Special Senses PDF
Document Details
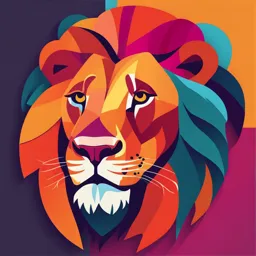
Uploaded by PositiveFermium
Tags
Summary
This document provides a general overview of special senses. It covers various topics like sensory receptors, their functions, classification, and adaptation..
Full Transcript
UNIT 5 SENSES PROFFESOR MCGEE 1 2 Sensory receptors Provide information about external and internal environments Respond to a stimulus General Each type of receptor re...
UNIT 5 SENSES PROFFESOR MCGEE 1 2 Sensory receptors Provide information about external and internal environments Respond to a stimulus General Each type of receptor responds best to a type of Function of stimulus Sensory For example, light energy for eye receptors; sound energy for ear receptors Receptors Transducers—convert stimulus energy into electrical Obj 5, 6 energy Receptors have a resting membrane potential Receptor membranes have modality gated channels that respond to their type of stimulus (temp, stretch, mechanical changes). Action potentials are conveyed to CNS for interpretation 3 3 Receptors convey signals to CNS by sensory neurons General Structure Receptive field—the distribution area of the endings of of Sensory a sensory neuron Smaller receptive fields allow more precise stimulus Receptors localization 4 4 Sensory receptors: specialized to respond to changes in environment (stimuli) Activation results in graded Sensory potentials that trigger nerve Receptors impulses obj 7 Awareness of stimulus (sensation) and interpretation of meaning of stimulus (perception) occur in brain 5 Sensory Sensation—a stimulus we are consciously aware of Information To enter consciousness, signals must reach cerebral cortex Provided by Only a fraction of stimuli result in sensations Sensory A lot of sensory input goes to other areas of the Receptors brain Obj 7 For example, blood pressure signals relayed to brainstem where responses are initiated without conscious awareness Receptors provide CNS information about stimulus modality, location, intensity, and duration 6 6 Modality—type of stimulus based on “labeled line” Sensory For example, brain interprets optic nerve Information signals to occipital lobe as visual, cochlear nerve signals as to temporal lobe as Provided by auditory Sensory Location of stimulus determined by which Receptors receptive field is active Obj 7, 8 Postcentral gyrus has body map represented by homunculus Intensity of stimulus determined by frequency of nerve signals to CNS Stronger stimuli cause more neurons to fire and cause sensitive neurons to fire more frequently 7 7 Receptor adaptation helps determine stimulus duration Adaptation—decreased sensitivity to continuous stimulus Receptor membranes become less responsive TONIC VS Receptor potentials decline in frequency or PHASIC - stop adaptation Phasic receptors: (fast-adapting) send signals at Obj 8 beginning or end of stimulus Examples: receptors for pressure, touch, and smell Tonic receptors: adapt slowly or not at all Examples: nociceptors, head position receptors in inner ear and most proprioceptors Adaptation: The greater the frequency, the stronger the stimulus. Adaptation is a change in sensitivity of the receptor, which means it doesn’t fire as frequently whenever a stimulus is constant. Not all receptors adapt. Fast-adapting receptors typically report changes, rather than constant stimuli, such as lamellar and tactile corpuscles. Slow adapting receptors don’t adapt readily and provide a sustained response, such as nociceptors and proprioceptors. Adaptation is why you can put clothes on and feel them, but then you immediately forget you have them on, or you can jump into a cool pool and after a few minutes it feels warm. Some receptors don’t adapt, like pain receptors, which is an evolutionary adaptation for our survival. 8 Categorized by distribution, stimulus origin, and stimulus modality Categorization by receptor distribution General sense receptors Simple structures distributed throughout the body Sensory Somatic sensory receptors: tactile receptors of skin and mucous membranes; proprioceptors of Receptor joints, muscles, and tendons Classification Visceral sensory receptors: found in walls of Obj 1 internal organs, they monitor stretch, chemical environment, temperature, pain Special sense receptors Specialized receptors in complex sense organs of the head 5 special senses: olfaction, gustation, vision, audition, equilibrium 9 9 10 Categorization by stimulus origin Exteroceptors detect stimuli from external environment Skin and mucus membranes; Sensory special sense receptors Receptor Interoceptors detect stimuli