted o Heat, radiation, or chemicals can denature proteins and enzymes, stopping cellular metabolism C. Damage to nucleic acids o Damage – cannot replicate or produce proteins o chemicals like formaldehyde can damage DNA or RNA preventing replication and protein synthesis. o Usually heat, radiation or chemicals D. Interfering with protein synthesis o Ribosomes, DNA or RNA polymerase 6. How a chemical microbial control agent targeting plasma membranes would affect humans: A chemical agent that targets plasma membranes could be harmful to human cells because all living cells including human cells have plasma membranes. If a chemical is too strong or non- selective, it could damage human tissues causing cell death or tissue irritation. However, if the agent is carefully designed to be more effective against microbial membranes, it can target microbes with less damage to human cells. PHYSICAL METHODS OF CONTROL: 1. Comparing and contrasting types of heat for microbial control Moist heat: Pasteurization Dry heat: Filtration A) boiling or B) A) Direct B) C) hot-air flowing autoclaving flaming incineration sterilization steam Mechanism Protein Protein Protein Burning Burning to Oxidation Separation of action denaturation denaturation denaturization contaminants ashes of bacteria to ashes from suspending liquid Application Kills Very Heat Very Very Very Removes vegetative effective treatment for effective effective effective microbes bacterial method of milk (72℃ for method of method of method of by passage and fungal sterilization; about 15 sec) sterilization sterilization sterilization of a liquid pathogens at about 15 that kills all but requires or gas and almost psi of pathogens temperature through a all viruses pressure and most of 170℃ for screenlike within 10 (121℃), all non- about 2 material. mins; less vegetative pathogens. hours. effective on cells and endospores their endospores are killed in about 15 mins. Why a can of pork takes longer to sterilize than soup? ▪ The solid pieces of pork in the can provide insulation, slowing the transfer of heat compared to a liquid medium like soup. Additionally, the density of the soup pork requires more time for the heat to penetrate and reach the necessary temperature for sterilization. 2. Microbial growth control by pasteurization: How it controls growth ▪ High temperature / short time ▪ Kills pathogenic organisms ▪ Reduces number of spoilage microbes to safe level (expiration date) ▪ Doesn’t denature proteins What they aim to kill: ▪ Listeria monocytogenes ▪ Salmonella spp. ▪ Mycobacterium tuberculosis ▪ Brucella spp. 3. Methods of microbial growth suppression: Filtration: ▪ Filters with pores small enough to trap microbes physically remove them from air or liquid. Common filters are >0.22 or 0.45 𝜇𝑚 in pore size which can capture most bacteria. ▪ High-efficiency particulate air (HEPA) filters remove microbes >0.3 𝜇𝑚 ▪ Used for heat sensitive materials Low temperature: ▪ Inhibits growth – doesn’t kill (refrigeration around 4℃) or freezing to slow down microbial metabolism and reproduction. ▪ Listeria grows at low temperature High pressure: ▪ Denature proteins ▪ Disrupts cellular membranes and inactivates enzymes, effectively killing or inhibiting microbial growth Desiccation ▪ Removing water from microbial cells prevents them from growing or reproducing since water is essential for metabolism ▪ Can remain viable for years ▪ Add H2O will resume growth and division ▪ Lyophilization – freeze drying Osmotic pressure: ▪ Use salts and sugar to create hypertonic environment ▪ This dehydrates the microbes and inhibits growth ▪ Cause plasmolysis 4. Comparing ionic and non-ionic: Radiation Mechanism of Mode of actions Effectiveness Application action Ionizing Destruction of Ionizing radiation Very effective in Sterilizing medical DNA works by ionizing sterilization because it supplies, water in the cells penetrates deeply, pharmaceuticals, and producing reactive killing all microbial life, food preservation hydroxyl radicals OH∙ including spores. (irradiation of spices, that damage DNA and meats) other cellular components. It causes double strand breaks in DNA leading to mutations or cell death. Non-ionizing Damage of DNA Non-ionizing radiation UV light is less Disinfecting surfaces, causes the formation penetrating and air, and water (UV of thymine dimers in mostly used for lamps in hospital DNA which distort the surface disinfection rooms or water DNA structure and purification) interfere with replication and transcription. This leads to cell death if not repaired. 