Sensory System 2, Vision PDF
Document Details
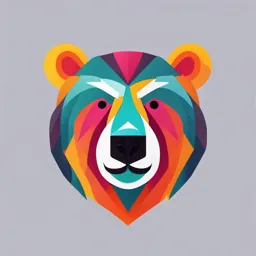
Uploaded by ProfuseKoala6986
University of Pécs
Tags
Summary
This document describes the main refractive media of the eye and their role in focusing light, including the cornea, aqueous humor, lens, and vitreous body. It explains diopters as a unit of measurement for refractive power and how their differences lead to different vision types such as myopia (nearsightedness) and hyperopia (longsightedness). It also touches on how accommodation works to adjust to different viewing distances.
Full Transcript
SENSORY SYSTEM 2 VISION Main Refractive Media of the Eye: Cornea: This is the first part of the eye that light encounters. It plays a crucial role in focusing light onto the retina. Humor Aquaeus (Aqueous Humor): The fluid between the cornea and the lens, which helps maint...
SENSORY SYSTEM 2 VISION Main Refractive Media of the Eye: Cornea: This is the first part of the eye that light encounters. It plays a crucial role in focusing light onto the retina. Humor Aquaeus (Aqueous Humor): The fluid between the cornea and the lens, which helps maintain the eye's shape and refracts light. Eyepiece: Likely referring to the lens of the eye, which further focuses the light. Corpus Vitreum (Vitreous Body): The transparent gel that fills the space between the lens and the retina, also helping with refraction. A diopter is the unit used to measure the refractive power of a lens. It’s the reciprocal of the focal length in meters. For example, a 2-diopter lens has a focal length of 0.5 meters. Cornea Refractive Power: The cornea typically has a refractive power between 40-43 diopters. Lens Refractive Power: The lens of the eye typically has a refractive power between 17-20 diopters, which allows it to adjust focus for near or far objects. A diopter is a way to measure how powerful a lens is in bending light. Think of it like a "strength level" for lenses. Here's an easy way to understand it: Focal Length: Every lens has a focal point, which is where the light rays converge to form a clear image. The focal length is the distance from the lens to that point where light comes together. This distance is measured in meters. Diopter Formula: o The diopter (D) is just the reciprocal (or 1 divided by) of the focal length in meters. o So, if a lens has a focal length of 1 meter, its diopter is 1/1 = 1 diopter. o If the lens is stronger and bends light more, the focal length gets shorter. For example, if the focal length is 0.5 meters, then the diopter is 1/0.5 = 2 diopters. Example to Simplify: Imagine you have a magnifying glass. If it focuses light from 2 meters away, the diopter is 1/2 = 0.5 diopters. If it focuses light from just 0.25 meters away (closer and stronger), the diopter is 1/0.25 = 4 diopters. Why Diopters Matter for the Eye: Cornea and Lens: Both parts of your eye have refractive power, which is measured in diopters. o The cornea is very powerful in bending light (about 40-43 diopters). o The lens can adjust its shape to change its diopters (17-20), helping you focus on things both near and far. If the refractive power (diopters) of your eye isn't right, you might need glasses or contact lenses to correct your vision, which also have a specific diopter value. Refraction error Emmetropia (Normal Vision): In a normal eye (emmetropia), distant light rays are focused directly on the retina, allowing for clear vision. Myopia (Nearsightedness): In a myopic eye, light rays from distant objects are focused in front of the retina, causing blurred vision for distant objects. Correction: A concave (spreading) lens is used to spread the light rays, pushing the focus back onto the retina. Hyperopia (Farsightedness): In a hyperopic eye, light rays are focused behind the retina, making it difficult to see near objects. Correction: A convex (collecting) lens is used to converge the light rays earlier, bringing the focus onto the retina. Astigmatism is caused by an irregular curvature of the cornea or lens, leading to light being focused unevenly on the retina. This means that different parts of the image are focused either in front of or behind the retina, resulting in blurred or distorted vision. It affects both near and distant vision Lens-Induced Defocus: Myopic Defocus: Occurs when light is focused in front of the retina. Hyperopic Defocus: Occurs when light is focused behind the retina. ACCOMODATION Accommodation is the eye’s ability to change the shape of the lens to focus on objects at different distances. When we look at things that are close to us, the lens becomes rounder to increase the refractive power, and when we look at far objects, the lens flattens to decrease the refractive power How Accommodation Works (Muscles and Fibers): Ciliary Muscle: This muscle controls the lens' shape. When