Soil Mechanics PDF
Document Details
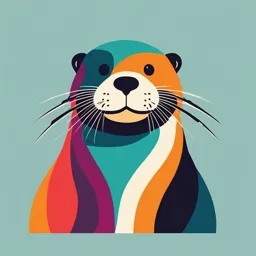
Uploaded by WorkablePink
Arrdekta Homoeopathic Technology
Tags
Summary
This document provides an overview of soil mechanics and foundation engineering, covering topics such as soil formation, properties, and index properties. The content appears to be a course outline rather than a full exam.
Full Transcript
SOIL MECHANICS AND FOUNDATION ENGINEERING 2.7.1 Pycnometer 34...
SOIL MECHANICS AND FOUNDATION ENGINEERING 2.7.1 Pycnometer 34 Method CH Topic Subtopic Page 2.8 Determination of In- 1 Soil Formation 1 35 Situ Unit Weight 1.1 Definition of Soil 1 2.9 Index Properties of 39 Soils 1.2 Definition of Soil 1 Mechanics 2.10 Particle Size Analysis 40 1.3 Definition of Soil 2.10.1 Sieve Analysis 40 1 Engineering 2.10.2 Sedimentation 1.4 Definition of Rock 43 1 Analysis Mechanics 2.11 Consistency of Clays 1.5 Geotechnical 49 1 (Atterberg's Limits) Engineering 2.12 Sensitivity 63 1.6 Origin of Soil 2 2.13 Thixotropy 63 1.6.1 Weathering Stage 2 2.14 Unconfined 1.6.2 Transportation and 63 3 Compressive Strength Deposition 2.15 Activity 64 1.7 Soil Deposits in India 4 2.16 Collapsibility 64 1.8 Organic and Inorganic 5 Soils 2.17 Relationship between Atterberg Limits 1.9 Common Types of 65 5 and Engineering Soils Properties Objective Brain Teasers 7 Objective Brain Teasers 71 2 Properties of Soils 9 Student's Assignments 73 2.1 Introduction 9 Identification and 3 74 Classification of Soils 2.2 Phase Diagram 9 3.1 Introduction 74 2.3 Basic Definitions 10 3.2 Field Identification of 2.4 Some Important 74 14 Soils Relationships 3.3 Engineering 2.5 Relative Density 27 75 Classification of Soils 2.6 Methods for 3.3.1 Textural Determination of Water 31 Classification (more Content 75 suitable for coarse- grained soils) 2.7 Determination of Specific Gravity of Soil 34 Solids 1 3.3.2 The Unified Soil 5.1 Introduction 95 Classification System 76 (USCS) 5.2 Principles of 95 Compaction 3.3.3 'AASHTO' Soil 76 Classification System 5.3 Difference between Compaction and 96 3.3.4 Indian Standard Soil Consolidation Classification System 76 (ISSCS) 5.3.1 Compaction 96 3.4 Classification of 5.3.2 Consolidation 96 77 Coarse Grained Soil 5.4 Advantages of 96 3.5 Classification of Fine Compaction 81 Grained Soil 5.5 Laboratory 96 Objective Brain Teasers 85 Compaction Soil Structure and 5.5.1 Standard Proctor 4 88 97 Clay Minerals Test 4.1 Introduction 88 5.5.2 Modified Proctor 98 Test 4.2 Clay Minerals 88 5.5.3 Indian Standard 4.3 Structure of Clay Equivalent of Standard 88 98 Minerals Proctor Test (Light Compaction Test) 4.4 Isomorphous 89 Substitution 5.5.4 Indian Standard Equivalent of Modified 98 4.5 Types of Clay Proctor Test (Heavy 89 Minerals Compaction Test) 4.6 Clay Water 5.6 Comparison of 90 Relationship Standard and Modified 98 Proctor Test 4.7 Clay Particle 91 Interaction 5.7 Zero Air Void Line 100 4.8 Soil Structure 91 5.8 Constant Percentage 100 Air Void Lines 4.9 Types of Soil 92 Structures 5.9 Factors Affecting 104 Compaction 4.9.1 Single Grained 92 Structure 5.10 Compaction 105 Behaviour of Sand 4.9.2 Honeycomb 92 Structures 5.11 Effect of Compaction 105 on Properties of Soils 4.9.3 Flocculated and 92 Dispersed Structures 5.11.1 Soil Structure 105 4.9.4 Structure of a 5.11.2 Permeability 106 93 Composite Soil 5.11.3 Compressibility 106 Objective Brain Teasers 93 5.11.4 Swelling 5 Soil Compaction 95 CH Topic Subtopic Page 2 5 Soil Compaction 5.11.5 Shrinkage 107 6.7.4 Limitations of 140 Darcy's Law 5.11.6 Shear Strength 107 6.7.5 Coefficient of 140 5.12 Field Compaction Permeability 107 and Equipment 6.8 Permeability of 143 5.13 Evaluation of Stratified Soils 107 Compaction 6.8.1 Horizontal Flow 143 5.14 Compaction Quality 108 Control 6.8.2 Vertical Flow 143 5.15 Settlement During 6.9 Determination of 109 Compaction Coefficient of 146 Permeability Objective Brain Teasers 114 6.9.1 Laboratory Methods 146 Student's Assignments 118 6.9.2 Field Methods 153 Principle of Effective 6 Stress, Capillarity and 120 6.9.3 Indirect Methods 160 Permeability 6.10 Factors Affecting 162 6.1 Introduction 120 Permeability 6.2 Total Stress, Pore 6.11 Coefficient of 163 Pressure and Effective 120 Absolute Permeability Stress Objective Brain Teasers 165 6.2.1 Total Stress 120 Student's Assignments 169 6.2.2 Pore Pressure 121 CH Topic Subtopic Page 6.2.3 Principle of 121 Effective Stress Seepage Through 7 7.1 Introduction 171 Soils 6.3 Effective Stress in 121 Partially Saturated Soils 7.2 Type of Head 171 6.4 Capillarity in Soils 122 7.3 Total Head 172 6.4.1 Capillary Rise in 7.3.1 Head Loss 172 122 Soils 7.4 Seepage Pressure and 6.4.2 Capillary Pressure 123 its Effect on Effective 174 Stress 6.5 Geostatic Stresses in 124 Soils 7.4.1 No Flow Condition 175 6.6 Effect of Water Table 7.4.2 Downward Flow 176 Fluctuations on Effective 136 Stress 7.4.3 Upward Flow 177 6.7 Permeability of Soils 138 7.5 Quick Sand Condition 177 6.7.1 Darcy's Law 138 7.6 Laplace Equations 182 6.7.2 Discharge Velocity 139 7.7 Flow Nets 183 6.7.3 Seepage Velocity 139 7.7.1 Properties of Flow 183 Nets 3 7.7.2 Application of Flow 9.3.4 Compression Test: 183 223 Nets Oedometer Test 7.8 Flow Through Non- 9.3.5 Computation of 187 223 Homogeneous Section Void Ratio 7.9 Piping Failure and its 9.4 Graph between Void 188 226 Protection Ratio and Effective Stress 7.9.1 Methods to Prevent 9.5 Determination of 188 Piping Failure Compressibility 227 Parameters 7.10 Seepage Through 188 Earthen Dams 9.6 Settlement Analysis 230 7.10.1 Procedure to Draw 9.7 Time Rate of 241 Phreatic Line and 189 Consolidation Computation of Seepage 9.8 Terzaghi's Theory of Objective Brain Teasers 196 One Dimensional 241 Consolidation Student's Assignments 198 Objective Brain Teasers 251 Stress Distribution in 8 8.1 Introduction 199 Soils Student's Assignments 254 8.2 Boussinesq's Theory 