Small Hydroelectric Power Plants PDF
Document Details
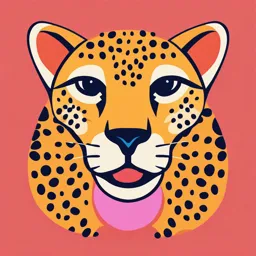
Uploaded by LuxuriousLagoon
Università degli Studi di Padova
D. Del Col
Tags
Summary
This document provides an overview of small hydroelectric plants, classifications of types, and the relationship between power and flow. It details different aspects of the technology, such as different head and flow rate classifications. It includes diagrams and calculations relating to these aspects.
Full Transcript
UNIVERSITẦ DEGLI STUDI DI PADOVA LM Energy engineering Renewable Energy Technologies Hydraulic energy D. Del Col Source: European Small Hydropower Association – ESHA, Guide on How to Develop a Small Hydropower...
UNIVERSITẦ DEGLI STUDI DI PADOVA LM Energy engineering Renewable Energy Technologies Hydraulic energy D. Del Col Source: European Small Hydropower Association – ESHA, Guide on How to Develop a Small Hydropower Plant, 2004 Hydropower electricity in the European Union, both large and small scale, represents 13% of the total electricity generated, reducing the CO2 emissions by more than 67-million tons a year. Whereas conventional hydro requires flooding of large areas of land, with consequential environmental and social issues, properly designed small hydro schemes are easily integrated into local ecosystems. In 2001, approximately 365 TWh of hydro energy was produced in the European Union from an overall capacity of 118 GW. Small hydro plants accounted for 8,4% of installed capacity (9,9 GW) and produced 39 TWh (about 11%). Given a more favorable regulatory environment, the European Commission set the objective of 14 000 MW by 2010. The large majority of small hydro plants are “run-of-river ” schemes, meaning that they have no or relatively small water storage capability. The turbine only produces power when the water is available and provided by the river. When the river flow falls below some predetermined value, generation ceases. WHAT DO WE MEAN BY SMALL HYDROELECTRIC SYSTEM? From the point of view of the definition of the concept of "small hydroelectric plant" there is still some confusion. The limit of distinction between the "large" and the "small hydroelectric", in fact, has not yet been established unambiguously. A definition for these plants has not been agreed at the European Union level and this circumstance could represent a handicap to their diffusion. Some EU member states such as Portugal, Spain, Ireland, Greece and Belgium set the border line at installed powers of 10 MW: in Italy the limit is set at 3 MW in France at 8 MW in Great Britain at 5 MW “SMALL” HYDROELECTRIC Speaking of hydroelectric energy, we intend to refer to the electricity that can be obtained from the exploitation of a water fall, converting the energy possessed by the water current with a special machinery. Hydroelectric plants therefore exploit the potential and kinetic energy of a body of water, available at a higher altitude than the return level of the derived water. The conversion of hydraulic energy into electricity takes place through a turbine- generator system. The power P produced by this system is expressed by the relationship: P = η γ Q H [W] being: Q volumetric flow rate [m3/s] H head [m] γ specific weight of liquid = ρg [N/m3] η global system efficiency [-] “SMALL” HYDROELECTRIC The electricity E produced by a plant is consequently determined by the relationship : E = P x n [kWh] being: P net power at given flowrate [kW] n number of working hours [h] “SMALL” HYDROELECTRIC On the basis of the nominal power Pn installed in the plant, hydroelectric plants can be conventionally classified into: · I. Micro-plants: Pn < 100 kW; II. Mini-plants: 100 < Pn < 1000 kW; III. Small-plants: 1000 < Pn < 10000 kW ; IV. Large-plants: Pn > 10000 kW. With reference to the hydraulic scheme, the systems can then be classified by distinguishing between: A. run-of-the-river systems B. plants with storage