Genetics, Biotechnology, Evolution & Natural Selection PDF
Document Details
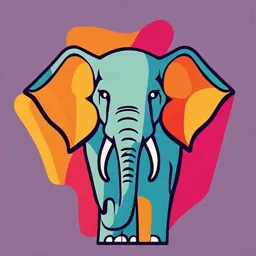
Uploaded by CushyPoisson947
Tags
Summary
This document outlines key concepts in genetics, biotechnology, evolution, and natural selection. Topics covered include genetics and heredity, DNA structure and replication, genetic disorders, advances in biotechnology, ethical considerations, and evidence for evolution including Darwin's theory of natural selection, genetics influence, and the role of the environment
Full Transcript
Genetics & Biotechnology, Evolution & Naturals Selection Human Reproductive System Functions........................................................................................................................2 Male reproductive system................
Genetics & Biotechnology, Evolution & Naturals Selection Human Reproductive System Functions........................................................................................................................2 Male reproductive system........................................................................................................................................... 2 Female reproductive system.......................................................................................................................................5 DNA, Genes & Heredity.......................................................................................................................................................8 DNA...................................................................................................................................................................................... 8 Genes & Inheritance...........................................................................................................................................11 Dominant vs. Recessive Traits................................................................................................................................. 12 Inheritance Patterns....................................................................................................................................................12 DNA & Chromosomes........................................................................................................................................................13 DNA....................................................................................................................................................................................13 Chromosomes................................................................................................................................................................ 14 Genetic Disorders Due to Chromosomal Abnormalities....................................................................... 15 Watson-Crick DNA Model............................................................................................................................................... 17 DNA Model............................................................................................................................................................17 DNA Replication........................................................................................................................................................... 18 Mutations & Their Effects......................................................................................................................................... 20 Advances in Biotechnology............................................................................................................................................. 21 Vaccines............................................................................................................................................................................21 Traditional Vaccines.....................................................................................................................................................22 mRNA Vaccines............................................................................................................................................................. 22 Genetic Engineering.................................................................................................................................................... 23 Stem Cell Therapy........................................................................................................................................................ 24 Ethical Considerations in Biotechnology.................................................................................................................... 26 Pros of Biotechnology.................................................................................................................................................26 Cons of Biotechnology................................................................................................................................................27 Ethical concerns............................................................................................................................................................ 28 Evidence for Evolution...................................................................................................................................................... 29 Fossil Evidence...............................................................................................................................................................29 Comparative Anatomy...................................................................................................................................................... 