199 Shear Strength of 10 10.1 Introduction 266 Soils 8.3 Vertical Stress 201 Distribution Diagrams 10.2 Concept of Stress 266 8.4 Westergaard's Theory 201 10.3 Shear Strength of 266 Soil 8.5 Comparison between Boussinesq and 205 10.3.1 Mechanism of 267 Westergaard Theories Shear Resistance 8.6 Approximate 10.3.2 Stress at a Point - 272 Methods for Vertical 214 Mohr Circle of Stress Stress Computation 10.4 Factors Affecting 276 Objective Brain Teasers 219 Shear Strength Student's Assignments 220 10.5 Representation of Results of Direct Shear 278 Compressibility and Test 9 9.1 Compressibility 221 Consolidation of Soil 10.6 Triaxial Test 278 9.2 Consolidation 221 Objective Brain Teasers 300 9.3 Normally and Over 222 Consolidated Soils Student's Assignments 304 9.3.1 Normally Earth Pressure and 222 11 11.1 Introduction 306 Consolidated Soils Retaining Walls 9.3.2 Over Consolidated 11.2 Retaining Structures 306 222 Soils CH Topic Subtopic Page 9.3.3 Over Consolidation 223 Ratios (OCR) CH Topic Subtopic Page 4 Earth Pressure and 11.3 Earth Pressure at 12.2.1 Infinite Slopes 370 11 308 Retaining Walls Rest 12.2.2 Finite Slopes 370 11.4 Calculation of Lateral Thrust due to 309 12.2.3 Stability of Infinite 371 Retaining Backfill Slopes 11.5 Active and Passive 12.3 Definitions of Factor 312 377 Earth Pressure of Safety 11.5.1 Active Earth 12.4 Stability of Finite 312 378 Pressure Slopes 11.5.2 Passive Earth 12.4.1 Types of Failure 379 312 Pressure 12.4.2 Total Stress 11.5.3 Earth Pressure Analysis for a Purely 379 314 Theories Cohesive Soil 11.6 Plastic Equilibrium 12.4.3 Swedish Method 314 380 State of Slices (for c-ϕ soils) 11.6.1 Rankine's Active 12.4.4 Friction Circle 314 380 State Method 11.6.2 Rankine's Passive 12.5 Effective Stress 315 381 State Analysis 11.7 Various Cases of 12.5.1 Steady Seepage 382 Earth Pressure in 316 Cohesionless Soil 12.5.2 Case of Rapid 382 Drawdown 11.8 Active and Passive Earth Pressure in 336 12.5.3 Case of Cohesive Soils Immediately after 382 Construction 11.8.1 Active State 338 12.6 Effective Stress 11.8.2 Total active Analysis by Bishop's 383 pressure per unit length 340 Method of wall 12.6.1 Taylor's Stability 383 11.9 Coulomb's Wedge Number Method 349 Theory Objective Brain Teasers 386 11.9.1 Active State 350 Student's Assignments 388 11.10 Sheet Pile Walls 352 13 Soil Exploration 13.1 Introduction 389 11.10.1 Cantilever Sheet 352 Pile 13.2 Purpose of Soil 389 Exploration 11.10.2 Approximate 354 Analysis 13.2.1 Stage in Sub- 389 surface Exploration Objective Brain Teasers 365 13.2.2 Depth of 389 Student's Assignments 368 Exploration 12 Stability of Slopes 12.1 Introduction 370 13.2.3 Methods of 390 Exploration 12.2 Types of Slopes 370 5 13.3 Types of Soil 15.8.4 Bell's Method 409 394 Samples 15.8.5 Fellenius's Method 409 13.4 Samplers 394 15.8.6 Terzaghi's Method 409 13.4.1 Disturbance of Soil 394 Samples 15.9 Factors Affecting 412 Ultimate Bearing Capacity 13.4.2 Design of Sampler 395 15.10 Another Approach 13.4.3 Field Tests 396 of Accounting the Effect 420 of Water Table Objective Brain Teasers 396 15.11 Safe Settlement 14 Soil Improvement 14.1 Introduction 399 Pressure based on SPT 435 Value 14.2 Improvement 399 Techniques Objective Brain Teasers 445 14.2.1 Soil Stabilization 399 Student's Assignments 449 14.2.2 Grouting and 16 Deep Foundation 16.1 Introduction 451 400 Injection 16.2 Floating Foundation 453 14.2.3 Soil Reinforcement 401 16.3 Classification based 14.2.4 Application of on Mechanism of Load 454 Geo-textiles and Geo- 401 Transfer membranes 16.3.1 Classification 454 14.2.5 Drainage Methods 401 based on Function 14.2.6 Surface 16.3.2 Classification 401 Compaction based on Soil 455 Displacement Objective Brain Teasers 402 16.3.3 Classification 455 Bearing Capacity and based on Material 15 15.1 Introduction 403 Shallow Foundation 16.3.4 Classification 15.2 Modes of Failure of based on Method of 456 403 a Structure Installation 15.5 Important 16.4 Methods of 404 Definitions Determining Bearing 456 Capacity of Pile 15.6 Mode of Shear 404 Failure 16.4.1 Static Method 457 15.7 Methods to 16.5 Determination of Determine Bearing 406 Load Carrying Capacity of 472 Capacity Pile Group 15.8 Analytical Methods 407 16.6 Pile Groups in Clay 472 15.8.1 Schleicher's 16.7 Pile Group in Sands 473 407 Method 16.8 Allowable or Safe 473 15.8.2 Rankine's Theory 407 Load on Pile Group 15.8.3 Pauker's Method 408 16.9 Efficiency of Pile 474 Group 6 16.10 Converse Labarre 474 Formula to Find n 16.11 Design of Pile 477 Group 16.12 Load Tests on Pile 480 16.13 Cyclic Load Test 481 16.14 Correlations with 482 Penetration Test Data 16.15 Settlement of Pile 483 Group 16.15.1 Determination of 483 Group Settlement Ratio 16.15.2 Settlement of Pile 483 Groups in Clay Objective Brain Teasers 491 Student's Assignments 494 7 CH-1_____Soil Formation________ 1.1 Definition of Soil: o Generally, "soil" refers to the upper layer of the earth's surface that supports plant life. o For engineering purposes, soil is defined as an uncemented aggregate of mineral grains and decayed organic matter (solid particles) with liquid and gas in the spaces between the solid particles. o Soil is an unconsolidated material resulting from the disintegration of rocks by weathering (water, air, etc.). It can contain inorganic or organic matter and is represented as a three-phase system: solids, water, and air. Soil is used in construction and supports structural foundations. 