Activity 1: The Chemical Bond PDF
Document Details
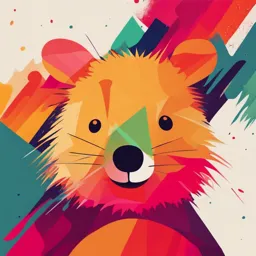
Uploaded by PleasedMeitnerium1911
Agusan del Sur State College of Agriculture and Technology
Tags
Summary
This learning module in chemistry for engineers provides an introduction to chemical bonding. It covers different types of chemical bonds and explores example molecules, along with activities and questions for students.
Full Transcript
LEARNING MODULE IN CHEMISTRY FOR ENGINEERS AGUSAN DEL SUR STATE COLLEGE OF AGRICULTURE AND TECHNOLOGY MAIN CAMPUS, BUNAWAN AGUSAN DEL SUR START ACTIVITY 1: THE CHEMICAL BOND CHEMICAL BONDING AS ART. The Atomium is a110-m-high steel sculpture commis...
LEARNING MODULE IN CHEMISTRY FOR ENGINEERS AGUSAN DEL SUR STATE COLLEGE OF AGRICULTURE AND TECHNOLOGY MAIN CAMPUS, BUNAWAN AGUSAN DEL SUR START ACTIVITY 1: THE CHEMICAL BOND CHEMICAL BONDING AS ART. The Atomium is a110-m-high steel sculpture commissioned for the1958 World’s Fair in Brussels. The nine spheres represent atoms, and the connecting rods evoke the chemical bonds holding them together. One sphere sits in the center of a cube formed by the other eight (8), a common arrangement of the atoms in metallic elements, such as iron. WHENEVER TWO ATOMS OR IONS are strongly held together, we say there is a chemical bond between them. The “Atomium” in Brussels. Answer the following questions on chemical bonding: 1. Atoms that are held together by the attractions between oppositely charged ions have ______. A. ionic bond C. metallic bond B. covalent bond D. polymeric bonds 2. Molecules formed of these atoms by the sharing of electrons between atoms have _______. A. ionic bond C. metallic bond B. covalent bond D. polymeric bonds 3. The spoon consists mainly of iron (Fe) atoms are formed by electrons that are relatively free to move through the objects have ______. A. ionic bond C. metallic bond B. covalent bond D. polymeric bonds 4. What determines the type of bonding in any substance? A. The classification of the element B. The electronic structure of the atom C. The physical and chemical properties D. The phase of the matter involved 5. What do you call of the electrons that are specifically involved in chemical bonding? A. Lone electrons C. Paired electrons B. Valence electrons D. Electron configuration 6. The different types of interactions between atoms lead to different types of chemical bonds. A. True B. False 7. Bonding almost always forms single bonds. A. True B. False 8. How are ionic bonded materials can easily be differentiated from covalent bonded materials? A. interaction between a metal and a non-metal is ionic and that between two non-metals is covalent. B. when covalent bonding is dominant; more often than not we expect compounds to exist as molecules C. when ionic bonding is dominant, we expect the compounds to be brittle and not easily melted solids D. All are true. (Check your answers using Answers Key at the end part of the module.) COLLEGE OF ENGINEERING & INFORMATION TECHNOLOGY ENGINEERING STUDENTS INSTRUCTIONAL MATERIALS DEVELOPMENT © 2024 LEARNING MODULE IN CHEMISTRY FOR ENGINEERS AGUSAN DEL SUR STATE COLLEGE OF AGRICULTURE AND TECHNOLOGY MAIN CAMPUS, BUNAWAN AGUSAN DEL SUR DISCOVER ACTIVITY 2: THE ELEMENT CALLED CARBON (C) For decades, chemistry textbooks said that there were two forms of the element carbon: graphite and diamond. How do graphite and diamond differ in the first place so that observable transformation from one to the other can be affected? Graphite is a raw material for the production of diamonds; but it has also other important applications of its own. It is the black material used in writing with your pencil. It is used as a lubricant in many mechanical applications. Graphite (C) On the other hand, the impressive appearance of cut diamonds is responsible for their most familiar use in jewelry. From an engineering perspective, however, the hardness of the diamond probably represents a more significant property. Diamond drill bits find uses in many areas, including the drilling of oil wells in some locations. A team of chemists at Rice University discovered a new form of Diamond (C) carbon, whose 60 atoms formed a framework that looks like a tiny soccer ball. It was given the whimsical name buckminsterfullerene. The discovery of C60 and other related molecules, now collectively known as the fullerenes, helped usher in a new form of carbon and also a new branch of science—nanotechnology. The story of C60 is an excellent example of the way the study of chemistry affects the development of new materials! Although the small sphere of C60 may not have any immediate Fullerene (C60) uses, manipulation of experimental conditions allows the growth of tubes of carbon, called nanotubes (CNT). Carbon nanotubes grown with a metallic element enclosed within them might represent a way to build wires that are one molecule wide. The implications for miniaturization of electronic devices are among the most immediate and exciting opportunities promised by new nanotechnologies. So scientists and engineers are actively exploring fundamental questions related to Carbon Nanotubes (CNT) nanotechnology. Answer the following questions on the element Carbon: 1. List down at least 6 applications of Carbon Nanotubes (CNT). 