31 Fossil Record & Earth’s Age............................................................................................................................................. 33 Fossil Dating Methods................................................................................................................................................ 33 Earth's Age & Evolutionary Timeline.................................................................................................................... 34 Natural Selection & Population Changes.................................................................................................................... 35 Darwin’s Theory of Natural Selection.................................................................................................................. 35 Examples of Natural Selection.................................................................................................................................37 Role of Genes & Environment in Survival................................................................................................................... 38 Genetic Influence on Traits.......................................................................................................................................38 Environmental Influence........................................................................................................................................... 39 LW3: Genetics & Biotechnology Human Reproductive System Functions Male reproductive system Primary Functions 1. Sperm Production (Spermatogenesis): ○ The testes are responsible for producing sperm, a process known as spermatogenesis. This occurs in specialised structures within the testes called seminiferous tubules. ○ Spermatogenesis begins during puberty and continues throughout a man's life, producing millions of sperm cells daily. The process involves meiosis, ensuring that sperm cells carry half the genetic material needed for fertilisation. 2. Sperm Delivery for Fertilisation: ○ Once sperm is produced, it is transported through the male reproductive tract and ultimately delivered to the female reproductive system during sexual intercourse. The goal is to facilitate the meeting of the sperm with an ovum (egg) for fertilisation. Key Structures and Their Functions 1. Testes: ○ Structure: Located in the scrotum, the testes are oval-shaped organs that consist of seminiferous tubules and interstitial cells. ○ Functions: Seminiferous Tubules: Sites of spermatogenesis. Interstitial Cells (Leydig Cells): Produce testosterone, the primary male sex hormone, which regulates sperm production and secondary sexual characteristics such as facial hair growth and voice deepening. 2. Epididymis: ○ Structure: A tightly coiled tube located on top of each testicle. ○ Functions: Stores sperm produced in the testes. Allows sperm to mature and gain motility over approximately two weeks. 3. Vas Deferens (Ductus Deferens): ○ Structure: A muscular tube that connects the epididymis to the urethra. ○ Functions: Transports mature sperm during ejaculation through rhythmic contractions of its walls. Provides a pathway for sperm to reach the ejaculatory ducts. 4. Seminal Vesicles: ○ Structure: A pair of sac-like glands located behind the bladder. ○ Functions: Produce seminal fluid rich in fructose to nourish sperm. This fluid makes up about 60-70% of the total semen volume, providing energy and mobility to sperm cells. 5. Prostate Gland: ○ Structure: A walnut-sized gland located below the bladder and encircling the urethra. ○ Functions: Produces an alkaline fluid that neutralises the acidic environment of the female reproductive tract, aiding sperm survival. Adds additional fluids to the semen that enhance sperm mobility and longevity. 6. Bulbourethral Glands (Cowper's Glands): ○ Structure: Small pea-shaped glands located below the prostate gland. ○ Functions: Secrete a clear, lubricating fluid that prepares the urethra for sperm passage. The fluid helps to neutralise traces of acidic urine in the urethra. 7. Urethra: ○ Structure: A tube that runs through the penis, serving as a common passageway for urine and semen. ○ Functions: Facilitates the delivery of sperm outside the male body during ejaculation. Ensures that urine and semen never mix due to the action of a muscular sphincter. 8. Penis: ○ Structure: Composed of specialised erectile tissues (corpora cavernosa and corpus spongiosum) that enable erection. ○ Functions: Serves as the organ of copulation, delivering semen to the female reproductive system. Facilitates sperm transfer during ejaculation. Hormonal Regulation The male reproductive system is intricately controlled by hormones: 1. Testosterone: Produced by Leydig cells in the testes, it is crucial for sperm production, libido, and the development of secondary sexual characteristics. 2. Follicle-Stimulating Hormone (FSH): Stimulates spermatogenesis within the seminiferous tubules. 3. Luteinising Hormone (LH): Promotes testosterone production by Leydig cells. Semen Composition Semen is the fluid that contains sperm and is essential for reproduction: Sperm Cells: The active reproductive cells. Seminal Fluid: Provides nourishment and energy to sperm cells. Prostate Fluid: Enhances mobility and survival of sperm. Bulbourethral Fluid: Lubricates the urethra and neutralises acidity. Female reproductive system Primary Functions 1. Egg Production (Oogenesis): ○ The ovaries are responsible for producing ova (eggs) through a process called oogenesis. ○ This process begins before birth, where a finite number of immature eggs (oocytes) are formed. These mature and are released cyclically during a woman's reproductive years (ovulation). ○ Each egg carries half the genetic material required for the creation of a new individual. 