Regulatory RNAs: CRISPR & miRNA PDF
Document Details
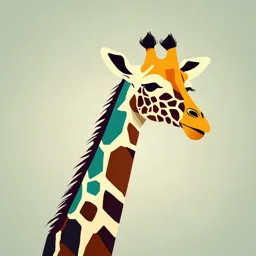
Uploaded by QuickerWormhole6372
Hebrew University of Jerusalem
Tags
Summary
This document discusses regulatory RNAs, including CRISPR-Cas9 and miRNA, and their roles in gene regulation and editing. It details the mechanisms of these systems, including gene modification and gene silencing, highlighting their importance in biology and medicine, with applications like making a specific cut in the genome.
Full Transcript
27/01 REGULATORY RNAs: CRISPR & miRNA CRISPR-CAs9 CRISPR-Cas9 is a system of bacterial origin that functions as an adaptive immune system, conferring immune memory and resistance against infections by phages (viruses against bacteria) CRISPR (Clusters of Regulatory Interspaced Palindromic Repeats)...
27/01 REGULATORY RNAs: CRISPR & miRNA CRISPR-CAs9 CRISPR-Cas9 is a system of bacterial origin that functions as an adaptive immune system, conferring immune memory and resistance against infections by phages (viruses against bacteria) CRISPR (Clusters of Regulatory Interspaced Palindromic Repeats) is a DNA sequence containing palindromic repeats separated by segments of foreign DNA (such as that of viruses). Cas9, a protein associated with CRISPR, is an RNA-guided endonuclease, capable of recognizing and cutting target DNA corresponding to a guide sequence. The Cas9 protein is like a pair of molecular scissors. Guided by the RNA, it precisely attaches to the corresponding viral DNA and cuts it, thus deactivating the virus. Natural origin: This system is a defense mechanism used by bacteria to protect themselves against viruses. When a virus attacks, the bacteria keeps a small portion of the viral DNA (as a "memory"), inserted in the form of a spacer. CRISPR is the overall structure that contains the repeats and spacers. Recognition: If the same virus attacks again, the bacteria uses an RNA copy of this memory (called a guide RNA) to recognize and target the virus's DNA. By modifying the spacers, it is possible to alter the bacteria's ability to protect itself against specific phages. Use in the laboratory: Scientists have hijacked this mechanism. By modifying the guide RNA, any piece of DNA in a cell can be targeted and cut. This makes it possible to "repair", "modify" or "deactivate" specific genes in the genome of various organisms. Process 1. A phage injects its DNA into a bacterium. 2. The bacterium identifies the phage DNA and extracts a fragment, which it inserts into its CRISPR sequence as a new spacer (located between repeated sequences). 3. The CRISPR sequence containing the spacers is transcribed into a long precursor RNA (an immature form of RNA that requires additional processing steps to become functional) 4. This precursor RNA is cut into small fragments, called guide RNAs (gRNAs). Each gRNA corresponds to a specific spacer and retains the information to target the phage DNA 5. The gRNAs bind to the Cas9 protein, forming an active complex capable of targeting specific DNA sequences. 6. If the phage reinfects the bacterium, the gRNA guides Cas9 to the viral DNA corresponding to its spacer 7. The Cas9 protein cuts the phage DNA, preventing its replication and protecting the bacterium CRISPR-Cas9 for gene editing CRISPR is a revolutionary tool in medicine to modify genes in a precise and targeted manner. Thanks to the Cas9 protein and a guide RNA, it is possible to identify and cut a specific DNA sequence in the genome responsible for a genetic disease. Once the DNA is cut, the cell repairs the break via two mechanisms: a) Non-homologous end joining (NHEJ): a natural DNA repair mechanism used by cells to repair double-strand breaks. It directly joins the broken ends without using a template, which is fast but often imprecise, leading to insertions or deletions (mutations). This process can inactivate genes or cause non-specific modifications. b) Homology-directed repair (HDR): a precise DNA repair mechanism that uses a homologous DNA template to correct double-strand breaks. This template can be neighboring DNA or an artificial fragment introduced by scientists, allowing specific sequences to be inserted or replaced. This process is more precise but requires specific conditions and occurs less frequently than NHEJ. Methods: 1. Combined Cas9/sgRNA plasmid: - A single plasmid contains the gene coding for the Cas9 protein and the one coding for the simplified guide RNA (sgRNA). - The transcription of Cas9 is under the control of a Pol-II promoter, adapted to express proteins, while the sgRNA is under the control of a Pol-III promoter, specific for transcribing small structural RNAs such as guides. - Result: mutation. - Mainly used to inactivate a gene (by random breakage and repair with NHEJ). 2. Cas9 plasmid + sgRNA gene fragment: - Two components are used: a plasmid carrying Cas9 and a fragment coding for the sgRNA - This method can be used to repair an existing mutation or to introduce a new mutation because a template has been incorporated. 3. In vitro transcribed Cas9 and sgRNA: - Cas9 and sgRNA are directly produced in vitro. - Cas9 is expressed as a functional protein, and sgRNA is synthesized into mature RNA - Both are then injected into the cell, bypassing transcriptional expression steps. - The complex Cas9-sgRNA disappears after its action, thus limiting the risks of toxicity, off-target cuts, or unwanted integration. 