2. How can the present times continue to understand the connections between molecular level characteristics and the macroscopic properties of materials? (Check your answers using Answers Key at the end part of the module.) COLLEGE OF ENGINEERING & INFORMATION TECHNOLOGY ENGINEERING STUDENTS INSTRUCTIONAL MATERIALS DEVELOPMENT © 2024 LEARNING MODULE IN CHEMISTRY FOR ENGINEERS AGUSAN DEL SUR STATE COLLEGE OF AGRICULTURE AND TECHNOLOGY MAIN CAMPUS, BUNAWAN AGUSAN DEL SUR LEARN ACTIVITY 3: EXPAND YOUR KNOWLEDGE Kon’nichiwa! It’s your second module with me buddy! Here let’s look deeper into the materials which you will be handling soon in your everyday applications as an engineer. BUT FIRST LET’S answer some questions. LESSON 1: BASIC CONCEPTS OF CRYSTAL STRUCTURES The various forms of carbon that described Activity 2 share one important physical property—they are all solids at normal laboratory temperature and pressure. The vast majority of elements are solids under such conditions. Forces between atoms and molecules certainly play a role in the answer to this question, but the basic structure of condensed phases also contributes to an understanding of their stability. We will begin our treatment from this structural perspective. CLASSIFICATIONS OF SOLIDS We can classify solids according to the molecular forces that hold them together: 1. Metallic solids 2. Ionic solids -are held together by the mutual attraction between cations and anions - are hard but they tend to be brittle 3. Covalent-network solids - are held together by an extended network of covalent bonds 4. Molecular solids - are held together by dispersion forces, dipole-dipole interactions, and hydrogen bonds - because these forces are relatively weak, molecular solids tend to be soft and have low melting points COLLEGE OF ENGINEERING & INFORMATION TECHNOLOGY ENGINEERING STUDENTS INSTRUCTIONAL MATERIALS DEVELOPMENT © 2024 LEARNING MODULE IN CHEMISTRY FOR ENGINEERS AGUSAN DEL SUR STATE COLLEGE OF AGRICULTURE AND TECHNOLOGY MAIN CAMPUS, BUNAWAN AGUSAN DEL SUR STRUCTURE OF SOLIDS The structures of many solids have patterns that repeat over and over in three dimensions. Amorphous solids - The particles of such solids lack an ordered internal structure and are randomly arranged. Classification of solid materials according to their structure and properties. Crystalline solids - the atoms, ions, or molecules are arranged in an orderly repeating pattern. Though both categories include important examples, much more is known about crystalline materials simply because their regular periodic arrays can be studied much more easily with available experimental tools. We will, therefore, focus on crystalline solids in our description in this module. THE CRYSTALLINE SOLIDS UNIT CELLS In a crystalline solid, there is a relatively small repeating unit, called a unit cell, that is made up of a unique arrangement of atoms and embodies the structure of the solid. To understand real crystals, we must move represent in three dimensions. In three dimensions, a lattice is defined by three lattice vectors a, b, and c. Fig. 1 - The 7 three-dimensional primitive lattices. COLLEGE OF ENGINEERING & INFORMATION TECHNOLOGY ENGINEERING STUDENTS INSTRUCTIONAL MATERIALS DEVELOPMENT © 2024 LEARNING MODULE IN CHEMISTRY FOR ENGINEERS AGUSAN DEL SUR STATE COLLEGE OF AGRICULTURE AND TECHNOLOGY MAIN CAMPUS, BUNAWAN AGUSAN DEL SUR These lattice vectors define a unit cell that is a parallelepiped (a six-sided figure whose faces are all parallelograms). If we place a lattice point at each corner of a unit cell, we get a primitive lattice. Shown on the figure 1 on previous page are the seven primitive lattices. The three (3) types of cubic lattices ✓ Primitive cubic lattice - lattice points are placed at each corner of a unit cell ✓ Body-centered cubic lattice - has one lattice point at the center of the unit cell in addition to the lattice points at the eight corners ✓ Face-centered cubic lattice - lattice point at the center of each of the six faces of the unit cell in addition to the lattice points at the eight corners Translating the crystal lattice into a molecular structure is represented by a figure shown below: In the crystal structure, notice that the atoms on the corners and faces of a unit cell do not lie wholly within the unit cell. In primitive cubic cell, the corner and face atoms are shared by neighbouring unit cells. Because a cube has eight (8) corners, each primitive cubic unit cell contains (1/8) x 8 = 1 atom. Similarly, each body-centered cubic unit cell contains two (1/8) x 8 = 1 atoms, from the corners and 1 at the center of the unit cell. In a face-centered cubic lattice, atoms that lie on the face of a unit cell, are shared by two unit cells so that only one-half of the atom belongs to each unit cell. Therefore, a face-centered cubic unit cell contains four atoms, atom from the corners and atoms from the faces. Fraction of Any Atom as a Function