Immunity and Immunization PDF
Document Details
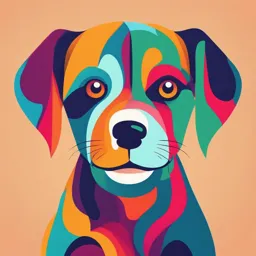
Uploaded by MemorableYtterbium
Tags
Summary
This document provides an introduction to immunology. It explains the concept of immunity as the body's response to foreign substances called antigens, and it describes various immune responses and defenses.
Full Transcript
Phagocytosis Plasma cells Primary follicles Primary lymphoid organs Secondary follicles Secondary lymphoid organs Spleen T lymphocytes Thymocytes Thymus Although humans have been trying for centuries to unravel the secrets of preventing disease, the field of immunology is a relatively new...
Phagocytosis Plasma cells Primary follicles Primary lymphoid organs Secondary follicles Secondary lymphoid organs Spleen T lymphocytes Thymocytes Thymus Although humans have been trying for centuries to unravel the secrets of preventing disease, the field of immunology is a relatively new science. Immunology can be defined as the study of a host’s reactions to foreign substances that are introduced into the body. Such foreign substances that induce a host response are called antigens. Antigens are all around us in nature. They vary from substances, such as pollen, that may make us sneeze to serious bacterial pathogens, such as Staphylococcus aureus or Group A Streptococcus, that can cause life-threatening illnesses. The study of immunology has given us the ability to prevent diseases such as smallpox, polio, diphtheria, and measles through the development of vaccines. In addition, understanding how the immune system works has made successful organ transplantation possible and has given us new tools to treat diseases such as cancer and autoimmune diseases. Immunological techniques have affected testing in many areas of the clinical laboratory and allowed for such testing to be more precise and automated. Thus, the study of immunology is important to many areas of medicine. We begin this chapter by providing a brief look at the history of immunology. An introduction to the cells and tissues of the immune system follows to help the student form a basis for understanding how the immune response works. In later chapters, we will apply this knowledge to principles of testing for specific diseases. Immunity and Immunization Immunology as a science has its roots in the study of immunity: the condition of being resistant to infection. The first recorded attempts to deliberately induce immunity date back to the 15th century when people living in China and Turkey inhaled powder made from smallpox scabs in order to produce protection against this dreaded disease. The hypothesis was that if a healthy individual was exposed as a child or a young adult, the effects of the disease would be minimized. However, rather than providing protection, the early exposure had a fatality rate of 30%. Further refinements did not occur until the late 1700s when an English country doctor by the name of Edward Jenner was able to successfully prevent infection with smallpox by injecting a less harmful substance—cowpox— from a disease affecting cows. Details of the development of this first vaccine can be found in Chapter 25. The next major development in disease prevention did not occur until almost a hundred years later when Louis Pasteur, often called the “father of immunology,” observed by chance that older bacterial cultures accidentally left out on a laboratory bench for the summer would not cause disease when injected into chickens (Fig. 1–1). Subsequent injections of more virulent organisms had no effect on the birds that had been previously exposed to the older cultures. In contrast, chickens that were not exposed to the older cultures died after being injected with the new fresh cultures. In this manner, the first attenuated vaccine was discovered; this event can be considered the birth of immunology. Attenuation, or change, means to make a pathogen less virulent; it takes place through heat, aging, or chemical means. Attenuation remains the basis for many of the immunizations that are used today. Pasteur applied this same principle of attenuation to the prevention of rabies in exposed individuals. He was thus the first scientist to introduce the concept that vaccination could be applied to any microbial disease. Innate Versus Adaptive Immunity In the late 1800s, scientists began to identify the actual mechanisms that produce immunity in a host. Élie Metchnikoff, a Russian scientist, observed under a microscope that foreign objects introduced into transparent starfish larvae became surrounded by motile amoeboid-like cells that attempted to destroy the penetrating objects. This process was later termed phagocytosis, meaning “cells that eat cells.” He hypothesized that immunity to disease was based on the action of these scavenger cells and was a natural, or innate, host defense. He was eventually awarded a Nobel Prize for his pioneering work. FIGURE 1-1 Louis Pasteur. (Courtesy of the National Library of Medicine.) Other researchers contended that noncellular elements in the blood were responsible for protection from microorganisms. Emil von Behring demonstrated that diphtheria and tetanus toxins, which are produced by specific microorganisms as they grow, could be neutralized by the noncellular portion of the blood, or serum, of animals previously exposed to the microorganisms. Von Behring was awarded the first Nobel Prize in Physiology for his work with serum therapy. The theory of humoral immunity was thus born and sparked a long-lasting dispute over the