2. Embryo Development and Pregnancy Support: ○ The system provides a nurturing environment for the fertilised egg to implant and grow into an embryo, and later a fetus. ○ It ensures the delivery of nutrients, oxygen, and the removal of waste products during development. Key Structures and these groupings identified patterns of behaviour regarding atomic structureTheir Functions 1. Ovaries: ○ Structure: Almond-shaped organs located on either side of the uterus. ○ Functions: Produce and release eggs (ovulation). Secrete female sex hormones, including estrogen and progesterone, which regulate the menstrual cycle and secondary sexual characteristics like breast development. 2. Fallopian Tubes (Oviducts): ○ Structure: Narrow, muscular tubes connecting the ovaries to the uterus. ○ Functions: Transport the ovulated egg from the ovary to the uterus. Serve as the site of fertilisation, where the egg meets the sperm. This typically occurs in the ampulla, the widened section of the fallopian tube. Facilitate the movement of the fertilised egg (zygote) toward the uterus using tiny hair-like structures (cilia) and muscular contractions. 3. Uterus (Womb): ○ Structure: A pear-shaped, hollow muscular organ located in the pelvic cavity. ○ Functions: Provides a site for the implantation of a fertilised egg. Supports embryo and fetal development by supplying nutrients and oxygen via the placenta. Contracts during childbirth to expel the baby and the placenta. 4. Cervix: ○ Structure: The lower, narrow part of the uterus that opens into the vagina. ○ Functions: Acts as a gateway between the uterus and vagina. Produces mucus that facilitates or restricts sperm entry, depending on the stage of the menstrual cycle. Dilates during childbirth to allow the passage of the baby. 5. Vagina: ○ Structure: A muscular, elastic canal extending from the cervix to the external genitalia. ○ Functions: Serves as the site for sperm deposition during sexual intercourse. Acts as the birth canal during childbirth. Provides an outlet for menstrual flow. Hormonal Regulation The female reproductive system is governed by a complex interplay of hormones: 1. Estrogen: Promotes the development of female secondary sexual characteristics and regulates the menstrual cycle. 2. Progesterone: Prepares the uterus for implantation of the fertilised egg and supports early pregnancy. 3. Follicle-Stimulating Hormone (FSH): Stimulates the growth and maturation of ovarian follicles. 4. Luteinising Hormone (LH): Triggers ovulation and the formation of the corpus luteum, which secretes progesterone. Cycle Overview: Menstrual and Reproductive The female reproductive system operates in cycles, typically lasting about 28 days: ○ Follicular Phase: The ovarian follicles mature, and estrogen levels rise. ○ Ovulation: A mature egg is released from the ovary, triggered by an LH surge. ○ Luteal Phase: The corpus luteum forms, secreting progesterone to prepare the uterus for a potential pregnancy. ○ Menstruation: If fertilisation does not occur, the uterine lining is shed, resulting in a menstrual period. DNA, Genes & Heredity DNA What is DNA? Definition: DNA is a complex molecule composed of two strands that form a double helix. It carries genetic information in the form of genes. Location: In eukaryotic cells, DNA is primarily located in the nucleus, organised into structures called chromosomes. Some DNA can also be found in the mitochondria, known as mitochondrial DNA. Structure of DNA 1. Double Helix: ○ DNA consists of two strands that twist around each other like a spiral staircase, held together by chemical bonds. 2. Building Blocks: DNA is made up of repeating units called nucleotides, which consist of: ○ Sugar Molecule: Deoxyribose. ○ Phosphate Group: Forms the backbone of the DNA strands. ○ Nitrogenous Bases: The “steps” of the staircase. These bases include: Adenine (A) Thymine (T) Guanine (G) Cytosine (C) ○ Base Pairing Rule: Adenine pairs with Thymine (A-T). Guanine pairs with Cytosine (G-C). 3. Chromosome Organisation: ○ DNA is tightly wound and packaged around proteins called histones to form chromosomes. This compact structure allows large amounts of DNA to fit inside the nucleus. Functions of DNA 1. Genetic Information Storage: ○ DNA contains genes, which are specific sequences of nucleotides that code for proteins and traits. Each gene is like a recipe for a particular protein that performs various functions in the body. 2. Replication: ○ DNA can replicate itself during cell division, ensuring that each new cell receives an identical copy of genetic material. 3. Protein Synthesis: ○ DNA provides instructions for building proteins via two processes: Transcription: DNA is transcribed into messenger RNA (mRNA). Translation: The mRNA is translated into proteins with the help of ribosomes and transfer RNA (tRNA). 4. Inheritance: ○ DNA carries hereditary information from parent to offspring, ensuring the continuation of genetic traits. Interesting Features Universal Code: The structure and function of DNA are remarkably consistent across all living organisms, emphasising its fundamental role in life. Mutations: Sometimes changes occur in the DNA sequence (mutations), which can lead to variations in traits or genetic disorders. These mutations are also a key driver of evolution. Genes & Inheritance Genes and Traits 1. What Are Genes? ○ Genes are located on chromosomes, which reside in the cell nucleus. Humans have 23 pairs of chromosomes (46 in total), with one set inherited from each parent. ○ Each gene contains instructions for producing proteins that contribute to physical and functional traits, such as eye color, hair texture, and even certain predispositions to diseases. 