and regulation basin “SMALL” HYDROELECTRIC Depending on the available head, hydroelectric plants can then be classified as plants: · 1. low head (H < 50 m); · 2. medium head (H = 50 ÷ 250 m); · 3. high head (H = 250 ÷ 1000 m); · 4. very high head (H > 1000 m). While, depending on the flow rate used, we speak of : · I. low flowrate (Q < 10 m³/s); · II. medium flowrate (Q = 10 ÷ 100 m³/s); · III. large flowrate (Q = 100 ÷ 1000 m³/s); · IV. very large flowrate (Q > 1000 m³/s). Hydroelectric plants are classified, on the basis of the duration of the water storage, into three categories: reservoir, basin, run-of-the-river. The storage duration of a reservoir is the time necessary to supply the storage itself with a volume of water equal to its useful capacity using the average annual flow rate of the watercourses that flow into it (excluding any pumping inputs). On the basis of their storage duration, the reservoirs are classified into: - seasonal reservoirs: those with a storage duration greater than or equal to 400 hours; - weekly or daily modulation basins: those with a reservoir duration of less than 400 hours and greater than 2 hours. The three categories of plants are therefore defined as follows: Reservoir systems: those that have a reservoir classified as a seasonal storage; Basin systems: those that have a storage classified as a modulation basin; Run-of-the-river systems: those that have no storage or have a storage with a duration equal to or less than two hours. IN ITALY 2009 152 reservoir systems (of which 22 with pure or mixed pumping) 177 basin plants 1927 run-of-the-river systems Average annual yield from natural contributions 47003 GWh/year Average annual yield from pumping inlets (depending on where the pumps draw): - voluntary 6654.8 GWh/year - other 183.6 GWh/year POSSIBLE HYDRAULIC SCHEMES FOR THE EXPLOITATION OF HYDRAULIC ENERGY AND THE ELECTRICITY PRODUCTION Medium and high head schemes use weirs to divert water to the intake, from where it is then conveyed to the turbines via a pressure pipe or penstock. Penstocks are expensive and consequently this design is usually uneconomic. An alternative (Fig. 1) is to convey the water by a low slope canal, running alongside the river to the pressure intake or forebay and then in a short penstock to the turbines. If the topography and morphology of the terrain does not permit the easy layout of a canal a low pressure pipe can be an economical option. At the outlet of the turbines, the water is discharged to the river via a tailrace. Low head schemes are typically built in river valleys. Two technological options can be selected. Either the water is diverted to a power intake with a short penstock (Fig. 2), as in the high head schemes, or the head is created by a small dam, provided with sector gates and an integrated intake (Fig. 3), powerhouse and fish ladder. A small hydropower scheme cannot afford a large reservoir to operate the plant when it is most convenient; the cost of a relatively large dam and its hydraulic appurtenances would be too high to make it economically viable. But if the reservoir has already been built for other purposes, such as flood control, irrigation, water abstraction for a big city, recreation area, etc, – it may be possible to generate electricity using the discharge compatible with its fundamental use or the ecological flow of the reservoir. The main issue is how to link headwater and tail water by a waterway and how to fit the turbine in this waterway. If the dam already has a bottom outlet, see Fig. 4, for a possible solution. Provided the dam is not too high, a siphon intake can be installed. Integral siphon intakes (Fig. 5) provide an elegant solution in schemes, generally, with heads up to 10 m and for units up to about 1000 kW, although there are examples of siphon intakes with an installed power up to 11 MW (Sweden) and heads up to 30,5 m (USA). The turbine can be located either on top