from Classification internal organs Obj 3 Visceral sensory receptors monitoring internal environment Proprioceptors detect body and limb movements Somatosensory receptors of muscles, tendons, and joints 11 11 Mechanoreceptors—respond to touch, pressure, vibration, and stretch (examples: Meissner’s corpuscles, Pacinian corpuscles, Baroreceptors) Thermoreceptors—sensitive to changes in temperature Receptors classified by Photoreceptors—respond to light energy (example: retina) stimulus/ modality Obj 2 Chemoreceptors—respond to chemicals (examples: smell, taste, changes in blood chemistry) Nociceptors—sensitive to pain-causing stimuli (examples: extreme heat or cold, excessive pressure, inflammatory chemicals) 12 13 Majority of sensory receptors belong to one of two categories: A. Simple receptors of the general senses Sensory Modified dendritic endings of sensory receptor neurons Classification Are found throughout body and monitor Obj 4 most types of general sensory information B. Receptors for special senses Vision, hearing, equilibrium, smell, and taste All are housed in complex sense organs 14 Tactile Receptors General senses include tactile sensations (touch, pressure, stretch, vibration), temperature, pain, and muscle sense No “one-receptor-one- function” relationship Receptors can respond to multiple stimuli Receptors have either: Nonencapsulated (free) nerve endings or Encapsulated nerve endings 15 Un-encapsulated Tactile Receptors Dendritic ends of sensory neurons with no protective cover Abundant in epithelia/connective tissues Respond mostly to temperature, pain, or light touch Thermoreceptors Cold receptors are activated by temps from 10 to 40ºC Located in superficial dermis Heat receptors are activated from 32 to 48ºC located in in deeper dermis Outside those temperature ranges, nociceptors are activated and interpreted as pain General senses are those that monitor most types of body senses. Their receptors are modified dendritic endings of sensory neurons. Certain ion channels in their plasma membrane respond to the appropriate stimulus by opening. They include tactile, temperature, pain, proprioception stimuli. Nonencapsulated nerve endings are not covered in anything. Free nerve endings can also monitor itch and there is overlap of these sensations. Merkel discs are located in the stratum basale of the epidermis 16 Un- Nociceptors: pain receptors triggered encapsulated by extreme temperature changes, free nerve pinch, or release of chemicals from damaged tissue endings: Acts as ion channel that is opened by Nociceptors & heat, low pH, chemicals (example: Itch Receptors capsaicin in red peppers) Itch receptors in dermis can be triggered by chemicals such as histamine 17 Obj. #4 18 Tactile Receptors - Encapsulated tactile receptors Neuron endings wrapped by connective tissue or covered by connective tissue and glial cells (neurolemmocytes) End (Krause) bulbs are ensheathed in connective tissue Located in dermis and mucus membranes Detect pressure and low-frequency vibration Tonic receptors Bulbous (Ruffini) corpuscles are wrapped in CT Within dermis and subcutaneous layer Detect deep pressure and skin distortion Tonic receptors 19 19 Encapsulated Tactile Receptors Tactile (Meissner’s) corpuscles small receptors involved in discriminative (light) touch Found just below skin, mostly in sensitive and hairless areas (fingertips) Lamellalated (Pacinian) corpuscles: Large receptors respond to deep pressure and vibration when first applied (then turn off) Located in deep dermis 20 21 21 Proprioceptors Proprioceptors are general sensory receptors located in muscles, tendons, and joints Specialized mechanoreceptors relay sensory info regarding body position and movement Proprioceptors are all tonic receptors (adapt slowly) Proprioception (the “sixth sense”) – sense of body position and movement Three types of proprioceptors: Muscle spindle – detect stretch in skeletal muscle Golgi tendon organ – detect stretch in tendon Joint kinesthetic receptor – detect stretch in articular capsule 22 22 23 Referred Pain obj 10 Referred pain—inaccurate localization of sensory signals Signals from viscera perceived as originating from skin, muscle Many somatic and visceral sensory neurons send signals via the same ascending tracts within spinal cord Somatosensory cortex unable to determine true source 24 24 General Organization of the Somatosensory System obj 5-8 Somatosensory system: part of sensory system serving body wall and limbs Receives inputs from: Exteroceptors, proprioceptors, and interoceptors Input is relayed toward head, but processed along the way Levels of