2. How Do Genes Determine Traits? ○ Traits are determined by alleles, which are different versions of a gene. Each individual inherits two alleles for a gene: one from each parent. Dominant vs. Recessive Traits 1. Dominant Traits: ○ A dominant allele is expressed if it is present, even if only one copy is inherited. ○ For example, the allele for brown eyes (B) is dominant. A person with BB (homozygous dominant) or Bb (heterozygous) alleles will have brown eyes. 2. Recessive Traits: ○ A recessive allele is expressed only when both alleles are recessive (homozygous recessive). ○ For example, the allele for blue eyes (b) is recessive. To have blue eyes, a person must inherit bb alleles. Inheritance Patterns 1. Mendelian Inheritance: ○ Based on Gregor Mendel’s work, inheritance follows predictable patterns for traits influenced by a single gene with two alleles: Punnett Square: A tool used to predict the probability of offspring inheriting specific alleles from their parents. 2. Example of Eye Color Inheritance: Let’s say both parents have Bb alleles (heterozygous for brown eyes): ○ Offspring Possibilities: 25% chance of inheriting BB (brown eyes). 50% chance of inheriting Bb (brown eyes). 25% chance of inheriting bb (blue eyes). 3. Other Examples: ○ Dominant Traits: Brown hair, dimples, widows' peak. ○ Recessive Traits: Blonde hair, attached earlobes, straight hair. Complexities Beyond Dominance Not all traits follow simple dominant-recessive patterns: 1. Codominance: Both alleles are fully expressed, as seen in blood type AB, where alleles A and B are codominant. 2. Incomplete Dominance: The resulting phenotype is a blend of both alleles, such as pink flowers from red and white flower alleles. 3. Polygenic Traits: Traits like height and skin color are influenced by multiple genes, making them more complex. DNA & Chromosomes DNA Structure of DNA and Chromosomes 1. DNA Packaging into Chromosomes: ○ In human cells, DNA is extremely long, measuring about 2 meters per cell if stretched out. To fit inside the tiny cell nucleus, DNA is tightly coiled and compacted into structures called chromosomes. ○ This compact structure is achieved through the wrapping of DNA around proteins called histones, forming a bead-like structure called a nucleosome. These nucleosomes further coil and fold to form the dense, rod-shaped chromosomes visible during cell division Humans have 46 chromosomes, organized into 23 pairs. Autosomes: 22 pairs are non-sex chromosomes, responsible for the majority of genetic traits. Sex Chromosomes: 1 pair determines biological sex—XX in females and XY in males. 2. Chromosome Composition: ○ Each chromosome contains a single, continuous DNA molecule, made up of thousands of genes. ○ The DNA sequence in chromosomes carries the instructions for building and maintaining the body through processes like protein synthesis. Inheritance and Chromosome Pairs 1. One Set from Each Parent: ○ In humans, 23 chromosomes are inherited from the mother (via the egg) and 23 from the father (via the sperm), creating a total of 46. ○ This arrangement ensures a combination of genetic material from both parents, contributing to genetic diversity. 2. Homologous Pairs: ○ The 23 pairs of chromosomes consist of homologous pairs, where one chromosome from each pair is maternal, and the other is paternal. ○ Homologous chromosomes contain the same genes at the same locations, though they may have different versions (alleles) of those genes. Chromosome Behavior During Cell Division 1. Mitosis: ○ During mitosis, chromosomes replicate and ensure that each daughter cell receives an identical set of 46 chromosomes, maintaining genetic consistency. 2. Meiosis: ○ In meiosis, which produces gametes (sperm and egg cells), the number of chromosomes is halved to 23. This ensures that when the egg and sperm combine, the resulting zygote will have the correct total of 46 chromosomes. Chromosomes Types of Chromosomes 1. Autosomes: ○ Definition: Autosomes are non-sex chromosomes that make up 22 pairs out of the total 23 pairs in humans. ○ Function: These chromosomes carry the genetic information responsible for determining general traits, such as eye color, hair type, height, and metabolic functions. They contain genes that are inherited in pairs, one from each parent, ensuring a mix of characteristics. ○ Example: The first 22 pairs of human chromosomes are autosomes, and they are numbered 1 through 22 based on their size and shape (1 being the largest, ○ L 22 the smallest). 