1.2 Definition of Soil Mechanics: o Soil mechanics is the branch of science studying the physical properties of soil and the behavior of soil masses under various forces. o The term "Soil Mechanics" was coined by Dr. Karl Terzaghi in 1925, known as the Father of Soil Mechanics. o Terzaghi defined it as "the branch of civil engineering that concerns the application of the principles of mechanics, hydraulics, and chemistry to engineering problems related to the soil." 1.3 Definition of Soil Engineering: o Soil engineering applies the principles of soil mechanics to practical problems. o It's a broader term encompassing soil mechanics, geology, structural engineering, and soil dynamics to find practical solutions to soil-related problems. o It includes site investigation, lab testing, design, construction, and maintenance of foundations and earth-retaining structures. 1.4 Definition of Rock Mechanics: o A "rock" is a natural aggregate of mineral particles bound by strong, permanent cohesive forces. o Rock mechanics applies the principles of mechanics to understand the behavior of rock masses. 1.5 Geotechnical Engineering: o Geotechnical engineering is a sub-discipline of civil engineering dealing with natural materials found near the earth's surface. o It applies the principles of soil mechanics and rock mechanics. 8 1.6 Origin of Soil Almost all soils are formed by the disintegration of rocks through physical or chemical weathering. Residual Soil: If weathered sediments remain over the parent rock. Transported Soil: If weathered sediments are transported and deposited elsewhere. Pedogenesis: The process of soil formation. Geological Cycle (Rock Cycle): The cyclic nature of soil formation. Fig. 1.1 Geological Cycle (suggested by Bowles, 1984): This diagram illustrates the rock cycle: Igneous Rock: Formed from the melting of deeply buried rock. Can become metamorphic rock through heat, pressure, and solution, or sediment through weathering. Metamorphic Rock: Formed from igneous or sedimentary rock by heat, pressure, and solution. Can become igneous rock through melting or sediment through weathering. Sedimentary Rock: Formed from sediment (gravel, sand, mud, etc.) through compaction and cementation. Can become metamorphic rock through heat, pressure, and solution, or sediment through erosion and weathering. Sediment: The product of weathering of all rock types, which can then form sedimentary rock. Stages in the Geological Cycle of Soil Formation (in Transported Soil): (1) Weathering: Breakdown of rocks. (2) Transportation: Movement of weathered material. (3) Deposition of weathered materials: Settling of transported material. (4) Upheaval: Uplift of deposited material. 9 Fig. 1.2 Stages of Geological Cycle in the case of transported soil: This diagram visually represents the stages: weathering on a mountain, transportation of the weathered material down the slope, deposition at a lower elevation, and eventual upheaval of the deposited material. 1.6.1 Weathering Stage Rock disintegration, also called weathering, is a key geological process. Weathering can be: o Physical (Mechanical): Breakdown of rocks into smaller pieces without changing their chemical composition (e.g., freeze-thaw cycles, abrasion). o Chemical: Breakdown of rocks through chemical reactions that alter their composition (e.g., oxidation, hydrolysis). Physical Weathering: Rock disintegrates into smaller fragments due to: o Running water o Heavy wind o Temperature changes o Rainfall o Expansion due to freezing water o Human activities Generally, sand and gravel fall into this category. The mineral constituents remain the same as the parent rock. Chemical Weathering: Fragmented rock materials from physical weathering sometimes undergo changes in mineral composition, forming new compounds. Caused mainly by: o Oxidation o Hydration o Carbonation o Leaching water o Organic acids Generally, clays and, to some extent, silts fall into this category. 1.6.2 Transportation and Deposition: Weathered rock material is transported by: o Running water (rivers) o Moving ice (glaciers) o Blowing wind 10 Materials transported and deposited elsewhere are called "Transported soil." Classification of Transported Soil based on Transporting Agency: 1. Water transported soil: These sedimentary deposits are of three types: o (i) Alluvial deposit: ▪ Running water carries soil in suspension or by rolling/sliding along the stream bed. ▪ When water velocity decreases, these sediments are deposited. ▪ This type of soil is called "Alluvial soil" (alternate layers of sand, silt, and clay). ▪ Examples: Alluvial cones, natural levees, and deltas. o (ii) Lacustrine deposit: (but these are deposits formed in lake environments). o (iii) Marine deposit: but these are deposits formed in ocean environments. 2.0 Flash Experimental. Might not work as expected. (ii) Lacustrine Deposit: Soil carried by a river entering a lake deposits coarse particles due to a sudden decrease in velocity. Fine-grained particles move to the center of the lake and settle when the water becomes still. Alternate layers are formed with the seasons. Such lake deposits are called "Lacustrine deposits." (iii) Marine Soil: These are soils formed by the sedimentation of soil particles in deep sea water. Marine deposits have very low shearing strength and are highly compressible. They contain a large amount of organic matter. 11 2. Wind Transported Soil (Aeolian Deposits): Wind can erode, transport, and deposit fine-grained soils. Soils transported and deposited by wind are called "Aeolian deposits." Dunes are formed by the accumulation of wind-deposited sand. Loess: Loess is a silt deposit made by wind. Characteristics: Low density, slight cementation, high compressibility, and poor bearing capacity when wet. Cementation is due to calcium carbonate from decayed vegetable matter. When partially saturated, loess undergoes volume reduction with increased moisture content, even without changes in applied stress. This phenomenon is called "collapse compression," due to the collapse of the soil structure. Soils susceptible to collapse are called "collapsible soils" or "metastable soils." Glacial Deposits: Glaciers are large masses of ice formed by compacted snow. Glaciers move slowly, deforming and scouring the surface and bedrock. Melting glaciers deposit all the carried material; this deposit is called "till." "Drift" is a general term for deposits made by glaciers directly or indirectly. Glacial deposit particles are generally angular, unlike the rounded particles of waterborne deposit. Fig. 1.4 Glacier Deposited Soil: This diagram shows various glacial features: o Terminal moraine: Debris accumulated at the end of a glacier. o Outwash: Sediment carried by meltwater away from the glacier. o Ground moraine: Debris deposited beneath the glacier. Gravity Deposits (Colluvial Soil/Talus): Gravity transports materials over short distances. Gravity deposits are called "colluvial soil" or "talus." Found in mountain valleys, formed by gravity. 