relative importance of cellular immunity versus humoral immunity. In 1903, an English physician named Almroth Wright linked the two theories by showing that the immune response involved both cellular and humoral elements. He observed that certain humoral, or circulating, factors called opsonins acted to coat bacteria so that they became more susceptible to ingestion by phagocytic cells. These serum factors include specific proteins known as antibodies, as well as other factors called acute-phase reactants that increase nonspecifically in any infection. Antibodies are serum proteins produced by certain lymphocytes when exposed to a foreign substance, and they react specifically with that foreign substance (see Chapter 5). These discoveries showed that there were two major branches of immunity, currently referred to as innate immunity and adaptive immunity. Innate, or natural immunity, is the individual’s ability to resist infection by means of normally present body functions. Innate defenses are considered nonadaptive or nonspecific and are the same for all pathogens or foreign substances to which one is exposed. No prior exposure is required and the response lacks memory and specificity, but the effect is immediate. Many of these mechanisms are subject to influence by such factors as nutrition, age, fatigue, stress, and genetic determinants. Adaptive immunity, in contrast, is a type of resistance that is characterized by specificity for each individual pathogen, or microbial agent, and the ability to remember a prior exposure. Memory and specificity result in an increased response to that pathogen upon repeated exposure, something that does not occur in innate immunity. Both systems are necessary to maintain good health. In fact, they operate in combination and depend on one another for maximal effectiveness. Certain key cells are considered essential to both systems, and they will be discussed next. Cells of the Innate Immune System Leukocytes in Peripheral Blood White blood cells (WBCs), or leukocytes, in the peripheral blood play a key role in both innate and adaptive immunity. Leukocytes defend against invasion by bacteria, viruses, fungi, and other foreign substances. There are five principal types of leukocytes in peripheral blood: neutrophils, eosinophils, basophils, monocytes, and lymphocytes. The first four types are all part of innate immunity. Because lymphocytes are considered part of adaptive immunity, they will be considered in a separate section. Several cell lines that are found in the tissues, namely, mast cells, macrophages, and dendritic cells, will also be discussed in this chapter because they all contribute to the process of immunity. All blood cells arise from a type of cell called a hematopoietic stem cell (HSC). Approximately one and one-half billion WBCs are produced in the bone marrow daily. To form WBCs, the HSC gives rise to two distinct types of precursor cells: common myeloid precursors (CMPs) and common lymphoid precursors (CLPs). CMPs give rise to the WBCs that participate in phagocytosis, which are known as the myeloid line. Phagocytic cells are key to innate immunity, but they are also important in processing antigens for the adaptive response. Lymphocytes arise from CLPs and form the basis of the adaptive immune response. Mature lymphocytes are found in the tissues as well as in peripheral blood. Refer to Figure 1–2 for a simplified scheme of blood cell development, known as hematopoiesis. Neutrophils The neutrophil, or polymorphonuclear neutrophilic (PMN) leukocyte, represents approximately 50% to 70% of the total peripheral WBCs in adults. These cells are around 10 to 15 µm in diameter with a nucleus that has between two and five lobes, which are connected by thin, threadlike filaments (Fig. 1–3). Hence, they are often called segmented neutrophils, or “segs.” They contain a large number of neutral staining granules when stained with Wright stain, two-thirds of which are specific granules; the remaining one-third are called azurophilic granules. Azurophilic or primary granules contain antimicrobial products such as myeloperoxidase, lysozyme, elastase, proteinase-3, cathepsin G, and defensins, which are small proteins that have antibacterial activity. Specific granules, also known as secondary granules, contain lysozyme, lactoferrin, collagenase, gelatinase, and components essential for the oxidative burst. See Chapter 2 for a discussion of the oxidative burst, which takes place during phagocytosis. The main function of neutrophils is phagocytosis, resulting in the destruction of foreign particles. FIGURE 1-2 Simplified scheme of hematopoiesis. In the marrow, HSCs give rise to two different lines—a CLP and a CMP. CLPs give rise to T/NK progenitors, which differentiate into T and NK cells, and to B-cell progenitors, which become B cells and dendritic cells. The CMP differentiates into another type of dendritic cell, monocytes/macrophages, neutrophils, eosinophils, basophils, erythrocytes, and platelets. FIGURE 1-3 Neutrophils. (From Harmening D. Clinical Hematology and Fundamentals of Hemostasis. 