2. Sex Chromosomes: ○ Definition: The 23rd pair of chromosomes determines biological sex and differs between males and females. ○ Types: XX: Female individuals inherit two X chromosomes, one from each parent. XY: Male individuals inherit one X chromosome from the mother and one Y chromosome from the father. ○ Functions: The X Chromosome: Contains genes essential for various biological functions and some traits unrelated to sex determination. The Y Chromosome: Contains genes crucial for male development, including the SRY gene, which triggers male sexual differentiation. Importance of Chromosome Types Autosomes: Provide the instructions for most physiological and structural traits that are essential for survival and reproduction. Sex Chromosomes: Determine biological sex, influencing sex-specific traits and the functioning of reproductive systems. Genetic Disorders Due to Chromosomal Abnormalities Down Syndrome (Trisomy 21) Cause: ○ Down Syndrome occurs due to an extra copy of chromosome 21 (trisomy 21). Instead of having two copies, individuals with Down Syndrome have three copies of this chromosome. ○ It is often caused by nondisjunction during meiosis, where chromosomes fail to separate properly. Symptoms and Characteristics: ○ Intellectual disability ranging from mild to moderate. ○ Distinct facial features, including a flat nasal bridge, upward-slanting eyes, and small ears. ○ Hypotonia (low muscle tone). ○ Increased risk of congenital heart defects and other health issues, such as hearing loss or thyroid disorders. Turner Syndrome (Monosomy X) Cause: ○ Turner Syndrome occurs in females due to the absence of one X chromosome. Instead of the typical XX configuration, affected individuals have only one X chromosome (45 chromosomes in total). ○ It may result from nondisjunction or the loss of a part of the X chromosome during early cell division. Symptoms and Characteristics: ○ Short stature and delayed or absent puberty. ○ Infertility due to underdeveloped ovaries. ○ Broad chest and widely spaced nipples. ○ Possible heart defects and kidney abnormalities. ○ Normal intelligence, though some may experience specific learning difficulties. Klinefelter Syndrome (XXY) Cause: ○ Klinefelter Syndrome occurs in males due to an extra X chromosome, leading to the XXY configuration. This results in a total of 47 chromosomes rather than the usual 46. ○ It typically arises from nondisjunction during either paternal or maternal gamete formation. Symptoms and Characteristics: ○ Reduced testosterone levels, leading to low muscle mass, less facial and body hair, and small testes. ○ Infertility due to impaired sperm production. ○ Taller stature with longer legs relative to the torso. ○ Possible learning difficulties or mild cognitive impairment. ○ Increased risk of certain health issues, such as osteoporosis or breast tissue development (gynecomastia). Significance of Understanding Chromosomal Abnormalities These disorders highlight the critical role chromosomes play in normal development. Early diagnosis and intervention, such as speech therapy, hormone treatments, or surgical management, can greatly improve the quality of life for individuals with these conditions. wWatson-Crick DNA Model DNA Model Structure of DNA 1. Double-Helix Structure: ○ DNA is composed of two long strands twisted into a spiral shape known as a double helix. ○ This structure resembles a twisted ladder, with the sugar-phosphate backbone forming the sides and nitrogenous bases forming the rungs. 2. Nucleotides: ○ DNA is made up of repeating units called nucleotides, each containing three components: Deoxyribose Sugar: A five-carbon sugar. Phosphate Group: Links sugar molecules to form the backbone. Nitrogenous Bases: The information-carrying part of DNA. 3. Four Nitrogenous Bases: ○ DNA uses four types of nitrogenous bases: Adenine (A) pairs with Thymine (T) through two hydrogen bonds. Cytosine (C) pairs with Guanine (G) through three hydrogen bonds. ○ This complementary base pairing ensures accurate replication and transcription of genetic information. 4. Anti-Parallel Strands: ○ The two strands of DNA run in opposite directions, known as antiparallel orientation. One strand runs in the 5' to 3' direction, while the other runs 3' to 5'. ○ This arrangement is critical for processes like DNA replication. 5. Backbone Composition: ○ The sides of the double helix consist of alternating deoxyribose sugars and phosphate groups, connected by strong covalent bonds, providing stability to the DNA molecule. 6. Base Pairing Rules: ○ The specific pairing of A with T and C with G maintains a uniform width for the DNA double helix and ensures genetic fidelity. ○ These base pairs are held together by hydrogen bonds, which are strong enough to stabilize the molecule but weak enough to allow strand separation for replication and transcription. Significance of the Watson-Crick Model 1. Understanding Genetic Information: ○ The model revealed how DNA stores and transmits genetic information through the sequence of its bases. ○ It also explained how the molecule could replicate itself during cell division with high accuracy. 2. Foundation for Modern Biology: ○ The discovery laid the groundwork for molecular biology, leading to breakthroughs in genetic research, biotechnology, and medicine. DNA Replication What is DNA Replication? DNA replication is the process by which a cell duplicates its DNA, creating two identical copies from the original molecule. It occurs during the S phase of the cell cycle, prior to mitosis (for somatic cells) and meiosis (for gamete production). This process ensures that every daughter cell receives an exact copy of the genetic material. Steps in DNA Replication 1. Initiation: ○ DNA replication begins at specific locations called origins of replication. In eukaryotes, there are multiple origins to facilitate the process across the long DNA molecules. ○ The enzyme helicase unwinds the DNA double helix by breaking the hydrogen bonds between the base pairs, creating a replication fork (a Y-shaped structure). ○ Single-strand binding proteins (SSBs) attach to the separated strands to prevent them from re-annealing. 2. Priming: ○ The enzyme primase synthesizes a short RNA primer, providing a starting point for DNA polymerase to begin synthesis. ○ These primers are complementary to the DNA template strand. 