1. The sgRNA contains a 20-nucleotide guide region (in green) that pairs complementary to the target sequence in the DNA. 2. The Cas9 protein also recognizes a short neighboring sequence called PAM (Protospacer Adjacent Motif) (here TGG, in red). 3. Once the sgRNA is attached to the target, Cas9 cuts the two strands of DNA just before the PAM sequence, creating a double-strand break. The problem with CRISPR is that it is not absolutely precise because Cas-9 can cut other regions that we do not want to target, difficult to identify because we do not know where they occur. It is possible to mutate the Cas9 protein to suppress its cleavage activity at all potential sites, which helps limit accidental or off-target cuts in the genome. By modifying Cas9 to target only one specific gene (NYC, for example), this allows all activity to be concentrated on a specific gene, without affecting other regions. The chromosome remains intact (no double-strand breaks), which reduces the risk of adverse effects and minimizes problems related to random repairs. In addition, by completely mutating Cas9 so that it loses all cleavage capacity, we obtain an inactive version called dCas9 (dead Cas9). This version can still bind to DNA thanks to sgRNA, but does not cut. Specific effectors can then be attached to dCas9 for targeted applications: a) dCas9 with a transcriptional activator: Allows to increase the expression of a specific gene by guiding the activator to a specific promoter or regulatory region. b) dCas9-DNMT: When fused to a methyltransferase enzyme, dCas9 can add methylation marks to specific regions of DNA, thereby epigenetically altering gene expression. c) dCas9-TET: When fused to a demethylase enzyme, dCas9 can remove methylation marks from specific regions, potentially reactivating silent genes. Problems and Complications If dead Cas9 (dCas9) is used coupled with a transcriptional activator domain to target a promoter region, this can activate the expression of a target gene. However, to obtain efficient activation, it is necessary to target several gRNAs at the same time (multiplexed RNA), because a single gRNA is generally not enough to produce significant activation. The main problem is that several plasmids must be introduced into the cells: 4 plasmids to express the gRNAs, plus a 5th plasmid to express dCas9. Another solution is to design a single plasmid that contains both gRNA and dCas9, but this is still complex. In both cases, the difficulty lies in the efficiency of delivery of the plasmids into the cells, which often remains very low, thus limiting the overall efficiency of the system. To solve this problem: - One solution considered is to express the gRNAs from a Pol-II promoter. However, this poses a problem of polyadenylation (addition of a poly-A tail) which interferes with the functioning of the gRNAs, although Pol-II promoters are advantageous because they are expressed in almost all cells. - A solution proposed by your professor is to use a fluorescent protein linked to an RNA allowing the addition of a poly-A8 tail (similar to the bacterial mechanism) - Another attempt was to design gRNAs as introns inserted into a Pol-II transcript, so that they would be generated after splicing. However, this approach did not work, because the processing of the introns damaged the RNA, rendering the gRNAs nonfunctional. miRNA - RNA interference (RNAi), discovered by Andrew Fire and Craig Mello in 2006 in C. elegans, is a natural gene silencing mechanism using double-stranded RNAs to inhibit gene expression - In eukaryotes, 30–70% of genes are regulated by small inhibitory RNAs, acting at the transcription or translation levels. - These RNAs can be of endogenous (such as microRNAs) or exogenous (such as small interfering RNAs) origin. Synthesis 1. The miRNA gene is transcribed into pri-miRNA, a long RNA molecule forming a stem-loop structure. This is the “barcode” of the gene that we are trying to silence. 2. In the nucleus, the Drosha-DGCR8 complex cuts the pri-miRNA to produce a shorter precursor called pre-miRNA. 3. The pre-miRNA is transported out of the nucleus to the cytoplasm by protein Exportin-5 4. In the cytoplasm, the enzyme Dicer cuts the pre-miRNA to generate a miRNA-miRNA* duplex (two complementary RNA strands). 5. One of the two strands of the duplex is incorporated into the RISC complex (RNA-induced silencing complex) containing the Ago2 protein. 6. If the miRNA pairs perfectly with the target mRNA, the RISC cleaves the mRNA, blocking its translation. If the pairing is imperfect, the miRNA inhibits translation without degrading the mRNA. This step serves to turn off certain genes at the right time for the cell to function properly and adapt to its environment. It is like a switch that controls the production of proteins by targeting mRNAs. The miRNA does not need to pair with the entire mRNA. It pairs in a complementary manner with a specific region of the target mRNA, called the "Seed Sequence" (positions 2 to 8 of the miRNA). This region is super important because it allows targeting. The Flank parts on either side of the seed sequence play an accessory role in the stability of the interaction. In addition, G:U mismatches (wobble) are tolerated and influence the efficiency of inhibition. Target sequences are short and can tolerate mismatches, so each microRNA (miRNA) has the ability to regulate many mRNAs (messenger