5. Mode of action of microbial control agents targeting plasma membrane: A chemical microbial control agent that targets the plasma membrane would disrupt the membrane’s integrity causing leakage of cellular contents which eventually leads to cell death. Since human cells also have plasma membranes, agents that disrupt microbial membranes can also be toxic to human cells, especially at high concentrations. This is why such agents need to be carefully formulated to target microbial cells selectively without harming human tissues. CHEMICAL METHODS OF CONTROL: 1. Three main principles of effective chemical disinfection: a. Concentration of disinfectant ▪ Higher concentrations of disinfectant are generally more effective at killing microbes. However, there’s often an optimal concentration where the disinfectant works best without being too corrosive or toxic. ▪ Some disinfectants may without require dilution to be effective without damaging surfaces or tissues. b. Time of exposure ▪ The longer the chemical is in contact with the surface or object, the more effective it will be at killing or inhibiting microbial growth. ▪ Insufficient contact time can result in incomplete disinfection. c. Nature of the microbial population ▪ Different microbes have varying resistance to disinfectants. Example, bacterial spores and mycobacteria are more resistant, while vegetative bacteria and viruses are generally more susceptible. The effectiveness of the disinfectant depends on the type of microorganism being targeted. 2. General modes action and preferred uses for chemical disinfectant Alcohols (OH-) o Ethanol – pure ethanol less effective than diluted. Requires water to react and denature protein o Isopropanol – “rubbing alcohol”. Best “alcohol” antiseptic and disinfectant ▪ Denature proteins and dissolve lipids in the cell membrane ▪ Skin antiseptics (hand sanitizers), disinfecting surfaces. Often used for degerming before injections or minor surgeries ▪ No effects on endospores and non-enveloped viruses ▪ Best effect on capsulated/enveloped bacteria/viruses ▪ Ineffective as antiseptic against wounds – causes coagulation layer of protein – bacteria can grow Phenol and Phenolics ▪ Disrupt cell walls, membranes, and inactivates enzymes ▪ Injure lipids of plasma membranes causing leakage ▪ Surface disinfection especially in hospitals. ▪ Useful against a broad spectrum of organisms, including mycobacteria o Phenol = carbolic acid - Rarely used currently as antiseptic or disinfectant - Irritant and odorous Essential oils: phenol mixture extracted from plants - Centuries old traditional medicine to preserve food o Phenolics - Altered phenol to reduce irritating qualities and increase antimicrobial activity Aldehydes – functional group with the structure -- CHO o Inactive proteins by covalently cross-linking with protein functional groups - -NH2, -OH, -COOH, -SH o Used for preserving specimens and in medical equipment o Formaldehyde – used to preserve biological equipment o Glutaraldehyde – relative of formaldehyde - More effective than formaldehyde Bisphenols ▪ Contain two phenol groups connected by a bridge ▪ Disrupt plasma membranes ▪ Example: Hexachlorophene and Triclosan o Hexachlorophene – particularly effective against gm+ Staphylococci and Streptococci o Triclosan – antibacterial soaps, toothpaste, mouthwash - Inhibits enzyme that synthesizes lipids for membrane - Especially effective against gm+ bacteria Biguanides ▪ Organic compound - HN(C(NH)NH2)2) ▪ Water soluble – gives highly basic solution ▪ Broad spectrum of activity ▪ Primarily targeting bacterial cell membranes ▪ Most effective against gm+ ▪ Are effective against gm- [except Pseudomonas] ▪ Chlorohexidine: - Used in surgical hand scrubs - Disrupt plasma membranes Heavy metals and their compounds ▪ Oligodynamic action – very small (oligo) amounts of heavy metals exert antimicrobial activity o Several metals are bactericidal or antiseptic – Ag, Hg, Cu, Zn - Denatures protein – metal ions combine with sulfhydryl groups on proteins ▪ Mercuric chloride prevents mildew in paint ▪ Copper sulfate is an algicide (kills algae) ▪ Zinc chloride is used in mouthwash ▪ Silver nitrate is used to prevent ophthalmia neonatorum o Ophthalmia neonatorum – bacterial infection as path through birth canal Silver nitrate used as treatment 3. Mode of action for halogens: Halogens – reactive ▪ Iodine – one of oldest/most effective antiseptics - Impairs protein synthesis and alters membranes Chlorine ▪ Highly electronegative oxidizing agent ▪ Shuts down cellular enzyme systems ▪ Bleach: hypochlorous acid (HOCI) ▪ Chloramine: chlorine + ammonia 4. Appropriate uses for surface-active agents: Surface-active agents or surfactants ▪ Substance which lowers the surface tension of the medium in which it is dissolved ▪ Soaps and detergents o Soaps – good de-germing method but little value as antiseptic o Emulsifies fats – breaks them up then wash away ▪ Acid-anionic sanitizors – combinations of acid with surface-active agents o Anions react with plasma membrane o Effective only at low pH o Phosphoric acid combined