Scientific Knowledge GED.docx
Document Details
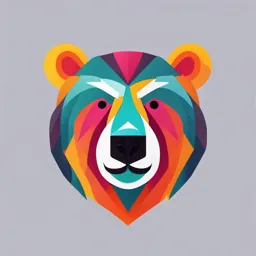
Uploaded by PanoramicRoseQuartz
Full Transcript
Scientific knowledge refers to any topic that is studied empirically, meaning that it is based on observation of a phenomenon in an objective way. The body of scientific knowledge is often broken down into several domains including biology, ecology, Earth science, space science, physics, and chemist...
Scientific knowledge refers to any topic that is studied empirically, meaning that it is based on observation of a phenomenon in an objective way. The body of scientific knowledge is often broken down into several domains including biology, ecology, Earth science, space science, physics, and chemistry. These each have further subdomains and are overlapping in many ways. For instance, ecology is the study of ecosystems, which are made up of biological factors and geological factors, so it contains elements of both biology and Earth science. Each of these domains is subject to the concepts of scientific inquiry, such as the scientific method, scientific facts, hypotheses, and scientific laws. Important Terminology · A phenomenon is an event or effect that is observed. · A scientific fact is considered an objective and verifiable observation. Usually, a fact can be repeated or demonstrated to others. · A scientific theory is a proposition explaining why or how something happens and is built on scientific facts and laws. Scientific theories can be tested, but are not fully proven. If new evidence is found that disproves the theory, it is no longer considered true. · A hypothesis is an educated guess that is not yet proven. It is used to predict the outcome of an experiment in an attempt to solve a problem or answer a question. · A law is an explanation of events that always leads to the same outcome. It is a fact that an object falls. The law of gravity explains why an object falls. The theory of relativity, although generally accepted, has been neither proven nor disproved. · A model is used to explain something on a smaller scale or in simpler terms to provide an example. It is a representation of an idea that can be used to explain events or applied to new situations to predict outcomes or determine results. When one examines the history of scientific knowledge, it is clear that it is constantly evolving. The body of facts, models, theories, and laws grows and changes over time. In other words, one scientific discovery leads to the next. Some advances in science and technology have important and long-lasting effects on science and society. Some discoveries were so alien to the accepted beliefs of the time that not only were they rejected as wrong, but were also considered outright blasphemy. Today, however, many beliefs once considered incorrect have become an ingrained part of scientific knowledge, and have also been the basis of new advances. Examples of advances include: Copernicus's heliocentric view of the universe, Newton's laws of motion and planetary orbits, relativity, geologic time scale, plate tectonics, atomic theory, nuclear physics, biological evolution, germ theory, industrial revolution, molecular biology, information and communication, quantum theory, galactic universe, and medical and health technology. Scientific Inquiry and Scientific Method Scientists use a number of generally accepted techniques collectively known as the scientific method. The scientific method generally involves carrying out the following steps: · Identifying a problem or posing a question · Formulating a hypothesis or an educated guess · Conducting experiments or tests that will provide a basis to solve the problem or answer the question · Observing the results of the test · Drawing conclusions An important part of the scientific method is using acceptable experimental techniques. Objectivity is also important if valid results are to be obtained. Another important part of the scientific method is peer review. It is essential that experiments be performed and data be recorded in such a way that experiments can be reproduced to verify results. Historically, the scientific method has been taught with a more linear approach, but it is important to recognize that the scientific method should be a cyclical or recursive process. This means that as hypotheses are tested and more is learned, the questions should continue to change to reflect the changing body of knowledge. One cycle of experimentation is not enough. The metric system is the accepted standard of measurement in the scientific community. The International System of Units (SI) is a set of measurements (including the metric system) that is almost globally accepted. The United States, Liberia, and Myanmar have not accepted this system. Standardization is important because it allows the results of experiments to be compared and reproduced without the need to laboriously convert measurements. The SI is based partially on the meter-kilogram-second (MKS) system rather than the centimeter-gram-second (CGS) system. The MKS system considers meters, kilograms, and seconds to be the basic units of measurement, while the CGS system considers centimeters, grams, and seconds to be the basic units of measurement. Under the MKS system, the length of an object would be expressed as 1 meter instead of 100 centimeters, which is how it would be described under the CGS system. The mathematical concept of significant figures or significant digits is often used to determine the accuracy of measurements or the level of confidence one has in a specific measurement. The significant figures of a measurement include all the digits known with certainty plus one estimated or uncertain digit. There are a number of rules for determining which digits are considered “important” or “interesting.” They are: all non-zero digits are significant, zeros between digits are significant, and leading and trailing zeros are not significant unless they appear to the right of the non-zero digits in a decimal. For example, in 0.01230 the significant digits are 1230, and this number would be said to be accurate