5th ed. Philadelphia, PA: F.A. Davis; 2009: Fig. 1–4.) Normally, half of the total neutrophil population in peripheral blood is found in a marginating pool adhering to blood vessel walls, whereas the rest of the neutrophils circulate freely for approximately 6 to 8 hours. There is a continuous interchange, however, between the marginating and the circulating pools. Margination occurs to allow neutrophils to move from the circulating blood to the tissues through a process known as diapedesis, or movement through blood vessel walls. They are attracted to a specific area by chemotactic factors. Chemotaxins are chemical messengers that cause cells to migrate in a particular direction. Once in the tissues, neutrophils have a life span of up to several days. Normally, the influx of neutrophils from the bone marrow equals the output from the blood to the tissues to maintain a steady state. However, in the case of acute infection, an increase of neutrophils in the circulating blood can occur almost immediately. These cells are then driven rapidly to the site of an infection. Eosinophils Eosinophils are approximately 10 to 15 µm in diameter and normally make up between 1% and 4% of the circulating WBCs in a nonallergic person. Their number increases in an allergic reaction or in response to certain parasitic infections. The nucleus is usually bilobed or ellipsoidal and is often eccentrically located (Fig. 1–4). Eosinophils take up the acid eosin dye, and the cytoplasm is filled with large orange to reddish-orange granules. Granules in eosinophils, which are spherical and evenly distributed throughout the cell, contain a large number of previously synthesized proteins, including eosinophil-derived neurotoxin, peroxidase, histamine, proteases, cytokines (chemical messengers), growth factors, and cationic proteins. FIGURE 1-4 Eosinophil. (From Harmening D. Clinical Hematology and Fundamentals of Hemostasis. 5th ed. Philadelphia, PA: F.A. Davis; 2009: Fig. 1–6.) Eosinophils are capable of phagocytosis but are much less efficient than neutrophils because they are present in smaller numbers and they lack digestive enzymes. Eosinophils are able to neutralize basophil and mast cell products. In addition, they can use cationic proteins released during degranulation to damage cell membranes and kill larger parasites, such as helminth worms, that cannot be phagocytized. (See Chapter 22 for details.) However, the most important role of eosinophils is regulation of the adaptive immune response through cytokine release. Basophils Basophils are the least numerous of the WBCs found in peripheral blood, representing less than 1% of all circulating WBCs. The smallest of the granulocytes, basophils are slightly larger than red blood cells (RBCs) (between 10 to 15 µm in diameter) and contain coarse, densely staining deep-bluish-purple granules that often obscure the bilobed nucleus (Fig. 1– 5). Constituents of these granules include histamine, cytokines, growth factors, and a small amount of heparin, all of which have an important function in inducing and maintaining allergic reactions. Histamine contracts smooth muscle, and heparin is an anticoagulant. In addition, basophils regulate some T-helper (Th) cell responses and stimulate B cells to produce the antibody immunoglobulin E (IgE). Basophils have a short life span of only a few hours in the bloodstream; they are then removed and destroyed by macrophages in the spleen. Monocytes Monocytes are the largest cells in the peripheral blood, with a diameter that can vary from 12 to 20 µm (the average is 18 µm). One distinguishing feature of monocytes is an irregularly folded or horseshoe-shaped nucleus that occupies almost one-half of the entire cell’s volume (Fig. 1–6). The abundant cytoplasm stains a dull grayish blue and has a ground-glass appearance because of the presence of fine, dustlike granules. These granules are actually of two types. The first type contains peroxidase, acid phosphatase, and arylsulfatase, indicating that these granules are similar to the lysosomes of neutrophils. The second type of granule may contain β- glucuronidase, lysozyme, and lipase, but no alkaline phosphatase. Digestive vacuoles may also be observed in the cytoplasm. Monocytes make up between 2% and 10% of total circulating WBCs; however, they do not remain in the circulation for long. They stay in peripheral blood for up to 30 hours; then, they migrate to the tissues and become known as macrophages. FIGURE 1-5 Basophil. (From Harmening D. Clinical Hematology and Fundamentals of Hemostasis. 5th ed. Philadelphia, PA: F.A. Davis; 2009: Fig. 1–7.) FIGURE 1-6 Two monocytes. (From Harmening D. Clinical Hematology and Fundamentals of Hemostasis. 5th ed. Philadelphia, PA: F.A. Davis; 2009: Fig. 1–13.) Tissue Cells Macrophages All macrophages arise from monocytes, which can be thought of as macrophage precursors, because additional differentiation and cell division take place in the tissues. The transition from monocyte to macrophage in the tissues is characterized by progressive cellular enlargement to between 25 and 80 µm. Unlike monocytes, macrophages contain no peroxidase. Tissue distribution appears to be a random phenomenon. Macrophages have specific names according to their particular tissue location. Macrophages in the lungs are called alveolar macrophages; in the liver, Kupffer cells; in the brain, microglial cells; in the bone, osteoclasts; and in connective tissue, histiocytes. Macrophages may not be as efficient as neutrophils in phagocytosis because their motility is slow compared with that of the neutrophils. Some macrophages progress through the tissues by means of amoeboid action, whereas others are immobile. However, their life span appears to be in the range of months rather than days. Macrophages play an important role in initiating and regulating both innate and adaptive immune responses. Their innate immune functions include phagocytosis, microbial killing, anti-tumor activity, intracellular parasite