of Location within the Unit Cell. COLLEGE OF ENGINEERING & INFORMATION TECHNOLOGY ENGINEERING STUDENTS INSTRUCTIONAL MATERIALS DEVELOPMENT © 2024 LEARNING MODULE IN CHEMISTRY FOR ENGINEERS AGUSAN DEL SUR STATE COLLEGE OF AGRICULTURE AND TECHNOLOGY MAIN CAMPUS, BUNAWAN AGUSAN DEL SUR Packing Efficiency No matter how we arrange the atoms/molecules in a material, there will always be some gaps between them; the atoms/molecules can never completely fill the given volume of the material. But it should also be clear that the amount of empty space among the atoms/molecules could be minimized by carefully arranging them. We can illustrate the differences in the way the atoms/molecules— may be packed by using a two-dimensional model, as shown in Figure on the left, using marbles as an example. It is easy to see that the gaps can be reduced if the rows of marbles are offset from one another. This idea can be quantified by thinking about the packing efficiency of a structure, which represents the percentage of space that is occupied in a given arrangement. The packing efficiency of the atoms will clearly be related to the density of a material because an increase in the packing efficiency will put more atoms into the same volume. Such arrangements are called Close- Packed Structures and are the three- dimensional equivalent of the staggered rows of marbles in the Figure below: Let’s continue with the analogy of stacking marbles or balls into a box and suppose that we have completed a single layer of balls in the close-packed arrangement. When a second layer of balls is added we could place them directly above the balls in the first layer, or we could again offset them so that each ball rests in a gap between balls in the lower layer. Cubic Close Hexagonal Close packing (ccp) packing (hcp) Exercise 1: Calculating Packing Efficiency It is not possible to pack spheres together without leaving some void spaces between the spheres. Packing efficiency is the fraction of space in a crystal that is occupied by atoms. Determine the packing efficiency of a face-centered cubic metal. Solution: A face-centered cubic metal has four atoms per unit cell. Therefore, the volume occupied by the atoms is: For a face-centered cubic metal, the atoms touch along the diagonal of a face of the unit cell: Therefore, a diagonal across a face of the unit cell is equal to 4 times the atomic radius, r. Using simple trigonometry: Finally, we calculate the packing efficiency by dividing the volume occupied by atoms by the volume of the cubic unit cell : COLLEGE OF ENGINEERING & INFORMATION TECHNOLOGY ENGINEERING STUDENTS INSTRUCTIONAL MATERIALS DEVELOPMENT © 2024 LEARNING MODULE IN CHEMISTRY FOR ENGINEERS AGUSAN DEL SUR STATE COLLEGE OF AGRICULTURE AND TECHNOLOGY MAIN CAMPUS, BUNAWAN AGUSAN DEL SUR Do this by yourself! Determine the packing efficiency by calculating the fraction of space occupied by atoms in a body- centered cubic metal. LESSON 2: METALS (Metallic Solids) The bonding in metals is too strong to be due to dispersion forces, and yet there are not enough valence electrons to form covalent bonds between atoms. The bonding, called metallic bonding, results from the fact that the valence electrons are delocalized throughout the entire solid. That is, the valence 14 karat gold electrons are not associated with specific atoms or bonds but are spread throughout the solid. In fact, we can visualize a metal as an array of positive ions immersed in a “sea” of delocalized valence electrons. Metallic solids are also good thermal conductors because the atoms are closely packed together and the delocalized electrons can carry kinetic energy through the solid. Properties of Metallic Solids ✓ a clean metal surface has a characteristic luster (shine) ✓ have a characteristic cold to touch ✓ have high electrical conductivity, which means that electrically charged particles flow easily through them ✓ high electrical conductivity also mean it has high thermal conductivity ✓ most metals are malleable, which means that they can be hammered into thin sheets ✓ most also are ductile, which means that they can be drawn into wires Models of Metallic Bonding The first and simplest explanation of metallic bonding is often referred to as the “sea of electrons” model. The sea of electrons model provides qualitative understanding, but quantitative models for metallic bonding also exist. The most important such model is band theory, and we can use our example of putting metal atoms into a carbon nanotube to explain the origins of this theory. Band Theory Here we examine the bonding in a one-dimensional row of lithium atoms, as might be assembled in a nanotube. In the left-hand panel, we have just two lithium atoms, each of which contributes one valence electron. With four atoms, as in the center panel, we have four electrons. As the number of atoms becomes very large, the available orbitals stack up very close together in energy. With one electron from each atom, the lower half of the available orbitals will be filled, creating the valence band. The higher energy states in the conduction band remain