to the hundred-thousandths place. The zero indicates that the amount has actually been measured as 0. Other zeros are considered place holders, and are not important. A decimal point may be placed after zeros to indicate their importance (in 100. for example). Estimating, on the other hand, involves approximating a value rather than calculating the exact number. This may be used to quickly determine a value that is close to the actual number when complete accuracy does not matter or is not possible. In science, estimation may be used when it is impossible to measure or calculate an exact amount, or to quickly approximate an answer when true calculations would be time consuming. Errors that occur during an experiment can be classified into two categories: random errors and systematic errors. Random errors can result in collected data that is wildly different from the rest of the data, or they may result in data that is indistinguishable from the rest. Random errors are not consistent across the data set. In large data sets, random errors may contribute to the variability of data, but they will not affect the average. Random errors are sometimes referred to as noise. They may be caused by a student’s inability to take the same measurement in exactly the same way or by outside factors that are not considered variables, but influence the data. A systematic error will show up consistently across a sample or data set, and may be the result of a flaw in the experimental design. This type of error affects the average, and is also known as bias. Scientific notation is used because values in science can be very large or very small, which makes them unwieldy. A number in decimal notation is 93,000,000. In scientific notation, it is . The first number, 9.3, is the coefficient. It is always greater than or equal to 1 and less than 10. This number is followed by a multiplication sign. The base is always 10 in scientific notation. If the number is greater than ten, the exponent is positive. If the number is between zero and one, the exponent is negative. The first digit of the number is followed by a decimal point and then the rest of the number. In this case, the number is 9.3, and the decimal point was moved seven places to the right from the end of the number to get 93,000,000. The number of places moved, seven, is the exponent. Statistical Terminology Mean - The average, found by taking the sum of a set of numbers and dividing by the number of numbers in the set. Median - The middle number in a set of numbers sorted from least to greatest. If the set has an even number of entries, the median is the average of the two in the middle. Mode - The value that appears most frequently in a data set. There may be more than one mode. If no value appears more than once, there is no mode. Range - The difference between the highest and lowest numbers in a data set. Standard deviation - Measures the dispersion of a data set or how far from the mean a single data point is likely to be. Regression analysis - A method of analyzing sets of data and sets of variables that involves studying how the typical value of the dependent variable changes when any one of the independent variables is varied and the other independent variables remain fixed. The laws of thermodynamics are generalized principles dealing with energy and heat. · The zeroth law of thermodynamics states that two objects in thermodynamic equilibrium with a third object are also in equilibrium with each other. Being in thermodynamic equilibrium basically means that different objects are at the same temperature. · The first law deals with conservation of energy. It states that neither mass nor energy can be destroyed; only converted from one form to another. · The second law states that the entropy (the amount of energy in a system that is no longer available for work or the amount of disorder in a system) of an isolated system can only increase. The second law also states that heat is not transferred from a lower-temperature system to a higher-temperature one unless additional work is done. The third law of thermodynamics states that as temperature approaches absolute zero, entropy approaches a constant minimum. It also states that a system cannot be cooled to absolute zero. Heat is energy transfer (other than direct work) from one body or system to another due to thermal contact. Everything tends to become less organized and less orderly over time (entropy). In all energy transfers, therefore, the overall result is that the energy is spread out uniformly. This transfer of heat energy from hotter to cooler objects is accomplished by conduction, radiation, or convection. Temperature is a measurement of an object’s stored heat energy. More specifically, temperature is the average kinetic energy of an object’s particles. When the temperature of an object increases and its atoms move faster, kinetic energy also increases. Temperature is not energy since it changes and is not conserved. Thermometers are used to measure temperature. Mass — Mass is a measure of the amount of substance in an object. Weight — Weight is a measure of the gravitational pull of Earth on an object. Volume — Volume is a measure of the amount of space occupied. There are many formulas to determine volume. For example, the volume of a cube is the length of one side cubed ( ) and the volume of a rectangular prism is length times width times height ( ). The volume of an irregular shape can be determined by how much water it displaces. Thermal contact refers to energy transferred to a body by a means other than work. A system in thermal contact with another can exchange energy with it through the process of heat transfer. Thermal contact does not necessarily involve direct physical contact. Heat is energy that can be transferred from one body or system to another without work being done. Everything tends to become less organized and less useful over time (entropy). In all energy transfers, therefore, the overall result is that the heat is spread out so that objects are in thermodynamic equilibrium and the heat can no longer be transferred