eradication, and secretion of cell mediators. Killing activity is enhanced when macrophages become “activated” by contact with microorganisms or cytokines that are released by certain lymphocytes during the immune response. (See Chapter 6 for a complete discussion of cytokines.) Macrophages play a major role in the adaptive immune response by presenting phagocytosed antigens to T lymphocytes. Mast Cells Tissue mast cells resemble basophils, but they come from a different lineage. Mast cells are distributed throughout the body in a wide variety of tissues, such as skin, connective tissue, and the mucosal epithelial tissue of the respiratory, genitourinary, and digestive tracts. Mast cells are larger than basophils, with a diameter of up to 20 µm, and have a small ovoid nucleus with many granules (Fig. 1–7). Unlike basophils, they have a long life span of between 9 and 18 months. The enzyme content of the granules in mast cells helps to distinguish them from basophils because they contain serine proteases, heparin, and neutrophil chemotactic factor, as well as histamine. Mast cells act to increase vascular permeability and increase blood flow to the affected area. Mast cells also play a role in allergic reactions, as well as functioning as antigen-presenting cells (APCs). Because of their versatility, mast cells function as a major conduit between the innate and adaptive immune systems. Dendritic Cells Dendritic cells are so named because they are covered with long, membranous extensions that resemble nerve cell dendrites. They were discovered by Steinman and Cohn in 1973. Progenitors in the bone marrow give rise to dendritic cell precursors that travel to lymphoid as well as nonlymphoid tissue. They are classified according to their tissue location in a similar manner to macrophages. After capturing an antigen in the tissue by phagocytosis or endocytosis, dendritic cells travel to the nearest lymph node and present the antigen to T lymphocytes to initiate the adaptive immune response in a similar way as macrophages. Dendritic cells, however, are considered the most effective APC in the body, as well as the most potent phagocytic cell. Cells of the Adaptive Immune System The key cell involved in the adaptive immune response is the lymphocyte. Lymphocytes represent between 20% and 40% of the circulating WBCs. The typical small lymphocyte is similar in size to RBCs (7 to 10 µm in diameter) and has a large rounded nucleus that may be somewhat indented. The nuclear chromatin is dense and tends to stain a deep blue (Fig. 1–8). Cytoplasm is sparse, containing few organelles and no specific granules, and consists of a narrow ring surrounding the nucleus. The cytoplasm stains a lighter blue. These cells are unique because they arise from an HSC and then are further differentiated in the primary lymphoid organs, namely, the bone marrow and the thymus. Lymphocytes can be divided into three major populations—T cells, B cells, and innate lymphoid cells (of which natural killer [NK] cells are the most prominent type)—based on specific functions and the proteins on their cell surfaces. In the peripheral blood of adults, approximately 10% to 20% of lymphocytes are B cells, 61% to 80% are T cells, and 10% to 15% are NK cells. FIGURE 1-7 Mast cell. (From Harmening D. Clinical Hematology and Fundamentals of Hemostasis. 5th ed. Philadelphia, PA: F.A. Davis; 2009: Fig. 1–13.) FIGURE 1-8 Typical lymphocyte found in peripheral blood. (From Harr R. Clinical Laboratory Science Review. 4th ed. Philadelphia, PA: F.A. Davis; 2013: Color Plate 31.) The three types of cells are difficult to distinguish visually. In the laboratory, proteins, or antigens, on cell surfaces can be used to identify each lymphocyte subpopulation. In order to standardize the nomenclature, scientists set up the Human Leukocyte Differentiation Antigens Workshops to relate research findings. Panels of antibodies from different laboratories were used for analysis, and antibodies reacting similarly with standard cell lines were said to define clusters of differentiation (CD). As each antigen, or CD, was found, it was assigned a number. The list of CD designations currently numbers more than 400. Table 1–1 lists some of the most important CD numbers used to identify lymphocytes. B Cells and Plasma Cells B cells are derived from a lymphoid precursor that differentiates to become either a T cell, B cell, or NK cell depending on exposure to different cytokines. B cells remain in the environment provided by bone marrow stromal cells. B-cell precursors go through a developmental process that prepares them for their role in antibody production and, at the same time, restricts the types of antigens to which any one cell can respond. They are able to generate highly specific cell surface receptors through genetic recombination of their immunoglobulin genes (see Chapter 5 for details). The end result is a B lymphocyte programmed to produce a unique antibody molecule. B cells can be recognized by the presence of membrane- bound antibodies of two types, namely, immunoglobulin M (IgM) and immunoglobulin D (IgD). Other surface proteins that appear on the B cell include CD19, CD20, CD21, and class II major histocompatibility complex (MHC) molecules (see Chapter 3). Table 1-1 Surface Markers on T, B, and NK Cells ANTIGEN CELLTYPE FUNCTION CD3 Thymocytes, T cells Found on all T cells; associated with T-cell antigen receptor CD4 Th cells, monocytes, macrophages Identifies Th cells; also found on most Treg cells CD8 Thymocyte subsets, Tc cells