with surface agent ▪ Quaternary ammonium compounds (quats) – strong bases and their salts derived from ammonium o Cations are bactericidal, denature proteins, disrupt plasma membrane o Modifications of ammonium ion (NH 4-) – affect membrane permeability; loss of fluids (plasmolysis) o Strongly bactericidal against gm+ o Less for gm- o Doesn’t kill endospores 5. Use-dilution test and disk-diffusion method Use-dilution test – determines the effectiveness of a disinfectant by applying it to surfaces contaminated with microbes and then measuring microbial survival. ▪ Metal cylinders are dipped in test bacteria at different dilutions and dried ▪ Cylinders are placed in disinfectant for 10 minutes at 20℃ ▪ Cylinders transferred to culture media to determine whether the bacteria survived treatment CHAPTER 20 CHEMOTHERAPHY: 1. Terms related to chemical control: Antimicrobial drugs – synthetic substances that interfere with the growth of microbes Antibiotic – substance produced by a microbe that in small amounts inhibits another microbe Bactericidal – kills microbes directly Bacteriostatic – prevents growth of microbes Selective toxicity – selectively finding and destroying pathogens without damaging the host 2. Describe three ways microbes damage cells and cause disease: a. Direct damage – disrupt host cell function; produce waste product ▪ Some microbes invade and reproduce inside host cells leading to cell death or dysfunction. Bacteria for example may release enzymes that break down host tissue b. Using host nutrients: some microbes cause disease by triggering an excessive or inappropriate immune response leading to inflammation and tissue damage ▪ Side spores – bacteria whose proteins that bind iron more tightly than host cell; many hemolytic c. Produce toxins: poisonous substances that induce fever, diarrhea, shock, heart problems ▪ Some microbes cause disease by triggering an excessive or inappropriate immune response, leading to inflammation and tissue damage Describe exotoxin and endotoxin and the general differences between the two: Endotoxin Exotoxin Part of the outer membrane of gm- bacteria proteins secreted by living cells (gm+ (LPS) mostly) Released when bacteria die and the cell wall Destroy host cell; inhibit cell functions breaks – Lipid A Less specific but can trigger intense immune Extremely potent and cause specific effects responses leading to fever, inflammation, and (neurotoxins, enterotoxins) even septic shock Example: botulinum toxin produced by Example: LPS from E. coli Clostridium botulinum How viruses damage cells and cause disease: ▪ Invasion – virus infects by binding specific cell type and inserting its nucleic acid (DNA or RNA) into cell. Cell then replicates, transcribe/translates viral nucleic acid – producing more virus ▪ Cell lysis – new virus lysis out of cell, killing cell. ▪ Chronic infection – some viruses cause long term damage by integrating into host genome or creating a persistent infection damaging cells over time (HIV) 3. Describe an antibiotic; name the microbe types that produce most antibiotics; differentiate antibiotic with disinfectant. ▪ Antibiotics – a natural or synthetic compound that kills or inhibits the growth of bacteria used to treat bacterial infections. ▪ Molecule produced by one bacteria/fungus species toxic to another bacteria/fungus species. ▪ Target prokaryotic or fungi cell characteristics Microbe types that produce most antibiotics: ▪ Bacteria – especially species from the Streptomyces genus ▪ Fungi – some mols like penicillium produce antibiotics Difference between antibiotic and disinfectant: ▪ Antibiotics – are specifically designed to be sued inside the body to treat infections by selectively targeting bacterial cells without harming the host cells ▪ Disinfectant – are chemicals used on non-living surfaces to kill or inhibit microorganisms and are too toxic for internal use in the body. 4. Describe potential problems of chemical treatment for bacterial, viral, and fungal infections: Bacterial infection – the overuse or misuse of antibiotics can lead to antibiotic resistance, where bacteria evolve to survive treatments. Some treatments can also disrupt the body’s normal flora leading to conditions like superinfections (ex., Clostridium diffcile infections). Viral infections – antiviral drugs can be limited in effectiveness because viruses use host cells to replicate, making it difficult to target the virus without harming host cells. Additionally, viruses can develop resistance to antiviral treatments. Fungal infections – treating fungal infections is challenging because fungi are eukaryotic, like human cells meaning antifungal drugs may have toxic effects on human cells. Additionally, some fungi develop resistance to antifungal treatments reducing the options for effective therapy 5. Define the