without additional work. Models that can be used to explain the flow of electric current, potential, and circuits include water, gravity, and roller coasters. For example, just as a mass can have a potential for energy based on its location, so can a charge within an electrical field. Just as a force is required to move an object uphill, a force is also required to move a charge from a low to high potential. Another example is water. Water does not flow when it is level. If it is lifted to a point and then placed on a downward path, it will flow. A roller coaster car requires work to be performed to transport it to a point where it has potential energy (the top of a hill). Once there, gravity provides the force for it to flow (move) downward. If either path is broken, the flow or movement stops or is not completed. The motions of subatomic structures (nuclei and electrons) produce a magnetic field. It is the direction of the spin and orbit that indicates the direction of the field. The strength of a magnetic field is known as the magnetic moment. As electrons spin and orbit a nucleus, they produce a magnetic field. Pairs of electrons that spin and orbit in opposite directions cancel each other out, creating a net magnetic field of zero. Materials that have an unpaired electron are magnetic. Those with a weak attractive force are referred to as paramagnetic materials, while ferromagnetic materials have a strong attractive force. A diamagnetic material has electrons that are paired and, therefore, does not typically have a magnetic moment. There are, however, some diamagnetic materials that have a weak magnetic field. Movement of electric charge along a path between areas of high electric potential and low electric potential, with a resistor or load device between them, is the definition of a simple circuit. It is a closed conducting path between the high and low potential points, such as the positive and negative terminals on a battery. One example of a circuit is the flow from one terminal of a car battery to the other. The electrolyte solution of water and sulfuric acid provides work in chemical form to start the flow. A frequently used classroom example of circuits involves using a D cell (1.5 V) battery, a small light bulb, and a piece of copper wire to create a circuit to light the bulb. A magnet is a piece of metal, such as iron, steel, or magnetite (lodestone) that can affect another substance within its field of force that has like characteristics. Magnets can either attract or repel other substances. Magnets have two poles: north and south. Like poles repel and opposite poles (pairs of north and south) attract. The magnetic field is a set of invisible lines representing the paths of attraction and repulsion. Magnetism can occur naturally, or ferromagnetic materials can be magnetized. Certain matter that is magnetized can retain its magnetic properties indefinitely and become a permanent magnet. Other matter can lose its magnetic properties. For example, an iron nail can be temporarily magnetized by stroking it repeatedly in the same direction using one pole of another magnet. Once magnetized, it can attract or repel other magnetically inclined materials, such as paper clips. Dropping the nail repeatedly will cause it to lose its charge. A magnetic field can be formed not only by a magnetic material, but also by electric current flowing through a wire. When a coiled wire is attached to the two ends of a battery, for example, an electromagnet can be formed by inserting a ferromagnetic material such as an iron bar within the coil. When electric current flows through the wire, the bar becomes a magnet. If there is no current, the magnetism is lost. A magnetic domain occurs when the magnetic fields of atoms are grouped and aligned. These groups form what can be thought of as miniature magnets within a material. This is what happens when an object like an iron nail is temporarily magnetized. Prior to magnetization, the organization of atoms and their various polarities are somewhat random with respect to where the north and south poles are pointing. After magnetization, a significant percentage of the poles are lined up in one direction, which is what causes the magnetic force exerted by the material. Motion is a change in the location of an object and is the result of an unbalanced net force acting on the object. Understanding motion requires an understanding of three basic quantities: displacement, velocity, and acceleration. When something moves from one place to another, it has undergone displacement. Displacement along a straight line is a very simple example of a vector quantity. If an object travels from position to , it has undergone a displacement of 10 cm. If it traverses the same path in the opposite direction, its displacement is -10 cm. A vector that spans the object’s displacement in the direction of travel is known as a displacement vector. Sound is a pressure disturbance that moves through a medium in the form of mechanical waves, which transfer energy from one particle to the next. Sound requires a medium to travel through, such as air, water, or other matter since it is the vibrations that transfer energy to adjacent particles, not the actual movement of particles over a great distance. Sound is transferred through the movement of atomic particles, which can be atoms or molecules. Waves of sound energy move outward in all directions from the source. Sound waves consist of compressions (particles are forced together) and rarefactions (particles move farther apart and their density decreases). A wavelength consists of one compression and one rarefaction. Different sounds have different wavelengths. Sound is a form of kinetic energy. Pitch — Pitch is the quality of sound determined by frequency. For example, a musical note can be tuned to a specific frequency. A, for instance, has a frequency of 440 Hz, which is a higher frequency than middle C. Humans can detect frequencies between about 20 Hz to 20,000 Hz. Loudness — Loudness is a human’s perception of sound intensity. Sound intensity — Sound intensity is measured as