the ciliary muscle contracts, the lens becomes rounder, allowing us to focus on close objects. When the ciliary muscle relaxes, the lens flattens to focus on distant objects. Zonular Fibers (Zonula Zinnii): These fibers are attached to the lens. When the ciliary muscle relaxes, the zonular fibers become tense and pull the lens to flatten it (good for distant vision). When the ciliary muscle contracts, the zonular fibers relax, allowing the lens to become rounder (good for close vision). Two States of the Lens During Accommodation: Looking at Far Objects (Unaccommodated Lens): The ciliary muscle is relaxed, which means the zonular fibers are tense and pull the lens to make it flatter. This decreases the refractive power of the lens, allowing the eye to focus on distant objects. Looking at Close Objects (Accommodated Lens): The ciliary muscle is contracted, which makes the zonular fibers relax, and the lens becomes rounder (bulges). This increases the refractive power of the lens, allowing us to focus on nearby objects. Retina and Pathways Retina Overview: The retina is the layer at the back of the eye that is responsible for converting light into neural signals. These signals are then sent to the brain via the optic nerve to form images. Key Cells in the Retina: Photoreceptors: These are the rods and cones, responsible for detecting light. o Rods: Sensitive to low light, important for night vision but don't detect color. o Cones: Responsible for detecting color and fine detail, work best in bright light. Bipolar Cells: These cells receive signals from photoreceptors and pass them on to ganglion cells. Ganglion Cells: Their axons form the optic nerve, which carries visual information to the brain. Horizontal and Amacrine Cells: These cells are involved in lateral pathways, helping process and refine visual signals by connecting across the retina. Pathways: o Vertical Pathway: This is the direct path from the photoreceptor → bipolar cell → ganglion cell, which sends the signal to the brain. o Lateral Pathway: This involves horizontal and amacrine cells, which help modify the signals and contribute to more complex processing of visual information. Signal Processing: When light hits photoreceptors, they reduce their release of neurotransmitters. Some cells respond to this by depolarizing (getting excited, signaling a change), while others hyperpolarize (reducing activity, no signal change). Macula and Fovea: The macula is the central part of the retina responsible for color vision. The fovea centralis is located within the macula and is the region with the highest density of cones, making it the area of sharpest vision. Blind Spot: The blind spot occurs where the optic nerve exits the retina, and there are no photoreceptors here, so this area cannot detect light. Photoreceptors Overview There are two types of photoreceptors in the retina: Rods: Primarily responsible for vision in dim light (night vision, called scotopic vision). Cones: Responsible for vision in bright light (daytime vision, called photopic vision) and for color detection. Property ROD CONE Primarily in the periphery of the Concentrated in the fovea, the LOCATION retina central part of the retina 95% of all photoreceptors Only 5% of photoreceptors ABUNDANCE Rhodopsin: sensitive to dim light, Color Opsins: come in three Opsin (Light-Sensitive Pigment) used in monochromatic (black types (S, M, L) for color and white) vision detection—responsible for color vision 100 to 1: Many rods converge 1 to 1: Each cone connects to its Convergence onto Bipolar Cells onto a single bipolar cell, which own bipolar cell, providing high means more sensitivity but less spatial resolution (fine detail) spatial resolution High sensitivity to dim light, Low sensitivity, requiring bright sensitivity which makes rods ideal for night light for daytime vision vision Sluggish response to light Fast response to light changes Response time changes Responsible for peripheral vision Responsible for central vision, Functional role and detecting light in low-light color discrimination, and fine conditions, but with low spatial spatial resolution in bright light resolution (poor detail) Dark adaptation is the process by which rods adjust to darkness. It takes about 15 minutes for rods to resynthesize rhodopsin (their light-sensitive pigment) after being exposed to light. This is why it takes time to adjust to seeing in a dark room after being in a bright environment. Vision Types: Scotopic Vision (Night Vision): This is colorless vision, dominated by rods. Photopic Vision (Daytime Vision): This is colorful vision, dominated by cones. Summary: Rods are highly sensitive to dim light and are key for night vision but do not detect color or fine detail. They are mostly found in the periphery of the retina. Cones are responsible for detailed, color vision in bright light and are concentrated in the center of the retina (fovea), allowing for sharp vision Phototransduction This slide explains phototransduction, the process by which light is converted into electrical signals in the photoreceptor cells of the retina (rods and cones). Let’s break it down step-by-step: 1. What is cGMP? cGMP (cyclic guanosine monophosphate) is a molecule that plays a key role in the function of photoreceptors (rods and cones). It controls the opening and closing of ion channels in the cell membrane of these photoreceptors. 