following terms: spectrum of activity, broad-spectrum antibiotic, narrow-spectrum antibiotic, superinfection. Spectrum of activity – the range of microorganisms an antimicrobial agent can target. This can be broad or narrow depending on whether the drug affects many species or just a few. Broad-spectrum antibiotic – an antibiotic that is effective against a broad-range of bacterial species including both gm+ and gm- bacteria. Example: Tetracycline Narrow-spectrum antibiotic – an antibiotic that targets narrow or specific types of bacteria often effective against either gm+ or gm – bacteria. Example: Penicillin G. Superinfection – a secondary infection that occurs when broad-spectrum antibiotics kill of normal, healthy flora allowing resistant or opportunistic pathogens to thrive. Example: yeast infections after antibiotic treatment. 6. Compare and contrast broad vs. narrow-spectrum antibiotics Broad-spectrum antibiotics Narrow-spectrum antibiotics Advantages Effective when the causative organism Target specific bacteria reducing the is unknown or when there are mixed likelihood of disturbing normal flora and infections causing resistance in unrelated species Disadvantages Can kill beneficial bacteria leading to Only effective if the specific pathogen is superinfections and promote antibiotic known resistance in non-targeted bacteria Example Ciprofloxacin (targets variety of Isoniazid (specific to Mycobacterium bacteria) tuberculosis) 7. What is a potential danger with using broad-spectrum antibiotics for treatment? The main danger of using broad-spectrum antibiotics is that they can kill a wide range of bacteria, including beneficial microbes in the body. This can lead to superinfections by opportunistic pathogens such as yeast C. difficle which flourish when normal bacteria are wiped out. Additionally, broad-spectrum antibiotics promote development of antibiotic resistance in wide range of bacteria. 8. Explain why it is important to understand the modes of action for antimicrobial drugs Understanding how antimicrobial drugs work (their mode of action) is important for selecting the most appropriate treatment. It helps to: ▪ Avoid inappropriate use of antibiotics which could lead to resistance ▪ Ensure that the drug targets the pathogen without harming the host ▪ Inform the development of new drugs to target resistant strains 9. Identify five modes of action of antibiotics: 1. Inhibition of cell wall synthesis – bacteria have cell walls made of peptidoglycan which human cells lack. Antibiotics like Penicillin block the enzymes needed to build these walls causing bacterial cells to burst. ▪ Blocks cell wall cross-linking ▪ Inhibits enzyme that cross-link peptidoglycan. ▪ Example: Penicillin – bactericidal / broad-spectrum o Targets the bacterial cell wall by inhibiting peptidoglycan synthesis 2. Inhibition of nucleic acid synthesis (replication) – there drugs target bacterial enzymes responsible for DNA and RNA synthesis which differ from those in human cells ▪ Blocks “strand stabilizer” of unwound DNA o Inhibits DNA gyrase enzyme o Topoisomerase does this in eukaryotes ▪ Example: Ciprofloxacin – bactericidal / broad-spectrum o Inhibits bacterial DNA gyrase preventing DNA replication 3. Inhibition of essential metabolites – some antibiotics block metabolic pathways in bacteria that are not found in human cells, such as the synthesis of folic acid. ▪ Target nucleic acids and amino acids synthetic pathways ▪ Example: Sulfa antibiotics – inhibit the bacterial synthesis of folic acid, which is necessary for DNA production, but human cells acquire folic acid from the diet. o Competitive inhibitors for enzyme that act on PABA (para-amino-benzoic acid) o PABA needed for bacterial nucleotide synthesis o Human cells do not use PABA to make folate 4. Inhibition of protein synthesis (translation inhibitors) – bacterial ribosomes (responsible for translation) are structurally different from human ribosomes, making it possible to target bacterial protein synthesis without affecting human cells. ▪ Bind ribosome – inhibit tRNA binding ▪ Distort ribosome – disrupting mRNA code ▪ Example: Tetracycline – binds to the 30S subunits of the bacterial ribosome, preventing the attachment of tRNA and halting protein synthesis (translation). o Bacteriostatic / broad-spectrum ▪ Example: Streptomycin – binds and distorts ribosome o mRNA code cannot be read o bactericidal / broad-spectrum 5. Transcription inhibitor – type of antibiotic that blocks the process of transcription which is the synthesis if RNA from a DNA template. These drugs prevent bacteria from producing essential needed for survival. ▪ They typically work by binding to RNA polymerase, the enzyme responsible for reading the DNA and synthesizing RNA. ▪ This prevents the enzyme from functioning properly, stopping the transcription of DNA into messenger RNA (mRNA). ▪ Example: Rifampin (Rifampicin) – targets bacterial RNA polymerase, blocking the transcription process. o It is commonly used to treat bacterial infections like tuberculosis and works specifically on bacteria because the structure on bacterial RNA polymerase is different from that in human cells. 