the sound power per unit area and can be expressed in decibels. Timbre — This is a human’s perception of the type or quality of sound. Oscillation — This is a measurement, usually of time, against a basic value, equilibrium, or rest point. The Doppler effect refers to the effect the relative motion of the source of the wave and the location of the observer has on waves. The Doppler effect is easily observable in sound waves. What a person hears when a train approaches or a car honking its horn passes by are examples of the Doppler effect. The pitch of the sound is different not because the emitted frequency has changed, but because the received frequency has changed. The frequency is higher (as is the pitch) as the train approaches, the same as emitted just as it passes, and lower as the train moves away. This is because the wavelength changes. The Doppler effect can occur when an observer is stationary, and can also occur when two trains approach and pass each other. Electromagnetic waves are also affected in this manner. The motion of the medium can also affect the wave. For waves that do not travel in a medium, such as light waves, it is the difference in velocity that determines the outcome. Waves have energy and can transfer energy when they interact with matter. Although waves transfer energy, they do not transport matter. They are a disturbance of matter that transfers energy from one particle to an adjacent particle. There are many types of waves, including sound, seismic, water, light, micro, and radio waves. The two basic categories of waves are mechanical and electromagnetic. Mechanical waves are those that transmit energy through matter. Electromagnetic waves can transmit energy through a vacuum. A transverse wave provides a good illustration of the features of a wave, which include crests, troughs, amplitude, and wavelength. Light is the portion of the electromagnetic spectrum that is visible because of its ability to stimulate the retina. It is absorbed and emitted by electrons, atoms, and molecules that move from one energy level to another. Visible light interacts with matter through molecular electron excitation (which occurs in the human retina) and through plasma oscillations (which occur in metals). Visible light is between ultraviolet and infrared light on the spectrum. The wavelengths of visible light cover a range from 380 nm (violet) to 760 nm (red). Different wavelengths correspond to different colors. Reflection is the rebound of a light wave from a surface back toward the medium from where it came. A light wave that hits a reflecting surface at a 90-degree angle retraces its original path back to its source. Striking the surface at any other angle results in reflection of the wave at an angle in the opposite direction. Reflectance is the amount of light a material reflects; metals have high reflectance. The smoother a surface, the higher its reflectance. Refraction is the change in the direction of a light wave when it passes through a transparent medium with a different optical density from the one in which the wave had been traveling. This results in a change in the wave’s velocity. The ratio of the sine of the angle of the incoming ray to the sine of the angle of refraction is equal to the ratio of the speed of light in the original medium to the speed of light in the refracting medium. There have been many revisions to theories regarding the structure of atoms and their particles. Part of the challenge in developing an understanding of matter is that atoms and their particles are too small to be seen. It is believed that the first conceptualization of the atom was developed by Democritus in 400 B.C. Some of the more notable models are the solid sphere or billiard ball model postulated by John Dalton, the plum pudding or raisin bun model by J.J. Thomson, the planetary or nuclear model by Ernest Rutherford, the Bohr or orbit model by Niels Bohr, and the electron cloud or quantum mechanical model by Louis de Broglie and Erwin Schrodinger. Rutherford directed the alpha scattering experiment that discounted the plum pudding model. The shortcoming of the Bohr model was the belief that electrons orbited in fixed rather than changing ecliptic orbits. Atoms are extremely small. A hydrogen atom is about in diameter. According to some estimates, five trillion hydrogen atoms could fit on the head of a pin. Atomic radius refers to the average distance between the nucleus and the outermost electron. Models of atoms that include the proton, nucleus, and electrons typically show the electrons very close to the nucleus and revolving around it, similar to how the Earth orbits the sun. However, another model relates the Earth as the nucleus and its atmosphere as electrons, which is the basis of the term “electron cloud.” Another description is that electrons swarm around the nucleus. It should be noted that these atomic models are not to scale. A more accurate representation would be a nucleus with a diameter of about 2 cm in a stadium. The electrons would be in the bleachers. This model is similar to the not-to-scale solar system model. In reference to the periodic table, atomic radius increases as energy levels are added and decreases as more protons are added (because they pull the electrons closer to the nucleus). Essentially, atomic radius increases toward the left and toward the bottom of the periodic table (i.e., Francium (Fr) has the largest atomic radius while Helium (He) has the smallest). All matter consists of atoms. Atoms consist of a nucleus and electrons. The nucleus consists of protons and neutrons. The properties of these are measurable; they have mass and an electrical charge. The nucleus is positively charged due to the presence of protons. Electrons are negatively charged and orbit the nucleus. The nucleus has considerably more mass than the surrounding electrons. Atoms can bond together to make molecules. Atoms that have an equal number of protons and electrons are electrically neutral. If the number of protons and electrons in an atom is not equal, the atom has a positive or negative charge and is an ion.