empty COLLEGE OF ENGINEERING & INFORMATION TECHNOLOGY ENGINEERING STUDENTS INSTRUCTIONAL MATERIALS DEVELOPMENT © 2024 LEARNING MODULE IN CHEMISTRY FOR ENGINEERS AGUSAN DEL SUR STATE COLLEGE OF AGRICULTURE AND TECHNOLOGY MAIN CAMPUS, BUNAWAN AGUSAN DEL SUR Band Theory & Conductivity The band structure of a material plays much the same role as atomic orbital energy levels did for atoms. Just as the “Aufbau principle” dictates that electrons occupy the lowest energy orbitals, electrons in bulk materials fill the bands starting at the lowest energy. The highest energy with electron occupation and the energy gap between this energy level and the next available band provide a means for understanding the electrical conductivity of a material. Electrons carry a current by moving through a material, and this motion can be thought of in terms of electrons moving from one orbital to another. - but electrons within a filled band cannot move readily to conduct electricity - to have mobile electrons, we will need to have an unfilled band or portion of a band that is close in energy to a filled band or portion of a band. The figure below shows some general features of the bands for three different classes of materials: metals, semiconductors, and insulators These band energy diagrams point out the key differences among metals, insulators, and semiconductors. The green shaded portions represent energy levels filled with electrons, and the blue portions depict unoccupied energy levels. Differences in conductivity can be explained in terms of the band gap and the relative difficulty of promoting electrons from filled to unfilled levels. METAL ALLOYS DEFINING ALLOYS - An alloy is a material that contains more than one element and has the characteristic properties of a metal. - The alloying of metals is of great importance because it is one of the primary ways of modifying the properties of pure metallic elements. - Example: Steel, involve iron and other metals Bronze is formed by alloying copper + tin Brass is an alloy of copper + zinc Gold in jewellery is gold + other metals COLLEGE OF ENGINEERING & INFORMATION TECHNOLOGY ENGINEERING STUDENTS INSTRUCTIONAL MATERIALS DEVELOPMENT © 2024 LEARNING MODULE IN CHEMISTRY FOR ENGINEERS AGUSAN DEL SUR STATE COLLEGE OF AGRICULTURE AND TECHNOLOGY MAIN CAMPUS, BUNAWAN AGUSAN DEL SUR Alloys can be divided into four (4) categories: ✓ Substitutional alloys ✓ Interstitial alloys ✓ Heterogeneous alloys ✓ Intermetallic compounds Substitutional alloys - are formed when the two metallic components have similar atomic radii and chemical- bonding characteristics. For example: silver and gold Interstitial alloys - are formed when the solute atoms have a much smaller bonding atomic radius than the solvent atoms. - typically, the interstitial element is a non-metal that makes covalent bonds to the neighbouring metal atoms. For example: ✓ Steel - which is much harder and stronger than pure iron, is an alloy of iron that contains up to 3% carbon ✓ Mild steels - contain less than 0.2% carbon; they are malleable and ductile and are used to make cables, nails, and chains ✓ Medium steels - contain 0.2–0.6% carbon; they are tougher than mild steels and are used to make girders and rails ✓ High-carbon steel - used in cutlery, tools, and springs, contains 0.6–1.5% carbon ✓ Stainless steel - which contains about 0.4% carbon, 18% chromium, and 1% nickel Heterogeneous alloys - the metal components here are not dispersed uniformly - properties of heterogeneous alloys depend on both the composition and the manner in which the solid is formed from the molten mixture For example: Pearlite - contains two phases, one phase is essentially pure body-centered cubic iron, and the other Microscopic view of the structure of is the compound Fe3C, known as cementite the heterogeneous alloy pearlite Intermetallic compounds - are naturally compounds rather than mixtures - because they are compounds, they have definite properties and their composition cannot be varied - the ordering of atoms in an intermetallic compound generally leads to better structural stability and higher melting points than what is observed in the constituent metals - these featurescan be attractive for high-temperature applications - on the negative side, intermetallic compounds are often more brittle than substitutional alloys For example: Ni3Al - is a major component of jet aircraft engines Razor blades are often coated with Cr3Pt, which adds hardness, allowing the blade to stay sharp longer. Nb3Sn, is a superconductor, a substance that, when cooled below a critical temperature, conducts electricity with no resistance COLLEGE OF ENGINEERING & INFORMATION TECHNOLOGY ENGINEERING STUDENTS INSTRUCTIONAL MATERIALS DEVELOPMENT © 2024 LEARNING MODULE IN CHEMISTRY FOR ENGINEERS AGUSAN DEL SUR STATE COLLEGE OF AGRICULTURE AND TECHNOLOGY MAIN CAMPUS, BUNAWAN AGUSAN DEL SUR LESSON 3: POLYMERS (Polymeric Solids) In nature, we find many substances of very high molecular weight, running into millions of amu, that make up much of the structure of living organisms and tissues. Some examples are starch and