12 On sloping mountains, moisture variations cause sediments to creep downwards. Swelling and shrinkage can cause landslides, resulting in valley deposits. These soils consist of irregular particles of varying size. Swamp and Marsh Deposits: Form in waterlogged areas with fluctuating water tables and vegetation. Characteristics: Soft, high in organic content, unpleasant odor. Accumulation of partially or fully decomposed aquatic plants is called "muck" or "peat." Muck soil is lightweight and highly compressible, making it unsuitable for construction. 1.7 Soil Deposits in India: India is divided into five major soil groups: o Alluvial deposits o Black cotton soil o Laterite soil o Desert soil o Marine deposits 1.8 Organic and Inorganic Soils: Soils can be classified as organic or inorganic. Organic Soils: o Formed by the growth and subsequent decomposition of plants (e.g., peat and mosses). o Generally, organic soil is transported soil from rock weathering containing decomposed vegetable matter. Inorganic Soils: o Referred to as ordinary soil obtained from rock disintegration due to weathering. 1.9 Common Types of Soils: Loess: o Wind-blown, uniformly graded fine soil. o Formed in arid and semi-arid regions. o Yellowish-brown in color. o Deposits found in Rajasthan and North Gujarat (India). Caliche: Cemented soil rich in calcium carbonate, consisting of gravel, sand, and clays. Formed by wind action in semi-arid climates and cemented by calcium carbonate left from capillary water evaporation. Loam: A mixture of sand, silt, and clay in definite proportions, sometimes containing organic matter. 13 Cumulose (Peaty/Organic Soils/Muck): Formed by organic content accumulation under waterlogged conditions. Found in areas with poor sewerage or after river flooding. Gumbo: Highly sticky, plastic, and dark-colored soil. Marl: Fine-grained calcium carbonated soil of marine origin, formed by the decomposition of cell mass and bones of aquatic life. Humus: A mixture of mud and dead plants. Tiny rock pieces and humus combine to create various soils. Peat: Highly organic soil containing almost decomposed vegetable matter. Gravel: Coarse-grained soil with particle size 4.75 mm to 80 mm. Sand: Cohesionless aggregates of rounded, sub-angular, or angular sediment, size 0.075 mm to 4.75 mm. Silt: Fine-grained soil, particle size 0.002 mm to 0.075 mm. Clay: Aggregate of microscopic and submicroscopic mineral particles. Can be organic or inorganic. Cobbles: Large particles, 80 mm to 300 mm. Boulders: Large rock fragments, >300 mm. Tuff: Small-grained, slightly cemented volcanic ash transported by wind or water. Bentonite: Clay formed by chemical weathering of volcanic ash, high in montmorillonite. Pulverized bentonite slurry is highly plastic and used as a drilling lubricant. Kaolin (China Clay): Very pure white clay, used extensively in the ceramic industry. Hardpans: Soils resisting drilling tool penetration during soil exploration. Dense, well-graded, cohesive aggregates of mineral particles. Varved Clays: Sedimentary deposits of alternate thin layers of silt and clay, formed in lakes during alternating high and low water periods. Till: Formed by glaciers and icebergs, a mixture of gravel, sand, silt, and clay. Well-graded soils. Summary: Soil is unconsolidated material of sediments derived from rocks. Soil formation is cyclic (Geological Cycle). Soil formation process is Pedogenesis. Weathered sediments remaining over parent rock are "residual soil." Weathered sediments transported and deposited elsewhere are "transported soil." Major Indian soil deposits: alluvial deposits, black cotton soil, laterite/lateritic soil, desert soil, and marine deposits. Boulders: Boulders are rock fragments of large size (more than 300 mm). Tuff: These are small-grained, slightly cemented volcanic ash that has been transported by wind or water. 14 Bentonite: It is a clay formed by chemical weathering of volcanic ash which has a high content of montmorillonite. Pulverized slurry of bentonite is highly plastic and is often used as a lubricant in drilling. Kaolin (China Clay): It is a very pure form of white clay, which is extensively used in the ceramic industry. Hardpans: Hardpans are types of soils that offer great resistance to the penetration of drilling tools during soil exploration. These 1 are generally dense, well-graded, 2 cohesive aggregates of mineral particles. Varved Clays: These are sedimentary deposits consisting of alternate thin layers of silt and clay. These clays are the result of deposition in lakes during periods of alternate high and low waters. Till: It is formed by glaciers and icebergs and may contain a mixture of gravel, sand, silt, and clay. These soils are well-graded. Summary: Soil is an unconsolidated material which consists of sediments derived from rocks. The soil formation process is cyclic, which is called the 'Geological Cycle'. The process of soil formation is called 'Pedogenesis'. If weathered sediments remain over the parent rock, then the soil is called 'residual soil'. Weathered sediments transferred and deposited at other places are called transported soil. Major soil deposits of India are alluvial deposits, black cotton soil, laterite and lateritic soil, desert soil, and marine deposits. 