2. In the Dark (No Light Present): cGMP levels are high inside the photoreceptors. The Na+ (sodium) and Ca2+ (calcium) channels are open due to the presence of cGMP, allowing these ions to enter the cell. This inflow of positive ions keeps the cell in a partially depolarized state (the inside of the cell is less negative). Transmitter Release: In the dark, a lot of neurotransmitter (glutamate) is released from the photoreceptor to the bipolar cells because the cell is depolarized. This is called the dark current. 3. In the Light (When Light Hits the Photoreceptors): Rhodopsin Activation: Light activates rhodopsin, the light-sensitive pigment in rods (similar process occurs in cones with different pigments). Rhodopsin activation triggers a G-protein transduction cascade that activates an enzyme called phosphodiesterase (PDE). Phosphodiesterase breaks down cGMP into 5’-GMP, reducing the levels of cGMP in the photoreceptor. 4. Effects of Low cGMP (in Light): With less cGMP available, the Na+ and Ca2+ channels close, stopping the flow of these positive ions into the cell. However, K+ (potassium) channels remain open, allowing K+ to exit the cell. As a result, the photoreceptor becomes hyperpolarized (the inside of the cell becomes more negative). Transmitter Release Reduced: Due to hyperpolarization, the amount of neurotransmitter (glutamate) released to bipolar cells decreases. This is how the photoreceptor signals to the brain that light is present. 5. Signal Transmission: In the dark (with high cGMP), the rod or cone is constantly releasing neurotransmitter. In the light (with low cGMP), the hyperpolarization reduces neurotransmitter release. Bipolar Cells: These cells are sensitive to changes in neurotransmitter release. Depending on whether glutamate is released or reduced, bipolar cells either depolarize or hyperpolarize, which helps process visual signals. Key Concepts: cGMP regulates the flow of ions in and out of photoreceptor cells. In the dark, cGMP levels are high, ion channels stay open, the cell is depolarized, and neurotransmitter release is high. In the light, cGMP levels drop, ion channels close, the cell becomes hyperpolarized, and neurotransmitter release is reduced. This reduction in transmitter release tells the brain that light has been detected. Summary: Dark Response: High cGMP, Na+ and Ca2+ channels open, depolarization, high neurotransmitter release. Light Response: Low cGMP, Na+ and Ca2+ channels close, hyperpolarization, reduced neurotransmitter release. This process is what allows photoreceptors to convert light into electrical signals that can be sent to the brain for visual processing. CENTRAL SIGNAL PROCESSING 2D Retinal Image → 3D Central Processing: The image captured by the retina is 2-dimensional because the retina is flat. However, the brain processes this 2D information into a 3D perception. This happens through various depth cues, such as differences between the images captured by each eye (binocular vision) and the brain's interpretation of objects based on previous experience. Optic Nerve and Partial Crossing (Optic Chiasm): The optic nerve carries visual information from the retina to the brain. Partial crossing occurs at the optic chiasm, where the nerve fibers from the nasal part of the retina (which sees the outer visual field) cross to the opposite side of the brain. o This means the left visual field (from both eyes) is processed by the right hemisphere, and the right visual field is processed by the left hemisphere. Thalamic Switching (Lateral Geniculate Nucleus - LGN): After crossing, the visual information reaches the lateral geniculate nucleus (LGN) in the thalamus, which acts as a relay center. The LGN processes the incoming signals and passes them on to the primary visual cortex (V1). The thalamus is key for sensory relay, and in the case of vision, it helps organize the signals before sending them to the cortex for further processing. Primary Visual Cortex (V1): The primary visual cortex (V1), also known as Brodmann area 17, is located in the occipital lobe at the back of the brain. V1 receives visual information and starts to process basic aspects like edges, contrasts, and simple shapes. However, it doesn't handle more complex interpretations yet. Lower