neural integration in sensory systems: 1. Receptor level: sensory receptors 2. Circuit level: processing in ascending pathways 3. Perceptual level: processing in cortical sensory areas (brain) 25 Transduction: The stimulus energy must be converted into the energy of a graded potential (can be depolarizing or hyperpolarizing) When the receptor region is part of a sensory Processing at the neuron (like the free dendrites or encapsulated Receptor Level receptors of general sense receptors) , the graded excitability, potential is called a GENERATOR POTENTIAL Specificity, because it generates action potentials within the Frequency/Strength sensory neuron. When the receptor is a separate cell (like most special senses), the graded potential is called a RECEPTOR POTENTIAL because it occurs in a separate receptor cell – receptor potential changes the amount of neurotransmitter released by the receptor cell onto the sensory neuron. The stimulus must match the receptor, meaning the that light will not activate a touch receptor. The stimulus must be applied within the receptor’s receptive field, or the area in which it monitors. Transduction: the act of the energy of the stimulus being converted into a graded potential. May be EPSP or IPSP. This graded potential depends on if the receptor is part of a neuron, or a specialized cell, but most of the somatosensory receptors are parts of neurons. Separate cells are found in most special senses. Most graded potentials are the result of ion channels opening whether by mechanical or chemical changes. The graded potentials lead to threshold potentials and voltage- gated sodium channels opening, which instigates the action potential toward the CNS. 26 27 Olfaction: The Sense of Smell (obj 18-21) Olfaction—detection of odorants dissolved in the air Odorants (volatile molecules) dissolved in nasal mucus are detected by chemoreceptors Provides information about food, people, danger We can distinguish thousands of different odors Olfactory epithelium—sensory receptor organ Located in superior region of nasal cavity; has three types of cells Olfactory receptor cells detect odorants Supporting cells sustain receptors Basal cells continually replace olfactory receptor cells 28 28 Olfactory Epithelium 29 29 Olfaction: The Sense of Smell Olfactory receptor cells Primary neurons in sensory pathway for smell Bipolar structure: a single dendrite and unmyelinated axon Olfactory hairs: cilia projecting from receptor cell dendrite House chemoreceptors for a specific odorant (400 types of scent receptors. Perceived smell depends on which cells are stimulated Pain and temperature receptors are also in nasal cavities Respond to irritants, such as ammonia, or can “smell” hot or cold (chili peppers, menthol) Olfactory nerves (CN I) Bundles of olfactory cell axons Project through skull’s cribriform plate and enter olfactory bulbs 30 30 Olfaction: The Sense of Smell Olfactory nerve structures and pathways Olfactory bulbs (pair) Ends of olfactory tracts located under brain’s frontal lobes Olfactory nerve fibers synapse here with mitral cells and tufted cells Connections form olfactory glomeruli Olfactory tracts (pair) Axon bundles of mitral and tufted cells on inferior frontal lobe surface Project directly to primary olfactory cortex (in temporal lobe), hypothalamus, amygdala, and other regions Does not project through thalamus like other sensory pathways 31 31 Olfaction: The Sense of Smell Detecting smells Mucus contains odorant-binding proteins Olfactory sensations begin when odorant binds to protein and protein stimulates receptor cell (rapidly adapting receptor) G-protein in receptor cell activates adenylate cyclase, converts ATP to cAMP cAMP leads to opening of ion channels for Na+ Ca 2+ (depolarization) Action potential is triggered on axon, conducted to glomerulus Secondary neuron conducts signals to various CNS areas Adaption of smell occurs rapidly, as the initial changing of ion flow Cerebral cortex (perceive, identify smell), hypothalamus interferes with further generation of (visceral reaction to smell), amygdala (smell recognition, receptor potential. emotional reaction) 32 32 Gustation: The Sense of Taste (Obj 22-24) Gustation = sense of taste; detection of tastants Gustatory cells are chemoreceptors within taste buds Papillae of tongue Filiform papillae: short and spiked No taste buds (no role in gustation); help manipulate food Located on anterior two-thirds of tongue surface Fungiform papillae: mushroom-shaped Each contains a few taste buds Located on tip and sides of tongue 33 33 Gustation: The Sense of Taste Papillae of tongue Foliate