Identifies Tc cells CD16 NK cells, macrophages, neutrophils Low affinity Fc receptor for antibody; mediates phagocytosis CD19 B cells, follicular dendritic cells Part of B-cell coreceptor; regulates B-cell development and activation CD20 B cells B-cell activation and proliferation CD21 B cells, follicular dendritic cells Receptor for complement component C3d; part of B-cell coreceptor with CD19 CD56 NK cells, subsets of T cells Cell adhesion Plasma cells represent the most fully differentiated B lymphocyte, and their main function is antibody production. They are not normally found in the blood; rather, they are located in germinal centers in the peripheral lymphoid organs or they reside in the bone marrow. Plasma cells are spherical or ellipsoidal cells between 10 and 20 µm in size that are characterized by the presence of abundant cytoplasmic immunoglobulin and little to no surface immunoglobulin (Fig. 1–9). The nucleus is eccentric or oval with heavily clumped chromatin that stains darkly. An abundant endoplasmic reticulum and a clear, well-defined Golgi zone are present in the cytoplasm. Plasma cells can be long-lived in lymphoid organs and continually produce antibodies. Antibodies are the major contributor to humoral immunity. T Cells T lymphocytes are so named because they differentiate in the thymus. Lymphocyte precursors called thymocytes enter the thymus from the bone marrow through the bloodstream. As they mature, the T cells express unique surface markers that allow them to recognize foreign antigens bound to cell membrane proteins called MHC molecules. T cells have multiple roles in the immune system. They produce cytokines that stimulate B cells to make antibodies, they kill tumor cells and virus-infected cells, and they regulate innate and adaptive immune responses. The processes in which T cells have a primary role are collectively known as cell-mediated immunity. Three main subtypes of T cells can be distinguished according to their unique functions: helper T cells (Th), cytotoxic T cells (Tc), and regulatory T cells (Treg). All T cells possess the CD3 marker on their cell surface, and the T-cell subtypes can be identified by the presence of either CD4 or CD8 as well. T cells bearing the CD4 receptor are mainly either Th or Treg cells, whereas the CD8-positive (CD8+) population consists of Tc cells. The ratio of CD4+ to CD8+ cells is approximately 2:1 in peripheral blood. Th cells help B cells to make antibody, Tc cells kill virally infected cells and tumor cells, and Treg cells help to control the actions of other T cells. FIGURE 1-9 A typical plasma cell. (From Harmening D. Clinical Hematology and Fundamentals of Hemostasis. 5th ed. Philadelphia, PA: F.A. Davis; 2009: Fig. 1–47.) Innate Lymphoid Cells and Natural Killer Cells The innate lymphoid cells are a family of related cells that have important roles in innate immunity and tissue remodeling. These cells share three main properties: (1) They have a lymphoid morphology, (2) they do not possess antigen-specific receptors, and (3) they do not have myeloid and dendritic cell markers. A principal type of innate lymphoid cell is the natural killer (NK) cell. NK cells are so named because they have the ability to kill target cells without prior exposure to them. NK cells do not require the thymus for development but appear to mature in the bone marrow itself. NK cells are generally larger than T cells and B cells, at approximately 15 µm in diameter, and contain kidney-shaped nuclei with condensed chromatin and prominent nucleoli. Described as large granular lymphocytes, NK cells make up 10% to 15% of the circulating lymphoid pool and are found mainly in the liver, spleen, and peripheral blood. There are no surface markers that are unique to NK cells, but they express a specific combination of antigens that can be used for identification. Two such antigens are CD16 and CD56. CD16 is a receptor for the antigen-nonspecific part of antibody molecules. (See Chapter 5 for more details.) Because of the presence of CD16, NK cells are able to make contact with and then lyse any cell coated with antibodies (see Chapter 2). NK cells continuously scan for protein irregularities on host cells, and they represent the first line of defense against virally infected cells and tumor cells. NK cells are also capable of recognizing foreign cells and destroying them. Granules found in NK cells contain serine proteases called granzymes, and release of these enzymes causes target cell death. Although NK cells have traditionally been considered part of the innate immune system because they can respond to a variety of antigens, they are thought to play an important role as a transitional cell that bridges the innate and the adaptive immune responses against pathogens. Organs of the Immune System Just as the cells of the immune system have diverse functions, so, too, do key organs that are involved in the development of the immune response. The bone marrow and thymus are considered the primary lymphoid organs where maturation of B lymphocytes and T lymphocytes takes place, respectively. The secondary lymphoid organs provide a location where contact with foreign antigens can occur (Fig. 1–10). Secondary lymphoid organs include the spleen, lymph nodes, and various types of mucosal- associated lymphoid tissues (MALT). The primary and secondary organs are differentiated according to their function in both adaptive and innate immunity. Primary Lymphoid Organs Bone Marrow Bone marrow is considered one of the largest tissues in the body, and