RNAs), and conversely, each mRNA can be targeted by several different miRNAs. This creates a huge regulatory network in cells. Summary miRNA: Primary transcripts (pri-miRNA) - Encoded by Pol2/Pol3 promoters. - Present in exons and introns. Digested twice - Recognition is structural! Drosha - Digests the pri-miRNA. - Separates the stem-loop structure. - Produces a 65-70 base pair pre-miRNA. - The pre-miRNA is exported into the cytoplasm. Dicer - Digests the pre-miRNA. - Produces a mature miRNA of 21-22 base pairs. RISC (RNA-Induced Silencing Complex) - Perfect pairing: Degradation of mRNA. - Imperfect pairing: Inhibition of translation. siRNA siRNA (small interfering RNA) is a small double-stranded RNA that, unlike miRNA, mainly originates from exogenous sources such as viruses or transposons, although it can also be of endogenous origin. Unlike miRNA, siRNA does not need to go through the Drosha processing step in the nucleus; it is directly cleaved by Dicer in the cytoplasm to produce fragments of 21-25 nucleotides. Once loaded into the RISC complex (like miRNA), siRNA perfectly pairs with its target sequence on mRNA, usually leading to direct degradation of the mRNA. In contrast, miRNA can tolerate mismatches and often inhibits translation rather than directly degrading mRNA. Therefore, siRNA is more specific in its action, while miRNA often regulates multiple targets due to its partial complementarity. Recognition and binding strength The identification of genomic miRNAs is based on the prediction of their secondary structure, in particular by identifying hairpins (stem-loops). Then, the prediction of their homology with their targets is mainly based on the seed sequence, a key region for recognition. The human genome contains more than 1000 genes encoding miRNAs, and each of them can potentially regulate hundreds of targets. The targeting efficiency of miRNAs (binding strength) mainly depends on two factors: - The Seed Sequence: the binding is stronger if it is 8 nucleotides compared to 6, and the G:U wobble weakens the binding. If the mismatch is at the end of the seed, it affects the link less than if it is in the middle. - The number of binding sites present on the target mRNA. If 8 nucleotides is better than 7, which is better than 6… Evolutionary Origins 1. Probably protection against transposons and viruses - 45% of the human genome is foreign DNA! - Transposons (mobile genetic elements) and viruses represent a large part of human DNA. siRNAs and miRNAs are thought to have evolved to protect the genome by targeting and reducing the expression of these foreign elements, thus preventing their proliferation or deleterious effects. 2. RNA editing and the role of miRNAs - Destabilization of double-stranded RNAs (dsRNAs) from viruses/retroelements: miRNAs and siRNAs recognize and cleave double-stranded RNAs produced by viruses or retroelements. This prevents their translation into functional proteins, thereby limiting their infectious activity or integration into the genome. - Targeting virus/retroelement expression: miRNAs specifically regulate genes associated with retroelements, reducing their impact on genome stability. 3. MicroRNAs are likely to have evolved to fine-tune endogenous gene expression - Although they likely first emerged as a defense against genetic invaders, miRNAs have subsequently become key regulators of endogenous gene expression. miRNA-Dependent Regulation: 1. Tissue-Specific miRNAs: - Crucial for embryonic development, maintenance of cell identity, and fine-tuning of gene expression. - Dicer suppression leads to embryonic lethality. 2. Cancer: - Decrease in tumor suppressor miRNAs. - Increase in onco-miRNAs promoting tumor growth. miRNA sponges are molecules (non-coding RNAs or artificial RNAs) that act as "sponges" by binding to miRNAs via complementary sequences. They prevent miRNAs from interacting with their natural targets (mRNAs), blocking their regulatory function. This can modulate the expression of genes regulated by miRNAs. Example of application: 1. A reporter gene (lacZ) is fused to a target sequence complementary to miR-196a. 2. When the miRNA is present and active, it cleaves or inhibits the expression of lacZ, reducing the blue coloration. 3. This allows visualization of the areas where miR-196a is expressed, revealing its spatial and temporal role in embryonic development. The miRNA does not completely silence the genes it targets; it acts rather as a fine tuning regulator. Even in the case of a perfect match with the target mRNA, it reduces expression by about 95%. This percentage decreases further in the presence of mismatches in the target sequence. Ribozymes - Ribozymes are catalytic RNA molecules capable of cleaving RNA targets in cis (same molecule) or trans (different molecule). - They play an essential role in processes such as RNA splicing, viral replication, and tRNA biosynthesis. 1. The ribozyme binds to the target mRNA via base pairing at a specific region. 2. Once attached, it catalyzes a cleavage reaction of the phosphodiester backbone at a specific cleavage site. 3. This cleavage results in the fragmentation of the mRNA into two pieces, preventing its translation into protein. 4. After the reaction, the ribozyme remains intact and can act on other target mRNA molecules, thereby acting catalytically to effectively regulate or inhibit the expression of the targeted genes.