papillae: leaflike ridges Not well developed House a few taste buds in early childhood Located on posterior lateral tongue Vallate (circumvallate) papillae: largest, least numerous Contain most of the taste buds Located in a row of 10 to 12 along posterior dorsal tongue surface 34 34 Tongue Papillae and Taste Buds 35 35 Gustation: The Sense of Taste Taste buds: onion-shaped organs housing taste receptors Gustatory cells: receptor cells detect tastants Supporting cells: sustain gustatory cells Basal cells: neural stem cells that replace gustatory cells 36 36 16.3b Gustation: The Sense of Taste Gustatory cells: neuroepithelial chemoreceptive cells of taste buds Gustatory microvillus (taste hair) forms dendritic ending Microvillus often extends through taste pore, to tongue surface Tastants (tasty molecules) dissolve in saliva and stimulate microvillus Gustatory pathways Sensory neurons connect to multiple gustatory cells in the tongue and project to the medulla In anterior parts of tongue, sensory neurons are part of the facial nerve (CN VII) In posterior two-thirds of tongue, sensory neurons are part of the glossopharyngeal nerve (CN IX) Secondary medullary neurons project to thalamus Tertiary thalamic neurons project to primary gustatory cortex in insula 37 37 Five basic taste sensations spread over broad regions of the tongue Sweet Produced by organic compounds, for example, sugar or artificial sweeteners Salt Gustation: The Produced by metal ions, for example, Na + and K + Sour Sense of Taste Associated with acids, for example, vinegar Bitter Produced by alkaloids, for example, unsweetened chocolate Umami Taste related to amino acids producing savory or meaty flavor 38 38 Transduction in gustatory cells For sweet, bitter, and umami the tastants are molecules Tastant binds to specific cell membrane receptor G protein is activated causing Gustation: The formation of 2nd messenger Sense of Taste Results in cell depolarization For salt and sour the tastants are ions The tastant depolarizes the cell directly Depolarized gustatory cell releases neurotransmitter stimulating primary neuron (in CN VII or CN IX) 39 39 Gustation: The Sense of Taste Gustatory pathway Primary neuron in cranial nerve brings signal to nucleus solitarius within medulla Medullary activity triggers salivation and stomach secretions Nauseating stimuli instead trigger gag or vomiting Signal is relayed to thalamus Then relayed to primary gustatory cortex for conscious taste Taste is integrated with temperature, texture, and especially smell Food has less taste if olfaction is blocked (For example, having a cold) 40 40 Gustatory Pathway 41 41 Influence of Other Sensations on Taste ❖Taste is 80% smell If nose is blocked, foods taste bland ❖Mouth also contains thermoreceptors, mechanoreceptors, and nociceptors Temperature and texture enhance or detract from taste Spicy hot foods can excite pain receptors in mouth, which some people experience as pleasure Example: hot chili peppers 42 Vision Obj 11-17 70% of body’s sensory receptors are in eye Half of cerebral cortex is involved in visual processing Small sphere; only one-sixth of surface visible Most of eye enclosed and protected by fat cushion and bony orbit Consists of accessory structures and the eyeball 43 Accessory Structures of the Eye Accessory structures are attached to or around the eye Include: six extrinsic eye muscles, eyebrows, eyelids, eyelashes, conjunctiva, lacrimal glands Eyebrows - Located along supraorbital ridge Aid in nonverbal communication (for example, surprise) and prevent sweat from dripping into eyes Eyelashes - Extend from margins of eyelids Prevent objects coming into contact with eye; can initiate blink reflex Eyelids (palpebrae) Eyelids join at medial and lateral palpebral commissures (canthi) Near the medial commissure is the lacrimal caruncle: small pink body Tarsal glands are sebaceous glands of tarsal plate Releases oily secretion at eyelid edge; Infection = chalazion (cyst in eyelid) Sebaceous and sweat gland at eyelid base - Infection = stye 44 44 Accessory Structures of the Eye Conjunctiva: transparent lining of eye and lid surfaces Specialized stratified columnar epithelium Ocular conjunctiva covers anterior sclera (white of eye) Palpebral conjunctiva covers internal surface of eyelid Contains numerous goblet cells to moisten eye, many blood vessels to nourish sclera, abundant nerve endings Does not cover cornea so as not to interfere with light passage Pink eye = conjunctivitis 45 45 External Anatomy of the Eye and Surrounding Accessory Structures: Sagittal Section 46 46 Accessory Structures of the Eye