15 2______Properties of Soils_____________ 2.1 Introduction: Soil consists of solid particles with spaces or voids in between. The connected particles are called the "soil matrix" or "soil skeleton." Void spaces are filled with air, water, or both. Soil is a three-phase material: solid (soil grains), liquid (pore water), and gas (pore air). Fig. 2.1 Three-phase solid-water-air system: This shows a visual representation of soil with solid particles, water filling some voids, and air filling other voids. 2.2 Phase Diagram: Soil mass is a three-phase system (solid, liquid, and gaseous). These phases cannot be easily separated in reality, so a diagram is used for better understanding. Fig. 2.2 Three-Phase diagram: This diagram separates the three phases into distinct blocks: o Air: Volume of air (Va), Weight of air (Wa = 0 - weight of air is usually considered negligible in soil mechanics calculations) o Water: Volume of water (Vw), Weight of water (Ww) o Soil Solids: Volume of solids (Vs), Weight of solids (Ws) o Total Volume (V) = Va + Vw + Vs o Volume of voids (Vv) = Va + Vw o Total Weight (W) = Ws + Ww (since Wa is negligible) The diagrammatic representation of these phases is called the "phase diagram" or "block diagram." A three-phase diagram applies to partially saturated soil (0 < S < 1, where S is the degree of saturation). 16 When all voids are filled with water (S = 1), the soil is saturated, and the gaseous phase (air) is absent, making it a two-phase system. 17 This image describes two-phase systems (saturated and dry soil) and introduces basic soil definitions: water content and degree of saturation. Two-Phase Diagrams (Fig. 2.3): Fully Saturated Soil (S=1): All voids are filled with water. The air phase is absent. The diagram shows only water and soil solids. Volume of voids (Vv) equals the volume of water (Vw). Oven-Dry Soil (S=0): All water is removed. The liquid phase is absent. The diagram shows only air and soil solids. Volume of voids (Vv) equals the volume of air (Va). 2.3 Basic Definitions: (i) Water Content (w): Also called moisture content. Ratio of the weight of water (Ww) to the weight of soil solids (Ws). Formula: w = (Ww / Ws) w ≥ 0 (Water content cannot be negative). Expressed as a percentage. Oven-dry soil has a water content of zero. Natural water content for most soils is around 50%. No upper limit for water content; it can be greater than 100%. Fine-grained soils have higher natural moisture content than coarse-grained soils. NOTE: 1. Fine-grained soils have higher values of natural moisture content compared to coarse-grained soils. 2. Four forms of water in soil: o (i) Gravity Water (Free Water): Added by rain or flooding. o (ii) Capillary Water: Held by capillary action. o (iii) Hygroscopic Water: Absorbed by an oven-dried sample in open air. o (iv) Structural Water: Bound within the crystalline structure of the soil. o Oven drying removes gravity, capillary, and hygroscopic water, but structural water remains. 3. Water content (w) represents gravity, capillary, and hygroscopic water, which can be removed by oven drying. 18 (ii) Degree of Saturation (S) - Continued Formula: S = (Vw / Vv) * 100% o Where: ▪ Vw = Volume of water ▪ Vv = Volume of voids Expressed as a percentage. For dry soil, S = 0%. For fully saturated soil, S = 100%. Partially saturated soil has 0% < S < 100%. NOTE: If soil is partially saturated, the total volume of soil and volume of voids remain constant during variation of moisture content. If soil is supersaturated due to further addition of water, then the volume of voids and total volume increases. Hence, the void ratio will change, but the degree of saturation remains constant equal to 100%. (iii) Void Ratio (e): Ratio of the total volume of voids (Vv) to the volume of solids (Vs). Formula: e = Vv / Vs o Where: ▪ Vv = Volume of voids ▪ Vs = Volume of soil solids e > 0 (Void ratio cannot be negative). Expressed as a decimal. No upper limit for void ratio. Void ratio of fine-grained soils is generally higher than that of coarse-grained soils. Individual void spaces in coarse-grained soils are larger than in fine-grained soils, but the total void space is generally more in fine-grained soils. (iv) Porosity (n): Ratio of the volume of voids (Vv) to the total volume of soil (V). Formula: n = (Vv / V) * 100% o Where: ▪ Vv = Volume of voids ▪ V = Total volume of soil Expressed as a percentage. Porosity uses the total volume of soil (including voids). Porosity (n) cannot exceed 100%. 19 The range of porosity is 0% < n < 100%. NOTE: Void ratio (e) and porosity (n) have the same significance, but void ratio (e) is more widely adopted because the volume of solids (used in void ratio) is more stable than the total volume (used in porosity). (v) Air Content (ac): Ratio of the volume of air in voids (Va) to the total volume of voids (Vv). Formula: ac = Va / Vv o Where: ▪ Va = Volume of air in voids ▪ Vv = Volume of voids Expressed as a percentage. (vi) Percentage Air Voids (na): Ratio of the volume of air (Va) to the total volume of the soil mass (V). Formula: na = (Va / V) * 100% o Where: ▪ Va = Volume of air ▪ V = Total volume of soil Expressed as a percentage. (vii) Unit Weights: (a) Bulk Unit Weight (γ): Ratio of the total weight of soil (W) to the total volume of the soil mass (V). Formula: γ = W / V = (Ws + Ww) / (Vs + Vw + Va) Expressed as kN/m³ or kgf/cm³ (Note: kgf/cm³ is less common now; kN/m³ or Mg/m³ is preferred). NOTE: Bulk density (ρt) is the ratio of the total soil mass (M) to the total volume (V). Formula: ρt = M / V = (Ms + Mw) / (Vs + Vw + Va). Expressed as kg/m³. The key difference is that unit weight uses