cellulose, which abound in plants, as well as proteins, which are found in both plants and animals. DEFINING POLYMERS In 1827 Jons Jakob Berzelius coined the word polymer (from the Greek “polys,” which means many, and “meros,” which means parts) to denote molecular substances of high molecular weight formed by the polymerization (joining together) of monomers, molecules with low molecular weight. A polymer is a large chain molecule of high molecular weight that is composed of a single molecule (monomer) that is repeated many times in the chain. ▪ Natural polymers – materials taken from nature and processed into usable materials. Examples: Wool, Leather, Silk, and Natural rubber ▪ Synthetic polymers – man-made materials by polymerizing monomers through controlled chemical reactions. Examples: ✓ Plastics - materials formed into various shapes, usually by the application of heat and pressure. ▪ Thermoplastic ▪ Thermosetting plastic – plastic materials that can be - shaped through irreversible chemical reshaped processes and, therefore, cannot be reshaped readily Example: plastic milk containers Example: nylon, polyester ✓ Elastomer - material that exhibits rubbery or elastic behaviour. When subjected to stretching or bending, an elastomer regains its original shape upon removal of the distorting force, if it has not been distorted beyond some elastic limit. ✓ Rubber is the most familiar example of an elastomer. HOW POLYMERS ARE MADE? ADDITION POLYMERIZATION (a.k.a Chain reaction polymerization) - The polymers are made by coupling the monomers through their multiple bonds Stage of reaction: ✓ INITIATION - In this reaction, the double bond in each ethylene molecule “opens up,” and two of the electrons originally in this bond are used to form new single bonds with two other ethylene molecules. ✓ PROPAGATION – each single monomer create a bond and form a chain. ✓ TERMINATION – this stage stops the growth of the chain as desired properties are achieved. COLLEGE OF ENGINEERING & INFORMATION TECHNOLOGY ENGINEERING STUDENTS INSTRUCTIONAL MATERIALS DEVELOPMENT © 2024 LEARNING MODULE IN CHEMISTRY FOR ENGINEERS AGUSAN DEL SUR STATE COLLEGE OF AGRICULTURE AND TECHNOLOGY MAIN CAMPUS, BUNAWAN AGUSAN DEL SUR We can write the equation for the polymerization reaction as follows: Here n represents the large number—ranging from hundreds to many thousands—of monomer molecules (ethylene in this case) that react to form one polymer molecule. Polyethylene is an important material; its annual production exceeds 170 billion pounds each year. Although its composition is simple, the polymer is not easy to make. The right manufacturing conditions were identified only after many years of research. Today many forms of polyethylene, varying widely in physical properties, are known. Polymers of other chemical compositions provide still greater variety in physical and chemical properties. Several other common polymers obtained by addition polymerization are listed below: COLLEGE OF ENGINEERING & INFORMATION TECHNOLOGY ENGINEERING STUDENTS INSTRUCTIONAL MATERIALS DEVELOPMENT © 2024 LEARNING MODULE IN CHEMISTRY FOR ENGINEERS AGUSAN DEL SUR STATE COLLEGE OF AGRICULTURE AND TECHNOLOGY MAIN CAMPUS, BUNAWAN AGUSAN DEL SUR DEGREE OF POLYMERIZATION At chain termination, the polymer molecule stops from growing longer. Because the occurrence of these termination steps is somewhat random, not all of the polymer chains will grow to the same length. Instead, there is normally a range of lengths and hence a distribution of molecular masses. This distribution can be roughly described in terms of the degree of polymerization, which is the average number of repeating units in the polymer. This is usually calculated in terms of mass: One reason that polymers are such common engineering materials is that their properties can be tailored in a number of ways. The most obvious is the choice of the monomer unit because we know that different polymers can offer vastly different characteristics as shown in Table 3. CONDENSATION POLYMERIZATION (a.k.a. Step-Reaction polymerization) - These polymers are made with two functional groups on the monomer, which can react together to form small molecules. - Those small molecules then split off, and the remaining parts of the two monomers are joined together. Example: Condensation polymerization - an amine (R¬NH2) reacts with a carboxylic acid (R¬COOH) to form a bond between N and C plus an H2O molecule TYPES OF POLYMERS The chemical and physical properties of a polymer mainly depend on the type of monomer or monomers used to form the polymer. Based on the number of different types of monomers used to form a polymer molecule, there are two types of polymers: o HOMOPOLYMERS - is formed by a single type of monomer, thus, it consists of only one type of repeating unit. - they are usually made by a polymerization technique called addition polymerization. o COPOLYMERS - A polymer formed from more than one type of monomer is called a copolymer, thus, it contains two or more different types of repeating units. - Usually, most of the copolymers are formed through a process