Lacrimal apparatus: produces, collects, drains fluid Lacrimal fluid: water, Na +, antibodies, lysozyme Lubricates, cleanses and moistens eye, reduces eyelid friction, defends against microbes, oxygenates and nourishes cornea Lacrimal gland: produces fluid and secretes it through ducts Blinking (15 to 20 per minute) washes fluid over eye Fluid drains into lacrimal puncta (holes by lacrimal caruncle) Each punctum has a lacrimal canaliculus draining to lacrimal sac Sac drains to nasolacrimal duct to nasal cavity Fluid then mixes with mucus and is swallowed Excess lacrimal fluid produces tears 47 47 Eye Structure Eye, almost spherical 2.5 cm diameter Located in skull’s orbit, padded by orbital fat Interior contains two cavities Posterior cavity (behind lens) contains permanent vitreous humor Anterior cavity (in front of lens) contains circulating aqueous humor Subdivided into anterior chamber and posterior chamber, separated by the iris Wall is formed by three tunics Fibrous (external) Vascular (middle) Retina (inner) 48 48 Internal Eye Structures 49 49 Eye Structure Vitreous humor (vitreous body) - Transparent gelatinous fluid in posterior cavity (behind lens) Permanent fluid first produced in embryonic development Helps eye maintain shape, supports retina—keeps it flush against back of eye Aqueous humor - Transparent watery fluid in anterior cavity (in front of lens) Continuously produced by ciliary processes Nourishes and oxygenates lens and inner cornea Aqueous humor production circulation and drainage Plasma filtered across capillary walls of ciliary processes in posterior chamber Aqueous humor circulates through pupil into anterior chamber It drains from the chamber via the scleral venous sinus and then to nearby veins Drainage failure can lead to glaucoma 50 50 Aqueous Humor: Secretion and Resorption Glaucoma - Characterized by increased intraocular pressure 51 51 Lens: changes shape to focus light on retina Cells within it have lost organelles and are filled with crystallin protein Lens enclosed by dense, fibrous elastic capsule Eye Structure Shape determines degree of light refraction Shape is determined by ciliary muscle and suspensory ligaments 52 52 Eye Structure Fibrous tunic: tough outer layer Composed of sclera and cornea Sclera: white of the eye Provides eye shape Protects internal components Attachment site for extrinsic eye muscles Cornea: anterior convex transparent “window” No blood vessels Limbus: corneal scleral junction Refracts light 53 53 Vascular tunic (uvea): middle layer with many vessels Houses blood vessels, lymph vessels, intrinsic muscles Three regions: choroid, ciliary body, iris Choroid: extensive, posterior region Many capillaries nourish retina Many melanocytes make melanin to absorb extraneous light Eye Structure Ciliary body: ciliary muscles and processes Ciliary muscles: bands of smooth muscle connected to lens Muscle contraction loosens suspensory ligaments, altering lens shape Ciliary processes: contain capillaries secreting aqueous humor 54 54 Vascular tunic (continued) Iris: gives eye color; most anterior region of uvea Contains smooth muscle, melanocytes, vessels, neural structures Divides the anterior segment into the anterior chamber (between cornea and iris) and posterior chamber (between iris and lens) Pupil is opening in center of iris connecting the two chambers Eye Structure Iris controls pupil diameter Sphincter pupillae muscles: concentrically circular fibers constrict pupil with parasympathetic nervous system activity (CN III) Dilator pupillae muscle: radially organized smooth muscle dilates pupil with sympathetic nervous system activity Pupillary reflex alters pupil size in response to light (increased brightness leads to constriction) 55 55 Iris Control of Pupil Diameter 56 Retina: internal or neural tunic Pigmented layer Attached to choroid (internal to it) Provides vitamin A for photoreceptors Absorbs stray light to prevent light scatter Neural layer Eye Structure Houses photoreceptors and associated neurons Receives light and converts it to nerve signals Ora serrata – jagged edge Boundary between photosensitive and nonphotosensitive parts of retina Nonphotosensitive part is anterior—covers ciliary body and posterior side of iris 57 57 Eye Structure Retina: cells of the neural layer form 3 sublayers Photoreceptor cell layer: outermost neural layer Contains rods and cones Contain pigments that react to light Bipolar cell layer Their dendrites receive synaptic input from rods and cones Ganglion cell layer: innermost neural layer Their axons gather at optic disc and form optic nerve Capable of action potentials