Levels of the Visual System: The lower visual processing areas (like V1) mainly detect basic visual properties: o Contours: The outlines or edges of objects. o Shape: The form of objects. o Texture: The surface detail. o Movement: Whether the object is in motion. o Color: Basic color information. These lower-level areas don't yet give a full understanding of the image. They only recognize essential features. Parallel Processing and Higher Visual Areas (V2-V5): Visual processing involves parallel processing, meaning that different types of information (such as shape, color, movement) are processed simultaneously but in separate pathways or "channels." Beyond the primary visual cortex, the higher visual areas (V2-V5) help interpret more complex aspects of the image. o V2: Handles further processing of visual edges and object orientation. o V3: Involved in form recognition and dynamic (moving) shapes. o V4: Plays a role in color perception and object recognition. o V5 (also known as MT): Specializes in detecting motion and the speed of moving objects. Summary: Retinal Image is 2D, but central processing turns it into a 3D perception. The optic nerve partially crosses at the optic chiasm, organizing visual fields to be processed by opposite sides of the brain. Visual signals pass through the thalamus (LGN) and reach the primary visual cortex (V1) for basic visual feature detection. The lower levels of the visual system recognize only simple features like contours, shape, texture, movement, and color. Parallel processing ensures that information about different aspects of vision (like color and motion) is processed simultaneously in higher cortical areas (V2-V5). EXPLANATION OF THIS PROCESS in more depth : Vision starts at the retina When light enters your eye, it hits the retina, where specialized cells called photoreceptors (rods and cones) detect this light. The retina processes the light into electrical signals, but the image at this point is two-dimensional (2D). This means that your eye only captures the height and width of objects, not depth (how far or close things are). The brain processes a 3D world Although the image on the retina is 2D, your brain takes this information and processes it into a 3D perception, giving you the sense of depth. It does this by using depth cues, like the different images captured by each eye (called binocular vision) and interpreting things like shadows, object size, and relative motion to understand how far away things are. This transition from 2D to 3D is one of the complex tasks your brain performs when processing visual information. Crossing of signals: Optic nerve and optic chiasm After your retina converts light into electrical signals, these signals travel along the optic nerve toward the brain. The optic nerves from both eyes meet at a point called the optic chiasm. Here, some of the nerve fibers cross over to the opposite side of the brain. Specifically, signals from the nasal (inner) part of your retina, which sees the outer visual field, cross over. This crossover ensures that the left visual field from both eyes is processed by the right hemisphere of the brain, and the right visual field is processed by the left hemisphere. This crossing over of information is important because it helps your brain combine the visual input from both eyes and create a coherent, single image. Thalamus (The Relay Station) After crossing at the optic chiasm, the visual signals continue to a part of the brain called the thalamus. Specifically, they pass through the lateral geniculate nucleus (LGN), which is a relay center for visual information. The LGN acts like a switchboard operator, organizing and refining the visual signals before passing them on to the visual cortex for more detailed processing. Visual Cortex (Processing the basics) Once the signals leave the thalamus, they arrive in the primary visual cortex (V1), located in the occipital lobe at the back of your brain. This is where basic processing begins. Here, the brain starts to detect simple features of the image: Edges and contours: The visual cortex identifies where objects begin and end. Shapes: The overall form of objects is recognized. Texture: Surface details like smoothness or roughness are identified. Movement: The brain detects if objects are moving and in what direction. Color: Basic color information is processed here. But at this stage, the brain only sees simple aspects of the image. It still doesn’t "understand" what it’s looking at. Higher levels of processing (V2-V5): Beyond basic vision After the primary visual cortex (V1), the visual information gets sent to higher areas of the brain for further processing. This is where the real "magic" of visual interpretation happens. Different areas of the brain are responsible for different parts of visual processing: V2: Handles more complex edges, angles, and shapes, building on the basic contour information from V1. V3: Helps with detecting forms and dynamic shapes, which is crucial when objects are moving. V4: Specializes in color processing and more detailed object recognition. It helps you understand what objects are based on their color and shape. V5 (MT): Is primarily responsible for detecting motion and the speed at which objects are moving. What’s important here is that the brain uses parallel processing, meaning it processes different aspects of the visual information (like color, shape, and motion) at the same time, but in different areas of the brain. This allows the brain to quickly make sense of what you’re seeing. Summary of the process in simpler terms: When light hits your eye, the retina converts it into electrical signals. These signals are sent to the brain, where they are first passed through the optic chiasm, then relayed by the thalamus. The signals reach the primary visual cortex (V1), which detects simple details like edges, shapes, movement, and color. From there, the information moves to more specialized areas of the brain that process these details in parallel, allowing you to see a complete, 3D image with depth, color, and motion. This process is fast and complex, but it’s how your brain transforms light hitting the retina into the rich visual world you experience. This image focuses on the Corpus Geniculatum Laterale (Lateral Geniculate Nucleus, or LGN), a key part of the thalamus that acts as a relay center for visual information traveling from the retina to the brain. Yes, it is very much related to central signal processing, as it plays a crucial role in transmitting and refining the visual information before it reaches the primary visual cortex (V1). 1. 6 Layers of the LGN: The lateral geniculate nucleus (LGN) is organized into six layers, each of which processes different aspects of visual information: Retinotopic Organization: This means that each layer of the LGN maintains a spatial map of the visual field, so that information from neighboring regions of the visual field is processed in neighboring regions of the LGN. Concentric Receptive Fields: Each neuron in the LGN responds to specific parts of the visual field. These fields are typically organized in a concentric pattern, meaning they respond to light in circular areas. 2. Types of Neurons in the LGN: The LGN has different types of neurons that process specific aspects of visual input: Magno Cells (Magnocellular Pathway): These neurons are motion-sensitive and respond to large, fast- moving objects. They process black-and-white, coarse, and fast-moving stimuli. Parvo Cells (Parvocellular Pathway):These neurons are color-sensitive and are responsible for high- resolution color vision and the fine details of objects. Konio Cells (Koniocellular Pathway):These neurons are involved in processing blue color and eye movements. 3. Interneurons and Relay Cells: Interneurons are small neurons within the LGN that help modulate and refine the visual signals as they pass through. Relay Cells are the main output neurons that send visual information to the primary visual cortex (V1) after it has been processed by the LGN. 4. Visual Pathways in the LGN: The diagram shows the optic nerves from the left and right eyes, with fibers crossing at the optic chiasm. Visual signals from both eyes are sent to the LGN, where they are processed in parallel: Information from the left visual field (from both eyes) is processed in the right hemisphere, and information from the right visual field is processed in the left hemisphere. The signals are relayed through the LGN to the primary visual cortex for further processing. 5. Feed-back Connections: The LGN does not just passively relay information. It also receives feedback from the higher visual areas in the cortex. These feedback loops may play a role in functions such as: Vigilance: The ability to focus attention on important stimuli. Attention: Choosing which visual information to focus on. Motivation: Affecting what you pay attention to based on your needs or goals. Eye Movement: The LGN helps coordinate eye movements as you track moving objects or shift your gaze. 6. Relationship to Central Signal Processing: Yes, the LGN is a key part of central signal processing. The visual signals from the retina are first processed by the LGN before they are sent to the primary visual cortex (V1). The LGN helps organize and fine-tune these signals based on various visual features, such as motion, color, and contrast. After this initial processing, the signals are sent to the cortex for more complex visual interpretation. Visual Fields Overview: The visual field is the total area in the environment that your eyes can see when you are looking straight ahead and not moving your eyes. Each eye has its own visual field, and this field is divided into two halves: 1. Temporal visual field: The outer half of your field of view (towards your ears). 