called condensation polymerization. COLLEGE OF ENGINEERING & INFORMATION TECHNOLOGY ENGINEERING STUDENTS INSTRUCTIONAL MATERIALS DEVELOPMENT © 2024 LEARNING MODULE IN CHEMISTRY FOR ENGINEERS AGUSAN DEL SUR STATE COLLEGE OF AGRICULTURE AND TECHNOLOGY MAIN CAMPUS, BUNAWAN AGUSAN DEL SUR - Types of copolymers are hugely dependent on the arrangements of the alternating monomer components as shown below: STRUCTURES & PHYSICAL PROPERTIES OF POLYMERS 1. DEGREE OF CRYSTALLINITY - The fraction of the ordered molecules in the polymer is characterized by the degree of crystallinity, which typically ranges between 10% and 80% - Higher values are only achieved in materials having small molecules, which are usually brittle, or in samples stored for a long time at temperatures just under the melting point. 2. CROSSLINKING - is the general term for the process of forming covalent bonds or relatively short sequences of chemical bonds to join two polymer chains together. - The greater the number of cross-links, the more rigid the polymer. LESSON 4: ENGINEERED NANOMATERIALS DEFINING ENGINEERED NANOMATERIALS Engineered nanomaterials are chemical substances or materials that are engineered with particle sizes between 1 to 100 nanometers in at least one dimension. It is well established that engineered nanomaterials derive many functional advantages from their unique physical and chemical properties. Properties for which nanomaterials are engineered and exploited are: particle size, surface area and surface reactivity. However, such properties also influence their inherent hazard and potentially threaten the health of workers, communities and the environment. In general, engineered nanomaterials should not be considered a safer substitute to toxic chemicals. COLLEGE OF ENGINEERING & INFORMATION TECHNOLOGY ENGINEERING STUDENTS INSTRUCTIONAL MATERIALS DEVELOPMENT © 2024 LEARNING MODULE IN CHEMISTRY FOR ENGINEERS AGUSAN DEL SUR STATE COLLEGE OF AGRICULTURE AND TECHNOLOGY MAIN CAMPUS, BUNAWAN AGUSAN DEL SUR NANOSCALE SEMICONDUCTORS - On the nanoscale, the properties and behaviour of semiconductors may depend dramatically on the dimensionality of the material, structure or device. This offers an attractive means of influencing the system leading to novel physical properties. - minimizing the size of semiconductor materials has been shown to maximize the performance of semiconductors for their application in a wide range of material applications: 1. Quantum size effect (QSE) o arises as a result of the increased quantum confinement of the electrons and holes following the increased reduction in size of small crystalline structures, such as that which occurs during the nanoscaling of semiconductor materials o when present in this intermediate state, the individual energy density of states (DOS) of valence and conduction bands in metals and semiconductors undergo a unique transformation that leads to the spatial enclosure of charge carriers within these structures 2. Widened Bandgap Advantages o nanoscale semiconductors often exhibit a widened band gap that provides these materials with a unique chemical stability at high operating temperatures o this thermal stability therefore provides a wide range of advantages to systems equipped with nanoscale semiconductors, especially when compared to similar silicon-based devices. Challenges of Nanoscale semiconductors While this modification in the physical properties of nanomaterials has allowed for the development of increasingly strong materials, such as graphene, material chemistry researchers often struggle with the ability to determine the correlation between the physical properties that may arise with each newly created physical dimension. To this end, each component of a nanostructure must be carefully scaled in order to achieve the perfect combination of material compositions and length scales that will be appropriate for the final product of interest. NANOSCALE METALS - Nanoscale metal particles have been the subject of intense research over the past 20 years, especially because of their unusual optical, magnetic, and catalytic properties. - Other physical and chemical properties of metallic nanoparticles are also different from the properties of the bulk materials. Gold particles less than 20 nm in diameter melt at a far lower temperature than bulk gold, for instance, and when the particles are between 2 and 3 nm in diameter, gold is no longer a “noble,” unreactive metal; in this size range it becomes chemically reactive. - At nanoscale dimensions, silver has properties analogous to those of gold in its beautiful colors, although it is more reactive than gold. - Currently, there is great interest in research laboratories around the world in taking advantage of the unusual optical properties of metal nanoparticles for applications in biomedical imaging and chemical detection. FULLERENE, CARBON NANOTUBES, GRAPHENE - Until the mid-1980s, pure solid carbon was thought to exist in only two forms: the covalent- network solids diamond and graphite. - However, since 1985, many forms of carbons allotropes were discovered and