Other retinal interneurons Horizontal cells regulate signals sent between photoreceptors and bipolar cells Amacrine cells regulate signals between bipolar and ganglion cells Capable of action potentials 58 58 Composition of the Layers of the Retina 59 59 Components of the retina Optic disc Contains no photoreceptors—blind spot Where ganglion axons exit toward brain Macula lutea Rounded, yellowish region lateral to optic disc Eye Structure Contains fovea centralis (central pit) Highest proportion of cones (hardly any rods) Area of sharpest vision Peripheral retina Contains primarily rods Functions most effectively in low light 60 60 Regions of the Retina, Including the Optic Disc (Blind Spot) 61 61 Detached Retina - Occurs when outer pigmented and inner neural layers separate Increased risk in diabetics and nearsighted individuals Clinical View: Results in nutrient deprivation in inner neural layer Detached Retina and Symptoms of “floaters” and “curtain” in affected eye, flashes of light, decreased vision Macular Macular Degeneration - Physical deterioration of macula lutea Degeneration Leading cause of blindness in developed countries May be associated with Diabetes, ocular infection, hypertension, eye trauma Loss of visual acuity in center of visual field Diminished color perception and “floaters” 62 62 Physiology of Vision: Refraction and Focusing of Light Refraction of light Sharp vision requires light rays to be bent (refracted) as they pass toward retina Refraction results when light passes Through curved surfaces such as the lens 63 63 Physiology of Vision: Refraction and Focusing of Light Focusing of light For objects 20 feet away and further: Eyes directed forward Ciliary muscles relaxed, tensing suspensory ligaments and flattening the lens (it’s “resting position”) Dilator pupillae of iris contracts, dilating pupil For objects closer than 20 feet (near response): Eyes directed medially, so image of object directed onto fovea centralis Ciliary muscles contract, decreasing tension on suspensory ligaments and lens becomes more spherical Light refracted to a greater extent; process called accommodation Sphincter pupillae contract, pupil constricts 64 64 Clinical View: Functional Visual Impairments Emmetropia: normal vision Parallel light rays focused on retina Hyperopia: far-sighted Trouble seeing up close; eyeball too short Corrected with convex lens Myopia: near-sighted Trouble seeing faraway objects; eyeball too long Corrected with concave lens Astigmatism - Unequal focusing Unequal curvatures in one or more refractive surfaces Presbyopia: age-related change in vision Lens less able to become spherical Reading close-up words becomes difficult Corrective convex lens 65 65 66 Physiology of Vision: Phototransduction Phototransduction: converting light to electrical signals Performed by photorece ptor cells (rods and cone s) Photorecep tor parts (from oute r to inner) Outer se gme nt extends into pigmen te d layer of retina Hu ndre ds of photopigme nt-containing discs that abs orb light Discs are contin ually re placed Inner segment contains cell organelles Ce ll body contains nucleus Synaptic terminals contain ves icles s toring glutamate ne urotrans mitter 67 67 Photoreceptors: rods and cones Rods are longer and narrower than cones; more numerous Each rod is highly sensitive: activated by even dim light The periphery of the retina contains many rods Physiology of Many rods converge on fewer bipolar cells, which converge on fewer ganglion Vision: cells Results in sensitivity to dim light but a Phototransduction blurry image Cones are concentrated at fovea centralis Activated by high intensity light, allow color vision Cones have one-to-one relationship with bipolar cells and ganglion cells Results in a sharp image but only possible in bright light 68 68 Photoreceptors 69 Physiology of Vision: Phototransduction Photopigments: light-absorbing molecules Found within membranes of outer segments of rods and cones Made of opsin protein and light-absorbing retinal (made from Vitamin A) Different pigment types have different opsins transducing different wavelengths (colors) of light Each photoreceptor has only one photopigment type Rods contain rhodopsin Three types of cones each containing a type of photopsin with a different sensitivity Blue cones detect short wavelengths, green cones absorb intermediate wavelengths, and red cones best detect long wavelengths 70 70 Absorption Wavelengths 71 71 Clinical View: Color Blindness X-linked recessive condition more common in males Absence or deficit in one type of cone cell Red and green most commonly affected Results