6. Explain a mode of action and provide an example for an anti-fungal antibiotic: Inhibition of ergosterol synthesis – many antifungal drugs target the synthesis of ergosterol, an essential component of fungal cell membranes. ▪ Disrupts ergosterol production compromises the integrity of the cell membrane leading to cell death. ▪ Example – Fluconazole, an antifungal drug in the azole class, inhibits the enzyme lanosterol 14a-demethylase which is involved in ergosterol synthesis. This result in a weakened cell membrane and cell death. Describe the challenges of using anti-fungal antibiotics: ▪ Eukaryotic similarity – fungi are eukaryotic organism, like human cells. - Similar cellular structures and metabolic processes are more similar to humans than bacteria - Difficult to target fungi without harming human cells ▪ Toxicity – often have higher risk of toxicity and side effects compared to antibiotics used for bacterial infections. ▪ Resistance – fungi can develop resistance to antifungal drugs, reducing the effectiveness of treatments and limiting available therapeutic options. 7. Describe the general structure (beta lactam ring) of penicillin and its mode of action against bacteria: Beta-lactam ring – a four-membered ring structure that is crucial for its antibacterial activity. Penicillin – cell wall inhibitor ▪ Inhibiting penicillin-binding proteins (PBPs) involved in the synthesis of the bacterial cell wall (specifically peptidoglycan). ▪ Weakens the cell wall leading to cell lysis and death 8. What is the structural difference between a natural and synthetic penicillin? Natural Penicillin e.g., Penicillin G) – derived directly from the mold Penicillium ▪ Narrow spectrum of activity ▪ Susceptible to penicillinase – enzymes that can inactivate penicillin; produced by certain bacteria. Synthetic Penicillin (e.g., Methicillin, Amoxicillin) ▪ Chemically modified to improve stability ▪ Broaden the spectrum of activity ▪ Or resist degradation by bacterial enzymes like beta-lactamase. 9. What is penicillinase? Aka beta-lactamase is an enzyme produced by some bacteria that breaks the beta- lactam ring of penicillin, rendering it inactive Enzymes that can inactivate penicillin 10. Natural penicillins are sensitive to penicillinase… why? Natural penicillin such as Penicillin G are easily degraded by penicillinase because they lack the structural modifications found in some synthetic penicillin that protect the beta-lactam ring from enzymatic breakdown. 11. Describe why bacteria with capsules are more resistant to antibiotics: Capsules provide a protective outer layer that can shield bacteria from antibiotics and the host’s immune system. ▪ They make it harder for the antibiotic to penetrate and reach the cell wall or membrane. ▪ Capsule can prevent phagocytosis by immune cells, allowing bacteria to survive longer in the host 12. Explain why mycobacteria are highly resistant to antibiotics: Such as Mycobacterium tuberculosis have a unique cell wall structure that includes a thick layer of mycolic acids (waxy lipids). It makes it impermeable to many antibiotics, disinfectants, and even the host’s immune defenses. Slow growth rate also reduces effectiveness of antibiotics that target activity dividing cells 13. Provide an antibiotic example and its mode of action against mycobacteria: Isoniazid (INH) – inhibits the mycolic acid synthesis mycobacteria o Targets mycolic acid synthesis in the cell wall of mycobacteria o It inhibits the enzyme involved in production of mycolic acids o Disrupts the integrity of the cell wall leading to bacterial cell death 14. Describe the disk-diffusion test and broth-dilution test and how they work to evaluate antibiotic: Disk-diffusion test (Kirby-Bauer Test) – paper disk soaked in different antibiotics are placed on an agar plate that has been inoculated with a bacterial culture. ▪ As the bacteria grow, the antibiotics diffuse from the disk into the agar. If an antibiotic ▪ If the antibiotic is effective against the bacteria, it will create a zone of inhibition (clear are with no bacterial growth) around the disk ▪ The size of the zone of inhibition indicates the bacteria’s sensitivity to the antibiotic; larger zones mean higher sensitivity Broth-dilution test – preparing series of broth cultures with decreasing concentrations of the antibiotic. Bacteria are then added to each tube. ▪ After incubation, the minimum inhibitory concentration (MIC) is determined as the lowest concentration of the antibiotic that prevents