of the dam or on the downstream side. The unit can be delivered pre-packaged from the works, and installed without major modifications to the dam. DERIVATION WITH RESERVOIR reservoir dam DERIVATION WITH RUN-OF-RIVER immediate return remote return diversion Minimum Vital Flow: operators of hydroelectric power plants are required to make available part of water of their derivations in order to protect river ecosystems In 1989, with the enactment of the Italian Law n.183, the hydrological regime relevance was recognized by the Italian Government. In particular, the law established the idea of a “minimum constant flow” to be guaranteed in riverbeds, to promote a balance between human needs and the natural requirements of the riverine ecosystem (Law 183/1989). The Legislative Decree n.152/2006 introduced the concept of “Minimum Vital Flow” (hereafter MVF), as the instant outflow needed to be preserved in downstream water diversions in order to conserve the physical (morphological, hydrological and hydraulic), physical chemical (water quality) and biological features of natural riverine ecosystems. To date, the general formulas adopted among the Italian Districts by the River Basin Authorities, are based on simple hydrological formulas, mostly considering the percentage of mean annual or monthly flow, corrected through several coefficients taking into account different environmental aspects. For the Po River Basin Authority Minimum Vital Flow NATIONAL LAWS Legge 5 gennaio 1994 nr. 36 (cosiddetta legge Galli) D. Lgs. 152/99 corretto e integrato dal D. Lgs. 258/2000 D.M. 28 luglio 2004 REGIONAL LAWS DGR n.VII/2604 del 11/12/2000 Flow duration curves for a small hydro plant considering two different values of the nominal flowrate Flow rate Flow rate unusable area unusable area Days Days Head Flowrate Operating ranges of the different types of most used turbines. The figure was developed by integrating the values communicated by various european manufacturers. The limits are not strict, varying from manufacturer to manufacturer according to the technology used and therefore the diagram is indicative. efficiency % % nominal flowrate Typical values of efficiency EXERCISE Calculate the annual Energy Production from a mini-hydraulic system (see figure) Run-of-river with derivation Net head: 12 m Turbine: Semi-Kaplan Nominal flow rate: 17 m3 s-1 Cut-off flow rate: 5 m3 s-1 Minimum vital flow: 6 m3 s-1 remote return diversion EXERCISE, cont curve of annual duration of the flow of the watercourse RUN-OF-THE-RIVER- SYSTEM WITH DERIVATION AND REMOTE RETURN Volumetric flow rate, Days TYPICAL PERFORMANCES OF HYDRAULIC TURBINES efficiency % % nominal flowrate curve of annual duration of the flow of the watercourse RUN-OF-THE-RIVER- SYSTEM WITH DERIVATION AND REMOTE RETURN Volumetric flow rate, Minimum vital flow Days 1 2 3 4 5 6 7 8 9 10 11 12 13 14 15 16 17 18 19 NUMBER OF INTERVAL Each interval is made of 20 days N° Qtot Qtur Pid Pid η Preale E intervallo m3 s-1 m3 s-1 kW Pid nom kW MWh 1 26,00 17,00 2001 1 0,880 1761 845,3 2 23,26 16,80 1978 0,988 0,880 1741 835,5 3 21,86 15,86 1867 0,933 0,880 1643 788,6 4 20,82 14,82 1745 0,872 0,880 1536 737,1 5 20,04 14,04 1653 0,826 0,880 1455 698,2 6 19,35 13.35 1572 0,786 0,885 1391 667,8 7 18,67 12,67 1492 0,746 0,885 1320 633,8 8 18,19 12,19 1435 0,717 0,885 1270 609,6 9 17,80 11,80 1389 0,694 0,885 1229 590,0 10 17,44 11,44 1347 0,673 0,880 1185 569,0 11 17,15 11,15 1313 0,656 0,880 1155 554,6 12 16,86 10,86 1278 0,639 0,880 1125 539,8 13 16,48 10,48 1234 0,617 0,875 1080 518,3 14 15,88 9,88 1163 0,581 0,870 1012 485,7 15 15,03 9,03 1063 0,531 0,865 919 441,4 16 13,92 7,92 932 0,466 0,835 778 373,5 17 12,26 6,26 737 0,368 0,780 575 275,9 18 9,80 - - - - - - 19 8,7 - - - - - - Producibilità energetica Annual energyannua: yield : 10164 MWh/anno Specific production 10164 Producibilità oraria annua 5772 ore/anno (ratio of production to the nominal power) 1, 761