3. Elongation: ○ DNA polymerase adds complementary nucleotides to the template strands, synthesizing new DNA in the 5' to 3' direction. On the leading strand (synthesized continuously): DNA polymerase moves along the template strand smoothly as the replication fork progresses. On the lagging strand (synthesized in fragments): DNA polymerase works in short stretches, producing Okazaki fragments, as replication proceeds in the opposite direction of the fork's movement. ○ DNA ligase eventually joins these fragments to form a continuous strand. 4. Termination: ○ DNA replication ends when the entire molecule has been copied. ○ The RNA primers are replaced with DNA, and any gaps or nicks are sealed by DNA ligase. Accuracy in DNA Replication DNA replication is highly accurate due to the proofreading ability of DNA polymerase, which identifies and corrects mismatched base pairs. This ensures minimal errors (mutations), maintaining the integrity of the genetic information passed to daughter cells. Significance of DNA Replication 1. Genetic Continuity: ○ Ensures that each daughter cell receives an identical set of genetic instructions, maintaining species-specific traits. 2. Growth and Repair: ○ Facilitates cell division necessary for growth, tissue repair, and reproduction in multicellular organisms. 3. Evolutionary Importance: ○ Occasionally, errors (mutations) during replication introduce genetic variations, which contribute to evolution. Mutations & Their Effects What are Mutations? A mutation is an alteration in the nucleotide sequence of DNA, which can affect the structure or function of genes. While some mutations are harmless or even beneficial, others can disrupt normal biological processes and lead to diseases, including cancer. Types of Mutations 1. Point Mutations: ○ Definition: A point mutation occurs when a single nucleotide in the DNA sequence is replaced with another. ○ Subtypes: Silent Mutation: Does not change the resulting protein (no effect on function). Missense Mutation: Changes one amino acid in the protein, potentially altering its function. Nonsense Mutation: Creates a premature stop codon, leading to a truncated and usually nonfunctional protein. ○ Example: Sickle cell anemia is caused by a point mutation that alters the hemoglobin protein. 2. Deletion Mutations: ○ Definition: A deletion occurs when one or more nucleotides are removed from the DNA sequence. ○ Impact: Depending on the size and location of the deletion, it can disrupt the gene’s function or shift the reading frame (frameshift mutation), leading to a completely altered protein. ○ Example: Duchenne muscular dystrophy is often caused by deletions in the dystrophin gene. 3. Insertion Mutations: ○ Definition: An insertion involves the addition of one or more nucleotides into the DNA sequence. ○ Impact: Similar to deletions, insertions can cause a frameshift mutation, altering downstream amino acids. ○ Example: Huntington’s disease is associated with an insertion of repeated DNA sequences in the HTT gene. 4. Frameshift Mutations (Combined Effects): ○ These occur when deletions or insertions change the reading frame of the genetic code, drastically altering protein synthesis and function. ○ They can lead to severe genetic disorders or loss of gene function. Effects of Mutations 1. Cancer Development: ○ Mutations in genes that control cell growth (proto-oncogenes) or repair DNA (tumor suppressor genes) can lead to uncontrolled cell division and cancer. ○ For example, mutations in the TP53 gene are common in various cancers. 2. Genetic Disorders: ○ Mutations can lead to inherited conditions such as cystic fibrosis, Tay-Sachs disease, or chromosomal disorders (like Down Syndrome). 3. Beneficial Mutations: ○ Rarely, mutations can provide advantages, such as increased resistance to diseases. For example, the CCR5-Δ32 mutation confers resistance to HIV infection. Causes of Mutations Mutations can be triggered by: Spontaneous Errors: During DNA replication or repair. Environmental Factors: Exposure to radiation, chemicals, or viruses. Inherited Changes: Passed down from parents in genetic material. Advances in Biotechnology Vaccines Traditional Vaccines 1. How They Work: ○ Traditional vaccines introduce a weakened or inactivated form of a pathogen (virus or bacteria), or a specific part of it (like a protein or sugar), into the body. ○ These components, known as antigens, stimulate the immune system to recognize and attack the pathogen if encountered in the future. ○ By exposing the immune system to a harmless version of the pathogen, the body learns to respond more effectively during an actual infection. 2. Types of Traditional Vaccines: ○ Live Attenuated Vaccines: Contain a weakened form of the pathogen that cannot cause disease in healthy individuals. Examples: Measles, Mumps, and Rubella (MMR) vaccine, Yellow Fever vaccine. ○ Inactivated Vaccines: Contain pathogens that have been killed or inactivated so they cannot replicate. Examples: Polio (IPV) vaccine, Hepatitis A vaccine. ○ Subunit, Conjugate, or Toxoid Vaccines: Contain specific parts of the pathogen (e.g., proteins or toxins) rather than the entire organism. Examples: Whooping Cough (pertussis) vaccine, Hepatitis B vaccine. 3. Advantages: ○ Proven technology with long-standing effectiveness. ○ Provides long-lasting immunity with booster doses in some cases. 4. Disadvantages: ○ Live attenuated vaccines may not be suitable for immunocompromised individuals. ○ Production can be time-consuming for certain pathogens. mRNA Vaccines 1. How They Work: ○ mRNA vaccines use messenger RNA (mRNA) to instruct cells to produce a specific protein (antigen) associated with a pathogen. ○ For example, in COVID-19 vaccines, the mRNA encodes the spike protein of the SARS-CoV-2 virus. Once produced, this protein triggers an immune response, teaching the body to fight the actual virus. 