visible bacterial growth. ▪ The broth-dilution test helps identify the lowest effective dose of an antibiotic needed to inhibit bacterial growth. Compare and contrast synergism and antagonism: ▪ Synergism – when two antibiotics work together to produce a greater effect than the sum of their individual effects. o Using penicillin (which disrupts cell wall synthesis) along with gentamicin (which inhibits protein synthesis) to enhance bacterial killing. ▪ Antagonism – one antibiotic interferes with the action of another reducing its effectiveness. o Example – using bacteriostatic antibiotic like tetracycline can inhibit the action of bactericidal antibiotic like penicillin, which requires actively growing bacteria to work. 15. What is antibiotic resistance? Antibiotic resistance occurs when bacteria evolve mechanisms to survive exposure to antibiotics that would normally kill them or inhibit their growth. ▪ It can develop through genetic mutations or acquiring resistance genes from other bacteria. Factors like overuse, misuse, and incomplete courses of antibiotics contribute to the development and spread of resistance. ▪ It makes infections harder to treat, leading to longer illness, higher medical costs, and increased mortality rates. 16. What is MRSA? MRSA (Methicillin-Resistant Staphylococcus aureus) – a type of bacteria that has developed resistance to methicillin and other common antibiotics, making infections difficult to treat. ▪ Known for causing skin infections and more serious infections like pneumonia or bloodstream infections. Describe three ways bacteria can obtain genes for antibiotic resistance: a) Conjugation – transfer of resistance genes between bacteria through direct cell-to-cell contact using structures like pili. b) Transformation – uptake of free DNA from the environment which can include resistance genes. c) Transduction – transfer of genetic material by bacteriophages (viruses that infect bacteria). 17. Explain how antibiotic resistance can be spread to other cells in a colony. Horizontal degradation of the drug – bacteria can share resistance genes through conjugation, transformation, or transduction. Once one bacterium acquires resistance it can spread these genes to neighboring bacteria increasing the proportion of resistant cells in the population. 18. Describe 4 modes of action for ways bacteria resist (or are resistant to) antibiotics: a) Enzymatic Destruction or Inactivation of the Drug -- Bacteria produce enzymes that chemically break down or modify the antibiotic, rendering it ineffective. ▪ Example: Beta-lactamase is an enzyme that destroys the beta-lactam ring in penicillin and similar antibiotics, making them ineffective. b) Prevention of Penetration to the Target Site Within the Microbe -- Bacteria alter their cell wall or membrane structure to reduce the ability of the antibiotic to enter the cell. ▪ Example: Gram-negative bacteria have an outer membrane that can act as a barrier, preventing certain antibiotics from reaching their targets. c) Alteration of the Drug’s Target Site: ▪ The target molecule within the bacterium (e.g., a ribosome or enzyme) is modified so that the antibiotic can no longer bind effectively. ▪ Example: Some bacteria alter the structure of penicillin-binding proteins (PBPs), making beta-lactam antibiotics unable to bind and inhibit cell wall synthesis. d) Rapid Efflux (Ejection) of the Antibiotic: ▪ Bacteria use efflux pumps to actively transport the antibiotic out of the cell before it can reach its target. ▪ Example: Tetracycline resistance can occur when bacteria develop efflux pumps that expel the drug from the cell. e) Combinations of These Mechanisms: ▪ Bacteria can use multiple resistance mechanisms at once, making them highly resistant to treatment. ▪ Example: A bacterium might produce beta-lactamase, alter its cell wall to prevent antibiotic entry, and use efflux pumps, making it resistant to multiple antibiotics simultaneously. 19. How are we making antibiotic resistance worse? What happens if antibiotics stop working? Contributing factors: ▪ Overprescription of antibiotics ▪ Incomplete courses of treatment (not finishing the entire prescription) ▪ Use of antibiotics in agriculture and animal farming ▪ We are “selecting for” antibiotic resistant bacteria. Consequences of antibiotic failure: ▪ There will be nothing we can do for infection ▪ Common infections and minor injuries could become life-threatening ▪ Medical procedures such as surgeries or cancer treatments would become much riskier due to the increased risk of untreatable infections 20. How are we addressing the problem? Removing some antibiotics from circulation Reverse natural selection process – won’t evolve toward resistance if antibiotic molecule is not there Research to discover new antibiotics molecule (natural or synthetic)