taken to various applications. Allotropy is the property of some chemical elements to exist in two or more different forms, or allotropes, when found in nature. There are several new allotropes of carbon. COLLEGE OF ENGINEERING & INFORMATION TECHNOLOGY ENGINEERING STUDENTS INSTRUCTIONAL MATERIALS DEVELOPMENT © 2024 LEARNING MODULE IN CHEMISTRY FOR ENGINEERS AGUSAN DEL SUR STATE COLLEGE OF AGRICULTURE AND TECHNOLOGY MAIN CAMPUS, BUNAWAN AGUSAN DEL SUR Allotropes of Carbon a) Diamond b) Graphite c) Lonsdaleite d) C60 (Fullerene buckyball) e) C540 f) C70 g) Amorphous carbon h) Single-walled carbon nanotube (buckytube) Diamond is probably the most well-known carbon allotrope. Graphite is the most stable form of carbon under standard conditions and is used in thermochemistry as the standard state for defining the heat of formation of carbon compounds. Lonsdaleite, also called hexagonal diamond, is an allotrope of carbon with a hexagonal lattice, as opposed to the cubical lattice of conventional diamond. FULLERENE (C60) ✓ The fullerenes are constituted by a network formed by 12 pentagons and 20 hexagons, closing the sphere. ✓ Each carbon of a fullerene is sp hybridized in the form of a sigma bond (simple bond) with three other carbon atoms, subtracting one electron from each carbon. ✓ The most important property of Fullerenes is its high symmetry. APPLICATIONS of C60 1. Fullerene as a material for semiconductor technology - Molecular crystal of fullerene is a semiconductor with a bandgap of 1.5 eV ~ and its characteristics are almost similar to those of other semiconductors. It has conventional applications in electronics such as diode, transistor, photocell, etc. 2. Uses of Fullerenes in Solar Cells - Due to its electronic characteristics, one of the most advanced applications is its use in solar cells. 3. Fullerenes in Electronic Battery - The electronic battery must be emphasized in the midst of other exciting applications which used fullerenes. The basis of these batteries is lithium cathodes. Fullerenes can also be used as additives for synthetic diamonds high- pressure method. It increases the output by 30%. 4. Fullerenes in Fire Retardant Paint - Due to the introduction of fullerenes, paint under the influence of temperature swells in case of fire, there is a fairly dense layer which builds up a couple of times while being heated to a critical temperature of the protected structures. 5. Fullerenes' Medical Applications - Comprehensive research on the biomedical applications of this molecule has been underway since its discovery. 6. Fullerenes' Use in Nanomedicine - Another important field of use is nanomedicine. It is about enclosing beneficial molecules for the organism inside fullerene balls and directing them to bacteria, or cancer cells. 7. Incorporation of fullerenes in the polymers - Due to the incorporation of fullerenes in the polymers, electro-active and optical limiting properties would be achieved. This could mainly be applied in surface coating, conductive devices and in the creation of new molecular networks. COLLEGE OF ENGINEERING & INFORMATION TECHNOLOGY ENGINEERING STUDENTS INSTRUCTIONAL MATERIALS DEVELOPMENT © 2024 LEARNING MODULE IN CHEMISTRY FOR ENGINEERS AGUSAN DEL SUR STATE COLLEGE OF AGRICULTURE AND TECHNOLOGY MAIN CAMPUS, BUNAWAN AGUSAN DEL SUR CARBON NANOTUBES (CNT) ✓ Carbon nanotubes are made in a manner similar to that used to make. ✓ They can be made in two forms: Single-walled CNT - consists of single tubes. It can be 1000 nm long or even longer but is only about 1 nm in diameter. Depending on the diameter of the graphite sheet and how it is rolled up, carbon nanotubes can behave as either semiconductors or metals. Multi-wall CNT - consists of tubes within tubes, nested together APPLICATIONS of CNT CNTs are well-suited for virtually any application requiring high strength, durability, electrical conductivity, thermal conductivity and lightweight properties compared to conventional materials. 1. Additives to various materials - They are added to material manufacturing formulations to offer increased wear resistance and breaking strength, antistatic properties as well as weight reduction. - They are added to: sporting goods(bicycle frames, tennis rackets, hockey sticks, golf clubs and balls, skis, kayaks; sports arrows) yachting (masts, hulls and other parts of sailboats) textiles (antistatic and electrically conducting textiles ('smart textiles'); bullet-proof vests, water-resistant and flame-retardant textiles) automotive, aeronautics and space (light-weight, high-strength structural composites) industrial engineering (e.g. coating of wind-turbine rotor blades, industrial robot arms) 2. Transistors - Single-walled CNT are still regarded as strong candidates for the next generation of high- performance, ultra-scaled and thin-film transistors as well as for opto-electronic devices to replace silicon electronic - the ultrathin body of single-walled carbon nanotubes – only 1 nm in diameter – would allow for excellent transistor behavior even down to the sub-10 nm range 3. Sensors - CNTs are robust and inert structures, their electrical properties are extremely sensitive to the effects of charge transfer and chemical doping by various molecules 4. Nano inks - Ink formulations based on CNT dispersions are attractive for printed electronics applications such as transparent electrodes, RFID tags, thin-film transistors, light-emitting devices, and solar cells 5. Nanomedicine and biotechnology - CNT are widely researched for their potential uses in industrial applications, they also are of great interest to biomedical engineers working on nanotechnology applications. 