2. Key Features of mRNA Technology: ○ Unlike traditional vaccines, mRNA vaccines do not use live or inactive pathogens, reducing the risk of infection. ○ The mRNA is encapsulated in lipid nanoparticles to protect it and deliver it into cells. 3. Examples of mRNA Vaccines: ○ Pfizer-BioNTech and Moderna COVID-19 vaccines. 4. Advantages: ○ Rapid Development: mRNA vaccines can be designed and produced quickly in response to emerging diseases. ○ Safety: They cannot cause disease as they do not use live pathogens. ○ Adaptability: mRNA technology can be updated easily to address new variants or pathogens. 5. Disadvantages: ○ Requires storage at low temperatures (e.g., -70°C for Pfizer-BioNTech). ○ Limited long-term data on newer mRNA technologies, though current studies support their safety and efficacy. DNA Fingerprinting Overview of DNA Fingerprinting 1. What is DNA Fingerprinting ? → DNA Fingerprinting refers to the process of analysing and utilising the genetic disparities between individuals to establish their relation to another individual for the purpose of paternity or maternity tests or in forensics. → It involves examining specific sections of the DNA that vary from each individual whilst showing some similarities between relatives. This short segment of the DNA polynucleotide chain is compared to another potential relative or suspect to determine their relationship in crime or to the person. 2. How Does It Work ? → This procedure is the method of identifying and isolating variable elements in the base-pair sequence of DNA, allowing scientists to recognise differences and similarities in genetic material. This process can includes the steps : Step 1 : DNA Extraction - Acquiring a sample of the cells in the form of skin, blood, saliva or hair to obtain and examine the genetic material. Step 2 : DNA Amplification - The polymerase chain reaction (PCR) is utilised to produce an abundant amount of a specific genetic code which can be used in the test. Step 3 : Restriction - This part of the process involves the use of restriction enzymes to cut the base-pair sequences at specific points to produce fragments of the strand that vary in length. Step 4 : Separation - The restricted parts of the strands are placed onto a gel subjecting it to an electric current (electrophoresis), separating the fragments by size : where the shorter the fragment, the more quickly it moves towards the anode. This creates a pattern of bands of DNA fragments. Step 5 : Visualisation - The strands are transferred to a nylon sheet and exposed to radiation to create an X-ray that can help visualise the patterns created by the DNA fragments Step 6 : Comparison - The strands are compared with another source where a more similar or matching pattern suggests a higher probability of relation. 3. Applications Genetic Engineering Overview of Genetic Engineering 1. What is Genetic Engineering? ○ Genetic engineering involves directly manipulating an organism's DNA to introduce, remove, or modify genetic material. ○ This can be achieved using various techniques, including molecular cloning, recombinant DNA technology, and advanced gene-editing tools like CRISPR. 2. Goals: ○ Improve traits such as disease resistance, increased yield, or tolerance to environmental stress. ○ Develop treatments for genetic disorders and diseases. Examples and Applications 1. GMO Crops: ○ Definition: Genetically Modified Organisms (GMOs) are plants or animals whose DNA has been altered to express specific traits. ○ Key Traits: Pest Resistance: For example, Bt corn contains a gene from the bacterium Bacillus thuringiensis that produces proteins toxic to certain pests, reducing the need for chemical pesticides. Drought Tolerance: Crops can be engineered to withstand water scarcity, ensuring stable yields in arid regions. Nutritional Enhancement: Golden rice is enriched with beta-carotene, which is converted into Vitamin A in the human body, addressing deficiencies in certain populations. ○ Impact: GMO crops improve agricultural sustainability, reduce environmental impact, and enhance food security. 2. CRISPR Technology: ○ Definition: CRISPR (Clustered Regularly Interspaced Short Palindromic Repeats) is a precise and powerful gene-editing tool that can target and modify specific DNA sequences. ○ Applications in Medicine: Disease Treatment: CRISPR is being explored to treat genetic disorders such as sickle cell anemia and cystic fibrosis by correcting mutations in patient DNA. Cancer Research: Scientists are investigating the use of CRISPR to engineer immune cells to better target and attack cancer cells. Infectious Diseases: CRISPR is being studied to eliminate viral DNA from infected cells, offering potential cures for chronic infections like HIV. ○ Advantages: CRISPR offers unprecedented precision, low cost, and versatility compared to earlier genetic engineering methods. Ethical Considerations Genetic engineering raises important ethical questions: 1. Safety Concerns: Potential long-term effects on ecosystems and human health (e.g., GMO consumption). 2. Access and Equity: Ensuring fair distribution of genetically engineered treatments and crops. 