2. Nasal visual field: The inner half of your field of view (towards your nose). How Light is Projected in the Eye: The way the eye and brain process visual information is a bit tricky because what you see in the visual field is projected onto the retina in the opposite half of each eye: Temporal visual field (objects to the sides) are projected onto the nasal side of the retina. Nasal visual field (objects near your nose or center) are projected onto the temporal side of the retina. What Happens After Light Hits the Retina? Once light hits the photoreceptors in the retina, the ganglion cells process the visual signals and send them through their axons. These axons converge to form the optic nerve (nervus opticus), which carries the visual signals from each eye towards the brain. Crossing of Fibers in the Optic Chiasm (Chiasma Opticum): At a point called the optic chiasm, something important happens: The axons (nerve fibers) from the nasal half of each retina (which detect information from the outer/temporal visual field) cross over to the opposite side of the brain. This crossing is called hemidecussation. The axons from the temporal half of the retina, which detect information from the nasal visual field (the inner half), do not cross at the optic chiasm. They stay on the same side of the brain. This partial crossing of fibers allows the brain to process the visual field information from both eyes together. The brain now has information about the left visual field (from both eyes) being processed by the right side of the brain, and the right visual field being processed by the left side of the brain. Tractus Opticus (Optic Tract): After the optic chiasm, the nerve fibers continue as the optic tract. The optic tract carries the visual information to the lateral geniculate nucleus (LGN) in the thalamus, which acts as a relay center. From there, the visual information is passed on to the primary visual cortex for further processing The primary visual cortex (V1, or Brodmann area 17) is the first place in the brain that processes visual information received from the eyes. This area is critical for detecting basic visual features like orientation, contrast, and edges. Hubel and Wiesel's Discovery: Simple Cells Simple Cells: Neuroscientists Hubel and Wiesel found that some cells in the primary visual cortex (V1) respond to specific features of a visual stimulus, such as the orientation of a bar of light. o Specific Orientation: These cells are highly sensitive to narrow rectangles (bars) of light and respond only when these bars are in a specific orientation (vertical, horizontal, diagonal). For example: ▪ A vertical bar of light might cause the cell to fire strongly (maximum response). ▪ A diagonal bar might cause a weaker response. ▪ A horizontal bar might cause very little or no response. The "spiking" or "firing" refers to the electrical activity of the neuron (its response to a visual stimulus). The cells are highly tuned to recognize specific edges and lines in particular orientations, which are the building blocks of all visual objects. Complex Cells: These cells are more advanced and respond to larger, more complex geometric shapes or movements. They don’t just react to bars of light but also to moving shapes or more intricate patterns. While simple cells detect very basic features like the orientation of lines, complex cells detect more advanced patterns, including movement and shape. After basic processing in the primary visual cortex (V1), visual information is further processed in higher cortical areas (such as V2, V3, V4, MT) to build up a more complete understanding of what you're seeing. This process is often referred to as parallel processing, where different aspects of visual input (like color, motion, and shape) are processed simultaneously in different areas. The Two Main Pathways in the Brain: There are two main pathways by which visual information flows beyond V1: 1. Dorsal Pathway (Parietal Pathway): This is also called the “Where” pathway. o It is responsible for processing the location, movement, and spatial relationships of objects. This pathway helps you figure out where things are in space and how they’re moving. o Key areas: MT (middle temporal area) and MST (medial superior temporal area) are involved in detecting motion. 