weight (force due to gravity), while density uses mass. They are related by: γ = ρ * g, where g is the acceleration due to gravity. (b) Dry Unit Weight (γd): Ratio of the total dry weight of soil (Wd) to the total volume of the soil mass (V). Formula: γd = Wd / V Dry unit weight is a measure of soil denseness. Higher dry unit weight means denser or more compacted soil. NOTE: Dry density (ρd) is the ratio of the total dry mass (Md) to the total volume (V). Formula: ρd = Md / V. (c) Saturated Unit Weight (γsat): Ratio of the total saturated weight of soil (Wsat) to the total volume of the soil mass (V). Formula: γsat = Wsat / V 20 Saturated Density: Saturated density (ρsat) is defined as the ratio of the total saturated soil mass (Msat) to the total volume (V) of the soil mass. ρsat = Msat/V (d) Submerged Unit Weight or Buoyant Unit Weight (γ'sub or γ'): Ratio of the buoyant weight of soil (W'sub) to the total volume of the soil mass (V). Formula: γ' = W'sub / V When soil is below water (submerged condition), a buoyant force acts on the soil solids, equal to the weight of water displaced. This reduces the net weight of the soil; this reduced weight is the buoyant or submerged weight. Alternative Formula: γ' = γsat - γw (Saturated unit weight minus unit weight of water) γ' is roughly ½ of the saturated unit weight (γsat). Submerged Density or Buoyant Density: ρ' = ρsat - ρw (e) Unit Weight of Water (γw): Ratio of the weight of water (Ww) to the volume occupied by the water (Vw). Formula: γw = Ww / Vw Unit weight of water depends on temperature. Commonly taken as a constant: 9.81 kN/m³ or 1 g/cm³ (1 Mg/m³). (f) Unit Weight of Solids (γs): Ratio of the weight of soil solids (Ws) to the volume occupied by the soil solids (Vs). Formula: γs = Ws / Vs Expressed in kN/m³ or kgf/cm³ (or Mg/m³). (viii) Specific Gravity (G): Specific gravity of soil solids (G) is the ratio of the weight of a given volume of solids (Ws) to the weight of an equal volume of water at 4°C (Vsγw). Formula: G = Ws / (Vsγw) = γs / γw The specific gravity of most inorganic soils is 2.65 to 2.80. For organic soils, it ranges from 1.2 to 1.40. The bracketed portion in the image [γs = Ws/Vs] is simply restating the formula for the unit weight of solids. It's used in the derivation of the specific gravity formula. 21 Here's a breakdown of the two methods for determining water content from the image: 1. Oven Drying Method: Principle: This method involves completely drying the soil sample to remove all moisture. The weight loss due to drying represents the water content. Procedure: 1. Weigh an empty container (W1). 2. Weigh the container with a moist soil sample (W2). 3. Dry the soil sample in an oven at a suitable temperature (105-110°C for inorganic soils, 60°C for organic soils, 80°C if gypsum is present). 4. Weigh the container with the dry soil sample (W3). Calculations: o Weight of water (Ww) = W2 - W3 o Water content (w) = (Ww / Ws) * 100 where Ws is the weight of dry soil (Ws = W3 - W1) 2. Pycnometer Method: Principle: This method uses the principle of displacement of water by the soil particles to determine the volume of solids. Knowing the weight and volume of solids, and the total weight of the moist soil, the water content can be calculated. Procedure: 1. Weigh an empty pycnometer bottle (W1). 2. Weigh the pycnometer bottle filled with a known volume of water (W4). 3. Weigh the pycnometer bottle filled with water and a known weight of moist soil (W3). 4. Weigh the pycnometer bottle filled with water and the dry soil sample (W2). Calculations: o Weight of water displaced by the dry soil (Ww) = W4 - W2 o Volume of dry soil (Vs) = Ww / density of water o Weight of dry soil (Ws) = W2 - W1 o Water content (w) = (W3 - W2) / Ws * 100 Key Points: Accuracy: The oven drying method is generally considered more accurate than the pycnometer method. Time: The pycnometer method is typically faster than the oven drying method. Considerations: o The pycnometer method requires knowing the specific gravity of the soil solids. 22 o The oven drying method requires careful temperature control to avoid loss of organic matter or structural water. If you have a specific soil sample and want to determine its water content, you can choose the method that is most suitable for your needs based on accuracy, time constraints, and the availability of equipment. 3. Calcium Carbide Method/Rapid Moisture Meter Method: This is a rapid method, taking only 5 to 7 minutes, but it may not be highly accurate. A small soil sample (4-6 grams) is placed in a closed chamber within a moisture testing device. This device has a calibrated scale that measures pressure, which is directly related to the water content. Calcium carbide powder (CaC₂) is added to the soil. It reacts with the water in the soil, producing acetylene gas (C₂H₂), which increases the pressure within the chamber. The chemical reaction is: CaC₂ + 2H₂O → Ca(OH)₂ + C₂H₂↑ The moisture content is initially recorded as a percentage of the moist weight of the soil. However, the actual water content is expressed as a fraction of the dry weight of the soil. The image provides a formula to convert the recorded moisture content (wᵣ) to the actual water content (w): w = (wᵣ / (1 + wᵣ)) * 100% 4. Sand Bath Method: This is a quick field method used when an electric oven is not available. The soil sample is placed in a container and dried by heating it on a sand bath over a kerosene stove. The water content is determined similarly to the oven drying method. The image provides a formula to calculate the water content: w = ((W₁ - W₂) / (W₂ - W₃)) * 100, where it appears W1 could stand for the initial weight, W2 the weight after drying, and W3 the weight of the container. However, the image is unclear about what each weight represents. A more standard formula for moisture content is: Moisture Content (%) = [(Wet Weight - Dry Weight) / Dry Weight] * 100 5. Alcohol Method: This is another quick field method. Methylated spirit (denatured alcohol) is mixed with the soil sample to increase the rate of evaporation, and then the mixture is ignited. The formula provided is: w = ((W₁ - W₂) / W₂) * 100, where W₁ is the initial weight of the sample and W₂ is the weight of the soil after cooling of the soil and methylated spirit mixture. This formula calculates the moisture content as a percentage of the dry weight. This method is very rapid but less accurate. Important Note: Because alcohol is an oxidizing agent, this method should not be used for organic soils or soils containing organic compounds, as the alcohol could react with the organic matter and give inaccurate results. 