it fills the core of all long flat bones. It is the main source of HSCs, which develop into erythrocytes, granulocytes, monocytes, platelets, and lymphocytes. Each of these lines has specific precursors that originate from the pluripotent stem cells. Some lymphocyte precursors remain in the marrow to mature and become NK cells or B cells. B cells received their name because they were originally found to mature in birds in an organ called the bursa of Fabricius, which is similar to the appendix in humans. After searching for such an organ in humans, it was discovered that B-cell maturation takes place within the bone marrow itself. Thus, the naming of these cells was appropriate. Other lymphocyte precursors go to the thymus and develop into T cells, so named because of where they mature. Immature T cells appear in the fetus as early as 8 weeks in the gestational period. Thus, differentiation of lymphocytes appears to take place very early in fetal development and is essential to acquisition of immunocompetence by the time the infant is born. FIGURE 1-10 Sites of lymphoreticular tissue. Primary organs include the bone marrow and the thymus. Secondary organs are distributed throughout the body and include the spleen, lymph nodes, and MALT. The spleen filters antigens in the blood, whereas the lymphatic system filters fluid from the tissues. Thymus T cells develop their identifying characteristics in the thymus, which is a small, flat, bilobed organ found in the thorax, or chest cavity, right below the thyroid gland and overlying the heart. In humans, the thymus reaches a weight of 30 to 40 g by puberty and then gradually shrinks in size. It was first thought that the thymus produces enough virgin T lymphocytes early in life to seed the entire immune system, making the organ unnecessary later on. However, it now appears that although the thymus diminishes in size as humans age, it is still capable of producing T lymphocytes, although at a reduced rate. Each lobe of the thymus is divided into smaller lobules filled with epithelial cells that play a central role in the differentiation process. Maturation of T cells takes place during a 3-week period as cells filter through the thymic cortex to the medulla. Different surface antigens are expressed as T cells mature. In this manner, a repertoire of T cells is created to protect the body from foreign invaders. Mature T lymphocytes are then released from the medulla. Secondary Lymphoid Organs Once lymphocytes mature in the primary organs, they are released and make their way to secondary lymphoid organs, which include the spleen, lymph nodes, cutaneous-associated lymphoid tissue (CALT), and MALT in the respiratory, gastrointestinal, and urogenital tracts. It is within these secondary organs that the main contact with foreign antigens takes place. Lymphocyte circulation between the secondary organs is complex and is regulated by different cell surface adhesion molecules and by cytokines. Each lymphocyte spends most of its life span in solid tissue, entering the circulation only periodically to go from one secondary lymphoid organ to another. Lymphocytes in these organs travel through the tissue and then return to the bloodstream by way of the thoracic duct. The thoracic duct is the largest lymphatic vessel in the body. It collects most of the body’s lymph fluid and empties it into the left subclavian vein. The majority of circulating lymphocytes are T cells. Continuous recirculation increases the likelihood of a T lymphocyte coming into contact with the specific antigen with which it can react. Lymphocytes are segregated within the secondary lymphoid organs according to their particular functions. T lymphocytes are effector cells that serve a regulatory role, whereas B lymphocytes produce antibodies. It is in the secondary lymphoid organs that contact of the B and T lymphocytes with foreign antigens is most likely to take place. Lymphopoiesis, or multiplication of lymphocytes, occurs in the secondary lymphoid tissues and is strictly dependent on antigenic stimulation. Formation of lymphocytes in the bone marrow, however, is antigen-independent, meaning that lymphocytes are constantly being produced without the presence of specific antigens. Most naïve or resting lymphocytes die within a few days after leaving the primary lymphoid organs unless activated by the presence of a specific foreign antigen. Antigen activation gives rise to long-lived memory cells and shorter-lived effector cells that are responsible for the generation of the immune response. Spleen The spleen, the largest secondary lymphoid organ, has a length of approximately 12 cm and weighs 150 g in the adult. It is located in the upper-left quadrant of the abdomen just below the diaphragm and is surrounded by a thin capsule of connective tissue. The organ can be characterized as a large discriminating filter, as it removes old and damaged cells and foreign antigens from the blood. Splenic tissue can be divided into two main types: red pulp and white pulp. The red pulp makes up more than one-half of the total volume, and it is rich in macrophages. The major function of the red pulp is to destroy old RBCs, platelets, and some pathogens. Blood flows from the arterioles into the red pulp and then exits by way of the splenic vein. The white pulp comprises approximately 20% of the total weight of the spleen and contains the lymphoid tissue, which is arranged around arterioles in a periarteriolar lymphoid sheath (PALS) (Fig. 1–11). This sheath contains mainly T cells. Attached