2. Ventral Pathway (Temporal Pathway): Also called the “What” pathway. o It is responsible for object recognition, color perception, and understanding what things are. This pathway helps you recognize shapes, faces, and colors. o Key areas: V4 is important for processing color and detailed object recognition. Interactions and Feedback: The interaction between these pathways is not completely separated, and there is intermixing between the two streams of processing. This means that even though motion and object recognition are processed separately, they can influence each other. Blockage of Pathways: When certain parts of the visual processing system are blocked (for example, if you interrupt the magnocellular or parvocellular pathways in the LGN), it can affect how visual information is processed: o Blocking the magnocellular pathway (motion-sensitive) leads to increased activity in motion-detecting cells in area MT (motion-related cortex), but other visual areas are less active. o Blocking the parvocellular pathway (color-sensitive) has less of an effect on MT but impacts color and shape perception in areas like V4. The primary visual cortex (V1), also known as Brodmann area 17, processes visual information. This slide discusses some of the essential organizational structures within V1: 1. Orientation Columns: These are groups of neurons in V1 that respond to specific orientations of lines or edges in the visual field. For example, one column may respond best to vertical lines, while another responds to horizontal lines. Each orientation column is sensitive to the same position in the visual field but only reacts when the line or edge has a particular orientation (like a vertical bar). 2. Ocular Dominance Columns: These columns represent the input from each eye separately. Information from the right and left eye alternates across these columns, allowing the brain to compare the signals from both eyes for depth perception. 3. Hypercolumns: A hypercolumn is a larger unit in V1 that contains many orientation and ocular dominance columns. Each hypercolumn processes all the possible orientations of edges for a particular part of the visual field. It contains all the orientation columns and ocular dominance columns needed to fully process what both eyes are seeing at a specific location in space. Hypercolumns are like small processing units that contain all the information needed to understand both the orientation and depth of objects. Color-Sensitive Blobs: Within hypercolumns, there are also color-sensitive blobs that process color information. This slide focuses on how the brain processes shapes and movements beyond the primary visual cortex, involving different areas of the brain. 1. Pathways of Visual Processing: After V1, visual information is processed through two major pathways: Dorsal Pathway (also known as the "Where" pathway): o This pathway processes information related to motion and spatial awareness (e.g., where an object is in space and how it is moving). o It travels to the parietal lobe and is associated with areas like MT (middle temporal area), which detects motion. o For motion processing, the flow of information is: V1 → V2 → V3 → V5. Ventral Pathway (also known as the "What" pathway): o This pathway processes shape and object recognition (e.g., what an object is). o It travels to the inferior temporal lobe, including regions like V4, which is essential for color and shape recognition. o For shape processing, the flow of information is: V1 → V2 → V4 → Inferior Temporal Lobe. This slide highlights the sensitivity of different photoreceptors (cones and rods) to various wavelengths of light, which allows us to perceive different colors. 1. Cone Types and Light Absorption: Cones are responsible for color vision, and there are three types of cones, each sensitive to a specific range of wavelengths: o Blue Cones (Short Wavelength): Sensitive to light at around 420 nm (violet and blue). o Green Cones (Medium Wavelength): Sensitive to light at around 534 nm (green). o Red Cones (Long Wavelength): Sensitive to light at around 564 nm (yellow and red). Rods are responsible for vision in low light conditions and are most sensitive at around 498 nm, but they do not contribute to color vision. Instead, rods help with black-and-white vision in dim light. 2. Visual Pigments: Visual pigments in photoreceptors (rods and cones) undergo changes when they absorb light. This process triggers the conversion of light into electrical signals that can be sent to the brain. o Rhodopsin: The pigment in rods. o Opsins: Pigments in cones responsible for color vision. They are sensitive to different wavelengths depending on the type of cone (short, medium, or long). Summary of the Process: Rhodopsin is the pigment in rods that captures light. When rhodopsin absorbs light, it goes through several intermediate stages: bathorhodopsin, lumirhodopsin, metarhodopsin I, and metarhodopsin II. Metarhodopsin II is the active form that triggers the signaling pathway, eventually sending visual information to the brain. After this, rhodopsin releases trans-retinal, which must be recycled to regenerate cis-retinal for continued vision.