6. Torsion Balance Method: This is a laboratory method. Infrared radiation is used to dry the soil sample. 23 Drying and weighing are done simultaneously. This method is rapid, accurate, and most suitable for soils that quickly reabsorb moisture after drying (hygroscopic soils). 7. Radiation Method: This is an in-situ method (meaning it's performed in place, without removing a sample) to determine the water content of soil. Radioactive isotopes are used. A radiating device containing radioactive isotopes like Cobalt 60 is placed inside a capsule and lowered into a steel casing (A). The casing has a small opening through which rays can exit. A detector is placed inside another steel casing (B), also with an opening facing casing A. The principle is that the hydrogen atoms in the water molecules of the soil affect the radiation passing between the source and the detector. By measuring the change in radiation intensity, the water content can be determined. In summary, these three methods offer different approaches to measuring soil moisture: a rapid but less accurate field method using alcohol, a precise laboratory method using infrared radiation, and an in-situ method using radioactive isotopes. Each method has its own advantages and limitations depending on the specific application and soil type 2.7 Pycnometer Method for determining the specific gravity of soil solids (Gs). Here's a breakdown: 2.7.1 Pycnometer Method: This method is similar to the pycnometer method for water content determination, but uses oven-dried soil instead of moist soil. Procedure and Formulas: 1. Weights: o W₁ = weight of the empty pycnometer o W₂ = weight of the pycnometer + oven-dry soil sample o W₃ = weight of the pycnometer + soil solids + water (filled to the mark) 24 o W₄ = weight of the pycnometer filled completely with water 2. Calculations: o Weight of solid (soil): Ws = W₂ - W₁ o Weight of equivalent volume of water: (W₄ - W₁) - (W₃ - W₂) 3. Specific Gravity (Gs): o Gs = (Weight of solid) / (Weight of equivalent volume of water) o Gs = (W₂ - W₁) / [(W₄ - W₁) - (W₃ - W₂)] Important Notes: Standard Reporting Temperature: Specific gravity values are generally reported at 27°C (in India). Temperature Correction: If the test temperature is T°C, the specific gravity at 27°C (G27°C) can be calculated using the following formula: o G27°C = GT°C * (Unit weight of water at T°C) / (Unit weight of water at 27°C) Using Kerosene: If kerosene (a better wetting agent for some soils) is used instead of water, the formula becomes: o Gs = [(W₂ - W₁) / [(W₄ - W₁) - (W₃ - W₂)]] * K o Where K = specific gravity of kerosene. Indirect Determination: Gs can also be determined indirectly using the shrinkage limit of the soil. In simpler terms: The pycnometer method compares the weight of a dry soil sample to the weight of an equal volume of water. The ratio of these weights gives the specific gravity of the soil solids. The method uses a special flask called a pycnometer to accurately measure volumes. The notes address adjustments for temperature and the use of kerosene as an alternative liquid. 2.8 Determination of In-Situ Unit Weight 1. Core Cutter Method: Applicability: This method is used for cohesive soils. It is not suitable for hard or gravelly soils, as the cutter cannot easily penetrate these materials. Core Cutter: A core cutter is a cylindrical vessel with open top and bottom and sharp cutting edges. A typical core cutter has a diameter of 10 cm and a height of 12.5 cm, resulting in a volume of approximately 1000 cc (cubic centimeters). Procedure: 1. The soil surface is prepared and leveled in the field. 2. The cylindrical core cutter is driven (punched) into the ground. 3. The cutter, now filled with soil, is carefully removed. 4. The soil at the top and bottom of the cutter is trimmed to be level with the cutter's edges. 25 Calculations: o Let: ▪ W₁ = weight of the empty core cutter ▪ W₂ = weight of the core cutter + soil ▪ W = weight of the soil = W₂ - W₁ ▪ V = Volume of the soil sample = Volume of the core cutter = π/4 * D² * H (where D is the diameter and H is the height of the cutter) o In-situ bulk unit weight (γt): γt = W / V o Dry unit weight (γd): If the water content (w) is determined in the laboratory, the dry unit weight can be calculated as: γd = γt / (1 + w) In simpler terms: The core cutter method involves driving a cylindrical container into the ground to obtain an undisturbed soil sample. By weighing the soil within the known volume of the cutter, the in-situ bulk unit weight (total weight per unit volume) is determined. If the water content of the soil is also known, the dry unit weight (weight of solid particles per unit volume) can be calculated. This method is a direct and relatively simple way to determine the density of soil in its natural state. 2. Sand Replacement Method: Applicability: This method is used for hard and gravelly soils where the core cutter method is not suitable (because the cutter cannot be driven into such dense material). Procedure: 1. A small pit or hole is dug in the ground. 