in difficulty distinguishing red and green 72 72 Physiology of Vision: Phototransduction Phototransduction: light converted to electrical signal In the dark, rhodopsin contains cis-retinal Light causes reconfiguration to trans-retinal, which dissociates from opsin (bleaching reaction) After bleaching, rhodopsin must be rebuilt for rod to function Trans-retinal transported to pigmented layer and actively converted to cis-retinal Cis-retinal transported back into rod and combined with opsin Process is slow for rhodopsin—rods don’t function in bright light Process is similar for cone photopsins, but quicker More intense light needed for bleaching Photopsin regenerates rapidly 73 73 Dark adaptation Return of sensitivity to low light levels after bright light Bleached rods must regenerate rhodopsin May take 20 to 30 minutes to see well Physiology of Vision: Phototransduction Light adaptation Process of adjusting from low light to bright conditions Pupils constrict, but cones initially overstimulated Takes about 5 to 10 minutes for full adjustment 74 74 Phototransduction in Rod Photoreceptors 75 75 In retina Photoreceptors → bipolar cells → ganglion cells Ganglion cell axons bundle at disc to form optic nerve Optic nerves Exit backs of eyes and converge at optic chiasm Visual Medial axons cross to opposite side of Pathways brain Lateral axons remain on same side Optic tracts (ganglion cell axons from both eyes) Most axons go to lateral geniculate nucleus of thalamus Thalamic neurons’ axons project to visual cortex of occipital lobe 76 76 Visual Pathways Left and right eyes have overlapping visual fields Allows stereoscopic vision (depth perception) Some optic tract axons project to the midbrain Superior colliculi coordinate reflexive eye movements Pretectal nuclei coordinate pupillary reflex and lens accommodation reflex Ganglion cells projecting to pretectal nuclei are directly photoresponsive and contain melanopsin pigment 77 77 Ear Structure Obj 25-33 The ear detects sound and head movement Signals transmitted via vestibulocochlear nerve (CN VIII) External ear Auricle: funnel-shaped visible part of ear with elastic cartilage Protects ear entryway and directs sound waves inward External acoustic (auditory) meatus: ear canal Extends to tympanic membrane Ceruminous glands produce cerumen Ear wax impedes microorganism growth Tympanic membrane: eardrum Funnel-shaped epithelial sheet separating external and middle ear Vibrates when sound waves hit it 78 78 Ear Structure Middle ear Contains air-filled tympanic cavity Bony wall separates it from inner ear Wall has two membrane-covered openings: oval window and round window Auditory tube (Eustachian tube) Passage extending from middle ear to nasopharynx (upper throat) Middle ear infections often result from infections spreading from throat Usually closed, but yawning allows air movement through the tube Equalizes pressure on either side of tympanic membrane 79 79 Ear Structure Auditory ossicles: three tiny bones of middle ear Malleus - Attached to medial surface of tympanic membrane. Resembles a hammer in shape Incus - Middle ossicle resembling an anvil Stapes - Resembles a stirrup of a saddle. Has disclike footplate fitting into oval window Amplify sound waves and transmit them to oval window Vibrate along with eardrum, so stapes moves in and out of oval window initiating pressure waves in inner ear fluid Two small muscles restrict ossicle movement during loud sounds Tensor tympani attaches to malleus Stapedius attaches to stapes 80 80 Ear Structure Inner ear: spaces within petrous part of temporal bone Bony labyrinth: mazelike spaces in temporal bone Perilymph (interstitial fluid) fills most of this space Membranous labyrinth: membrane-lined fluid-filled tubes within bony labyrinth Contains receptors for hearing and equilibrium Contains endolymph, similar to intracellular fluid, rich in K + 81 81 Inner ear composed of three main regions: Cochlea Houses membranous cochlear duct Vestibule Contains two saclike, Ear Structure membranous parts: utricle and saccule, interconnected and positioned at right angles Semicircular canals Contains membranous semicircular ducts 82 82 Hearing Structures for hearing Cochlea: snail-shaped chamber of inner ear Modiolus: bony axis of the spiral Cochlear duct: membranous labyrinth in cochlea Roof formed by vestibular membrane Floor formed by basilar membrane Scala vestibuli: chamber of bony labyrinth adjacent to vestibular membrane Scala tympani: chamber of bony labyrinth adjacent to basilar membrane Helicotrema: small channel connecting scala vestibuli and scala tympani 83 83 Close-Up