to the sheath are primary follicles, which contain B cells that are not yet stimulated by antigens. Surrounding the PALS is a marginal zone containing dendritic cells that trap antigens. Lymphocytes enter and leave this area by means of the many capillary branches that connect to the arterioles. The spleen receives a blood volume of approximately 350 mL/minute, which allows lymphocytes and macrophages to constantly survey for infectious agents or other foreign matter. Lymph Nodes Lymph nodes serve as central collecting points for lymph fluid from adjacent tissues. Lymph fluid is a filtrate of the blood and arises from the passage of water and low-molecular-weight solutes out of blood vessel walls and into the interstitial spaces between cells. Some of this interstitial fluid returns to the bloodstream through venules, but a portion flows through the tissues and is eventually collected in thin-walled vessels known as lymphatic vessels. Lymph nodes are located along lymphatic ducts and are especially numerous near the joints and where the arms and legs join the body (see Fig. 1–10). FIGURE 1-11 Cross-section of the spleen showing organization of the lymphoid tissue. T cells surround arterioles in the PALS. B cells are just beyond in follicles. When stimulated by antigens, the B cells form germinal centers. All the lymphoid tissue is referred to as the white pulp. Filtration of interstitial fluid from around cells in the tissues is an important function of these organs because it allows contact between lymphocytes and foreign antigens from the tissues to take place. Whereas the spleen helps to protect us from foreign antigens in the blood, the lymph nodes provide the ideal environment for contact with foreign antigens that have penetrated into the tissues. The lymph fluid flows slowly through spaces called sinuses, which are lined with macrophages, creating an ideal location where phagocytosis can take place. The node tissue is organized into an outer cortex, a paracortex, and an inner medulla (Fig. 1–12). Lymphocytes and any foreign antigens present enter nodes via afferent lymphatic vessels. Numerous lymphocytes also enter the nodes from the bloodstream by means of specialized venules called high endothelial venules, which are located in the paracortical areas of the node tissues. The outermost layer, the cortex, contains macrophages and aggregations of B cells in primary follicles similar to those found in the spleen. These are the mature, resting B cells that have not yet been exposed to antigens. Specialized cells called follicular dendritic cells are also located here. These cells exhibit a large number of receptors for antibodies and help to capture antigens to present to T cells and B cells. Secondary follicles consist of antigen-stimulated proliferating B cells. The interior of a secondary follicle is known as the germinal center because it is here that transformation of the B cells takes place. When exposed to an antigen, plasma cells (see Fig. 1–9), which actively secrete antibodies, and memory cells, which can quickly develop into plasma cells, are formed. Thus, the lymph nodes provide an ideal environment for the generation of B-cell memory, or the ability of the immune system to react more quickly to a foreign substance it has already encountered in the past. T lymphocytes are mainly localized in the paracortex, the region between the follicles and the medulla. T lymphocytes are in close proximity to APCs called interdigitating cells. The medulla is less densely populated than the cortex but contains some T cells (in addition to B cells), macrophages, and numerous plasma cells. FIGURE 1-12 Structure of a lymph node. A lymph node is surrounded by a fibrous outer capsule. Right underneath is the subcapsular sinus, where lymph fluid drains from afferent lymphatic vessels. The outer cortex contains collections of B cells in primary follicles. When stimulated by antigens, secondary follicles are formed. T cells are found in the paracortical area. Fluid drains slowly through sinusoids to the medullary region and out the efferent lymphatic vessel to the thoracic duct. Particulate antigens are removed from the fluid as it travels across the node from cortex to medulla. Fluid and lymphocytes exit by way of the efferent lymphatic vessels. Such vessels form a larger duct that eventually connects with the thoracic duct and the venous system. Therefore, lymphocytes are able to recirculate continuously between lymph nodes and the peripheral blood. If contact with an antigen takes place, lymphocyte traffic shuts down. Lymphocytes able to respond to a particular antigen proliferate in the node. Accumulation of lymphocytes and other cells causes the lymph nodes to become enlarged, a condition known as lymphadenopathy. As lymphocyte traffic resumes, recirculation of expanded numbers of lymphocytes occurs. Other Secondary Organs Additional areas of lymphoid tissue include the mucosal-associated tissue known as MALT. MALT is found in the gastrointestinal, respiratory, and urogenital tracts. Some examples include the tonsils; appendix; and Peyer’s patches, a specialized type of MALT located at the lower ileum of the intestinal tract. These mucosal surfaces represent some of the main ports of entry for foreign antigens, and thus, numerous macrophages and lymphocytes are localized here. The skin is considered the largest organ in the body, and the epidermis contains several intraepidermal lymphocytes. Most of these are T cells, which are uniquely positioned to combat any antigens