2. The excavated soil is carefully collected and weighed (W). 3. The pit is then filled with a known type of dry sand (of known density) using a calibrated container (often a pouring cylinder with a conical attachment to ensure consistent pouring). 4. The volume of sand required to completely fill the pit (V) is measured. This volume is assumed to be equal to the volume of the excavated soil. Calculations: o In-situ bulk unit weight (γ): γ = W / V (where W is the weight of the excavated soil and V is the volume of the pit, determined by the volume of sand used). o Dry unit weight (γd): If the water content (w) of the excavated soil is determined (usually in the lab), the dry unit weight is calculated as: γd = γ / (1 + w) Application: This method is widely used in highway construction. In simpler terms: Since a core cutter can't penetrate hard or gravelly soils, the sand replacement method uses a different approach. A hole is dug, and the excavated soil is weighed. Then, the hole is filled with a known volume of sand. Because the sand fills the exact shape of the hole, the sand's volume represents the volume of the excavated soil. Knowing the weight of the soil and its volume allows for calculation of the bulk unit weight. 26 As with the core cutter method, the dry unit weight can be determined if the moisture content of the soil is known. 3. Water Displacement Method: Applicability: This method is suitable for highly cohesive and sticky soils where it's possible to obtain a lump (intact) sample. Procedure: 1. A small, intact soil sample is taken. 2. Let W₁ = weight of the soil sample. 3. The soil sample is coated with wax to prevent water from entering the soil pores during immersion. 4. The waxed soil sample is immersed in a water-filled cylinder. The volume of water displaced is measured (Vw). This displaced volume represents the total volume of the waxed soil sample (soil + wax). 5. Let W₂ = weight of (soil sample + wax). 6. Let γwax = unit weight of wax. The volume of wax (Vwax) can be calculated as: Vwax = (W₂ - W₁) / γwax Calculations: 1. Volume of the soil sample (V): V = Vw - Vwax = Vw - ((W₂ - W₁) / γwax) 2. Bulk unit weight of soil (γ): γ = W₁ / V = W₁ / (Vw - ((W₂ - W₁) / γwax)) In simpler terms: This method is used for cohesive soils that can be handled as a lump. The soil is coated with wax to make it waterproof. Then, the waxed soil is submerged in water, and the volume of displaced water is measured. This volume includes both the soil and the wax. Since we know the weight and unit weight of the wax, we can calculate the wax's volume. By subtracting the wax's volume from the total displaced volume, we get the volume of the soil alone. Finally, dividing the weight of the soil by its volume gives us the bulk unit weight. 27 2.9 Index Properties of Soils Soils with similar behavior can be grouped into categories. Tests are performed to assess the engineering behavior of soils. Definition: Index properties are used for the identification and classification of soils. Two General Types of Index Properties: o (i) Soil grain properties o (ii) Soil aggregate properties Distinction: Soil grain properties depend on the individual soil grains, while soil aggregate properties depend on the soil mass as a whole (including its history, mode of formation, and structure). Soil aggregate properties are of greater engineering importance. (I) Soil Grain Properties: These properties relate to the individual grains of the soil. The most important soil grain properties are: o (a) Grain size distribution: determined by sieve analysis (for coarser particles) and sedimentation analysis (for finer particles). o (b) Grain shape: described as bulky, flaky, needle-shaped, etc. (II) Soil Aggregate Properties: These properties relate to the behavior of the soil mass as a whole. The various soil aggregate properties are: o (a) Unconfined compressive strength (qu) o (b) Consistency and Atterberg limits (Liquid Limit, Plastic Limit, Shrinkage Limit) o (c) Sensitivity (loss of strength upon remolding) o (d) Thixotropy and soil activity (regain of strength over time after remolding) o (e) Relative density (for cohesionless soils) Type of Soil Index Property Coarse Soil Particle size, Grain shape, and Relative density Fine Soil Atterberg limits and consistency, unconfined compressive strength, thixotropy, and activity 28 2.10 Particle Size Analysis Grain Size Distribution: This analysis determines the proportions of different particle sizes within a soil sample. Methods: o Sieve analysis: Used for coarse-grained soils (gravel and sand). o Sedimentation methods (hydrometer or pipette): Used for fine-grained soils (silt and clay). Combined Analysis: Most soils contain a mix of coarse and fine particles, requiring a combined analysis. This involves: 1. Separation: Dry soil is sieved through a 4.75 mm sieve. 2. Coarse Sieve Analysis: The fraction retained on the 4.75 mm sieve (gravel) is analyzed using coarser sieves. 3. Fine Sieve Analysis: The fraction passing through the 4.75 mm sieve is further analyzed using finer sieves. 4. Sedimentation Analysis: The fraction passing through the 0.075 mm sieve is analyzed using a hydrometer or pipette method. S.N. Type of Soil Particle Size Remark 1 Boulder > 300 mm Not considered soil 2 Cobbles 80 mm - 300 mm 3 Gravel 4.75 mm - 80 mm 4 Sand 0.075 mm - 4.75 mm Coarse grained soil (4.1) Coarse sand 2 mm - 4.75 mm (4.2) Medium sand 0.425 mm - 2 mm (4.3) Fine sand 0.075 mm - 0.425 mm 5 Silt 0.002 mm - 0.075 mm (5.1) Coarse silt 0.02mm-0.075 mm (5.2) Medium silt 0.01 mm-0.02 mm Fine grained soil (5.3) Fine silt 0.002 mm - 0.01 mm 6 Clay < 0.002 mm 29 Particle Size Analysis Flowchart: The image also includes a flowchart summarizing the process: Particle size analysis o Sieve analysis (for coarse soils) ▪ Coarse sieving (>4.75 mm) ▪ Fine sieving (