of Cochlea 84 84 Hearing Spiral organ: sensory structure for hearing Within cochlear duct Thick sensory epithelium consisting of hair cells and supporting cells on basilar membrane Hair cells: receptors that release neurotransmitter to sensory neurons Single row of inner hair cells; three rows of outer hair cells Hair cells have many stereocilia (long microvilli) and one kinocilium (long cilium) at their apex Stereocilia and kinocilium embedded in tectorial membrane Base of hair cells synapse with sensory neurons Cell bodies of sensory neurons are located in spiral ganglia of modiolus 85 85 Sound Wave Pathways Through the Ear 86 Hearing Cochlear hair cell stimulation Inner hair cells contain ion channels at their tips and tip link proteins that connect them Hair cells are bathed in K+ endolymph that is far more positive than the fluid inside the cell When basilar membrane moves up, hair cells are pushed into tectorial membrane and their tips are tilted, pulling tip links Tip links pull open ion channel allowing K+ to diffuse into the hair cell and depolarize it Hair cell releases more neurotransmitter from its base, exciting the sensory neuron, which can fire action potentials When basilar membrane moves down, the process quickly reverses 87 87 Hearing Perception of sound Sound is the perception of pressure waves established from vibrating objects Pitch depends on the frequency of the vibrating object Frequency is rate of vibration in Hertz (Hz; cycles per second) Humans can hear 20 to 20,000 Hz, most sensitive to 1500 to 4000 Hz Variations in pitch are detectable due to variations in stiffness of basilar membrane from oval window to cochlear apex High-frequency sounds excite cells in stiff basilar membrane near oval window Low-frequency sounds excite cells in flexible basilar membrane near apex Loudness depends on wave amplitude (degree of molecular compression) - measured in decibels (dB) Louder sounds create larger movements of basilar membrane Larger movements cause faster rate of nerve signals and a larger number of stimulated cells Temporal lobe’s auditory cortex interprets this as loudness 88 88 Sound Wave Detection at the Basilar Membrane 89 89 Central Nervous System Pathways for Hearing 90 90 Clinical View: Deafness Deafness is any hearing loss Two types Conductive deafness: interference of wave transmission in external or middle ear Sensorineural deafness: malfunction in inner ear or cochlear nerve 91 91 Equilibrium and Head Movement Equilibrium Awareness and monitoring of head position Monitored by vestibular apparatus: utricle, saccule, semicircular ducts Information sent to brain to help keep our balance Vision and proprioception also help Utricle and saccule detect static equilibrium and linear acceleration Semicircular ducts detect angular acceleration 92 92 Equilibrium and Head Movement Static equilibrium and linear acceleration Macula is receptor for static equilibrium and linear acceleration Located in utricle and saccule of vestibule Composed of a layer of hair cells and supporting cells Hair cells have stereocilia and one kinocilium projecting into gelatinous otolithic membrane Membrane is covered with otoliths— calcium carbonate crystals Head tilt shifts otolithic membrane and bends stereocilia Bending stereocilia toward kinocilium depolarizes hair cells and increases their transmitter release This increases impulse frequency on vestibular part of CN VIII 93 93 Macula in Upright Head Position 94 94 Macula in Altered Head Position 95 95 Equilibrium and Head Movement Angular acceleration Base of each semicircular canal has region called the ampulla Ampulla contains crista ampullaris with hair cells and support cells Stereocilia and kinocilia of hair cells are embedded in gelatinous cupula When head rotates endolymph pushes against cupula Cupula bends stereocilia and changes hair cell voltage If stereocilia bent toward kinocilium, the hair cell depolarizes If stereocilia bent away from kinocilium, the hair cell hyperpolarizes Neurotransmitter release from hair cells changes Firing rate changes on vestibular branch of CN VIII 96 96 Function of the Crista Ampullaris 97 97 Equilibrium and Head Movement Equilibrium pathways Signals from maculae or crista ampullaris are conveyed by the vestibular branch of CN VIII These axons terminate in vestibular nuclei or cerebellum Vestibular nuclei (in superior medulla) help control reflexive eye movements and balance Cerebellum helps coordinate balance and muscle tone Vestibular nuclei and cerebellum send signals to thalamus Thalamus relays information to cerebral cortex for awareness of body position 98 98