that enter through the skin. In addition, monocytes, macrophages, and dendritic cells are found here. The collective term for these cells is the CALT (cutaneous-associated lymphoid tissue). All these secondary organs function as potential sites for contact with foreign antigens, and they increase the probability of an immune response. Within each of these secondary organs, T and B cells are segregated and perform specialized functions. B cells differentiate into memory cells and plasma cells and are responsible for humoral immunity or antibody formation. T cells play a role in cell-mediated immunity; as such, they produce sensitized lymphocytes that secrete cytokines. As we discussed, both cell-mediated immunity and humoral immunity are key components of the adaptive immune response. SUMMARY Immunology has its roots in the study of immunity—the condition of being resistant to disease. Edward Jenner performed the first vaccination against smallpox by using material from cowpox lesions. Louis Pasteur is considered the father of immunology for his use of attenuated vaccines. Élie Metchnikoff was the first to observe phagocytosis, the process by which cells eat other cells. Immunity has two branches: innate and adaptive. Innate immunity is the ability of the body to resist infection through means of preexisting barriers or nonspecific mechanisms that can be activated quickly. Adaptive immunity is characterized by specificity for antigen, memory, and dependence upon lymphocytes. There are two main types of adaptive immune responses: (1) humoral immunity, which involves production of antibodies by B lymphocytes and plasma cells, and (2) cell-mediated immunity, which is carried out by T lymphocytes to destroy internal pathogens. All blood cells arise from multipotent HSCs in the bone marrow during a process called hematopoiesis. The five principal types of leukocytes are neutrophils, eosinophils, basophils, monocytes, and lymphocytes. Tissue cells involved in immunity include mast cells, dendritic cells, and macrophages that arise from monocytes. Cells that are involved in the innate immune response and are actively phagocytic include neutrophils, monocytes, macrophages, and dendritic cells. Lymphocytes are the key cells involved in the adaptive immune response because they have antigen-specific receptors. CD stands for “clusters of differentiation,” which are proteins found on cell surfaces that can be used for identification of specific cell types and stages of differentiation. B cells are a type of lymphocyte that develop in the bone marrow and are capable of secreting antibody when they mature into plasma cells. They can be identified by the presence of CD19, CD20, and surface antibody. T cells acquire their specificity in the thymus and consist of two subtypes: CD4+, which are mainly Th or Treg cells, and CD8+, which are Tc cells. The CD3 marker is present on all T-cell subtypes. NK cells are lymphocytes that arise from a lymphocyte precursor but do not develop in the thymus. NK cells can kill virally infected or cancerous target cells without previous exposure to them. In humans, the bone marrow and the thymus are considered the primary lymphoid organs where lymphocytes mature. B cells remain in the bone marrow to mature, whereas T cells develop their specific characteristics in the thymus. The secondary lymphoid organs are the sites in which lymphocytes come into contact with foreign antigens and become activated in the adaptive immune response. Secondary lymphoid organs include the spleen, lymph nodes, MALT, and CALT. Study Guide: Cells of the Immune System WHERE CELL TYPE FOUND FUNCTION Neutrophils 50%–70% of First responders to circulating WBCs, infection, also in tissue phagocytosis Eosinophils 1%–4% of circulating Kill parasites, WBCs neutralize basophil and mast cell products, regulate mast cells Basophils Less than 1% of Produce circulating WBCs inflammatory mediators that induce and maintain allergic reactions Mast cells Skin, connective Produce tissue, mucosal inflammatory epithelium mediators that induce and maintain allergic reactions Monocytes 2%–10% of Phagocytosis; circulating WBCs migrate to tissues to become macrophages Macrophages Lungs, liver, brain, Phagocytosis; kill bone, connective intracellular tissue, other parasites; tissues tumoricidal activity; antigen presentation to T cells Dendritic cells Skin, mucous Most potent type of membranes, heart, phagocytic cell; lungs, liver, kidney, most effective at other tissues antigen presentation Lymphocytes 20%–40% of Key cells in adaptive circulating WBCs; immune responses. also found in lymph Major types are T nodes, spleen, cells, B cells, and other secondary NK cells. lymphoid organs B lymphocytes Develop in bone Key role in humoral marrow and immune response. migrate to Possess antibody secondary receptors that bind lymphoid organs. to specific antigen. Found in peripheral Mature into plasma blood and follicles cells. in lymph nodes and spleen Plasma cell Bone marrow; Secrete antibodies germinal centers in into blood and secondary other body fluids lymphoid organs T lymphocytes Mature in thymus. Key role in cell- Also found in mediated immunity. peripheral blood Different subtypes and secondary produce cytokines lymphoid organs. that help or Located in suppress adaptive paracortex in lymph and innate immune nodes and PALS in responses. spleen. NK cells Develop in bone Kill target cells such marrow. Found in as tumors or virus- peripheral blood, infected host cells liver, and spleen. without prior exposure