6. Electrodes - Carbon nanotubes have been widely used as electrodes for chemical and biological sensing applications and many other electrochemical studies. 7. Displays - Given their high electrical conductivity, and the incredible sharpness of their tip, CNT are considered the most promising material for field emitters and a practical example are CNTs as electron emitters for field emission displays (FED). 8. Optoelectronic and photonic applications - While individual nanotubes generate discrete fine peaks in optical absorption and emission, macroscopic structures consisting of many CNTs gathered together also demonstrate interesting optical behavior. 9. Filtration - High-flow membranes are an important part of future energy-efficient water purification. Already, researchers have demonstrated efficient water transport in carbon nanotubes with openings of less than one nanometer. COLLEGE OF ENGINEERING & INFORMATION TECHNOLOGY ENGINEERING STUDENTS INSTRUCTIONAL MATERIALS DEVELOPMENT © 2024 LEARNING MODULE IN CHEMISTRY FOR ENGINEERS AGUSAN DEL SUR STATE COLLEGE OF AGRICULTURE AND TECHNOLOGY MAIN CAMPUS, BUNAWAN AGUSAN DEL SUR GRAPHENE ✓ Graphene, which is an isolated layer from graphite, is a two-dimensional form of carbon. ✓ It is a semimetal, which means its electronic structure is like that of a semiconductor in which the energy gap is exactly zero. ✓ The combination of graphene’s two-dimensional character and the fact that it is a semimetal allows the electrons to travel very long distances, up to 0.3 μm, without scattering from another electron, atom, or impurity. ✓ It can also sustain electrical current densities six orders of magnitude higher than those sustainable in copper. APPLICATIONS of GRAPHENE 1. Thermal applications (microelectronics) - Being heat conductive, strong and light, it means that it is a great material for making heat- spreading solutions, such as heat sinks or heat dissipation films. - This could be useful in both microelectronics (to make LED lighting efficient and longer lasting) and larger applications (to make thermal foils for mobile devices). 2. Energy storage (supercapacitors) - Since graphene is the world's thinnest material, it also extremely high surface-area-to- volume ratio. - This makes graphene a very promising material for use in batteries and supercapacitors. Graphene may enable batteries and supercapacitors (and even fuel cells) that can store more energy - and charge faster, too. 3. Coatings , sensors, electronics and more - Graphene has a lot of promise for additional applications: anti-corrosion coatings and paints, efficient and precise sensors, faster and efficient electronics, flexible displays, efficient solar panels, faster DNA sequencing, drug delivery, and more. CHALLENGES IN NANOTECHNOLOGY 1. The advantage brought by innovations in nanotechnology comes with a price. 2. Environmental effects of mineral-based nanoparticles found in cosmetics, paints & clothing as in question as they go to canals, and riverbeds undetected and may affect water sources. 3. Carbon nanoparticles when inhaled are more toxic than natural sources. 4. Due to its size, it is undetectable in air and may pose unknown health risks. In this new century, emerging technologies face a more skeptical and demanding public—not only must their benefits to society be clear, but scientists and engineers must also anticipate and characterize potential risks associated with their implementation. In the case of nanotechnology, these risks surround the potential environmental implications of the widespread use of engineered nanomaterials. Currently, nanomaterial exposures and health effects are unlikely to pose any substantial risk to public health given the most prevalent exposure routes and the limited scope of their use. However, as the quantity and types of engineered nanomaterials used in society increase, the potential for unintended environmental consequences will also increase. Though it is challenging to assess the risks of engineered nanomaterials before commercial products are well defined, proactive research is critical to ensuring a sustainable nanotechnology industry. For more reading on the topics of this module, you may browse the following references: 1. “Solid and Modern Materials” page 462 (General Chemistry 12th Edition by Theodore Brown, et. al.) 2. “Molecules and Materials” page 240 (Chemistry for Engineering Students 2nd Edition by Laurence Brown, et. al.) COLLEGE OF ENGINEERING & INFORMATION TECHNOLOGY ENGINEERING STUDENTS INSTRUCTIONAL MATERIALS DEVELOPMENT © 2024