3. Genetic Modification of Humans: The possibility of editing human embryos sparks debate over ethical boundaries and unforeseen consequences. Stem Cell Therapy What is Stem Cell Therapy? Stem cells are unique cells with the ability to develop into various specialized cell types (e.g., muscle cells, nerve cells) and to self-renew, meaning they can divide and produce more stem cells. Stem cell therapy involves using these cells to repair or regenerate damaged tissues and organs, offering hope for treating conditions previously thought to be incurable. Types of Stem Cells Used 1. Embryonic Stem Cells (ESCs): ○ Derived from early-stage embryos, they have pluripotent ability, meaning they can become nearly any cell type in the body. ○ Controversial due to ethical considerations, they are less commonly used in clinical settings. 2. Adult Stem Cells (ASCs): ○ Found in specific tissues like bone marrow, blood, and fat, they have multipotent ability, meaning they can develop into a limited range of cells (e.g., blood cells or bone cells). ○ Commonly used for therapies like bone marrow transplants for leukemia. 3. Induced Pluripotent Stem Cells (iPSCs): ○ These are adult cells reprogrammed to behave like embryonic stem cells. ○ They are pluripotent and avoid the ethical issues associated with embryonic stem cells. Applications of Stem Cell Therapy 1. Regenerating Damaged Tissues: ○ In cases of spinal cord injuries, stem cells can potentially replace damaged nerve cells, promoting recovery of motor functions and sensation ○ Ongoing studies are exploring the ability of stem cells to treat conditions like stroke or traumatic brain injuries. 2. Chronic Diseases and Disorders: ○ Diabetes: Stem cells may help regenerate insulin-producing cells in the pancreas. ○ Heart Disease: Stem cells are being tested to repair damaged heart tissue after a heart attack. 3. Genetic Disorders and Immune Diseases: ○ Stem cells are already used in therapies like bone marrow transplants for leukemia or other blood-related diseases. ○ They hold potential for treating genetic disorders, such as sickle cell anemia or immune deficiencies. 4. Other Applications: ○ Osteoarthritis: Regenerating cartilage in damaged joints. ○ Eye Disorders: Repairing retinal damage to restore vision. Challenges and Risks 1. Immune Rejection: ○ In some cases, the body may reject transplanted stem cells if they are perceived as foreign. 2. Tumor Formation: ○ Pluripotent stem cells can potentially form tumors if not properly controlled during differentiation. 3. Ethical Concerns: ○ The use of embryonic stem cells raises moral and ethical questions, leading to debates about their research and application. 4. High Costs: ○ Stem cell therapies can be expensive, limiting their accessibility to patients. Ethical Considerations in Biotechnology Pros of Biotechnology Pros 1. Curing Genetic Diseases through Gene Therapy: ○ Definition: Gene therapy involves modifying or replacing faulty genes to treat or prevent genetic disorders. ○ Example: In conditions like cystic fibrosis, which is caused by mutations in the CFTR gene, gene therapy can introduce a functional copy of the gene, alleviating symptoms and improving patients' quality of life. ○ Impact: Offers potential cures for diseases previously considered untreatable. Reduces reliance on lifelong medications and invasive procedures. Opens the door for personalized medicine, where treatments are tailored to individual genetic profiles. 2. Improving Agricultural Yield with GMO Crops: ○ Definition: Genetically Modified Organisms (GMOs) involve altering crop DNA to enhance desirable traits such as pest resistance, drought tolerance, or nutritional content. ○ Examples: Bt Corn: Contains a bacterial gene that produces proteins toxic to certain pests, reducing pesticide use. Golden Rice: Engineered to be rich in beta-carotene, addressing Vitamin A deficiencies in many parts of the world. ○ Impact: Improves food security by increasing crop yields and reducing losses caused by pests or environmental stress. Supports sustainable farming practices by minimizing the need for chemical inputs like pesticides and fertilizers. Contributes to addressing global malnutrition by enhancing the nutritional value of crops. Cons of Biotechnology Cons 1. Ethical Concerns: ○ Gene Editing in Humans: Techniques like CRISPR could lead to the creation of "designer babies," raising moral questions about altering human traits for non-medical reasons. ○ Use of Embryonic Stem Cells: Some view the use of embryonic stem cells for research and therapy as unethical due to the destruction of embryos. 2. Environmental Risks: ○ Unintended Ecosystem Impact: The introduction of genetically modified organisms (GMOs) into the environment could disrupt ecosystems. For example, pest-resistant crops might harm non-target species such as beneficial insects. ○ Gene Flow: Cross-pollination between GMO crops and wild relatives may lead to the spread of modified traits, potentially creating invasive species. 3. Health and Safety Concerns: ○ Long-Term Effects of GMOs: While GMOs are rigorously tested, some critics argue that their long-term health impacts are not yet fully understood. ○ Allergenic Reactions: Genetically modified foods might introduce allergens, causing unexpected allergic reactions in some individuals. 4. Economic and Social Inequalities: ○ High Costs: Advanced biotechnologies like gene therapy or stem cell treatments are often expensive, making them inaccessible to many populations. ○ Dependence on Corporations: Farmers may become reliant on biotech companies for patented seeds, potentially increasing costs and limiting farming autonomy. 5. Biosecurity Risks: ○ Dual Use of Biotechnology: While biotechnology can benefit humanity, there is potential for misuse, such as creating bioweapons or conducting unethical experiments. 6. Reduced Genetic Diversity: ○ The widespread use of genetically modified crops could reduce biodiversity, making ecosystems and agriculture more vulnerable to diseases or environmental changes. Ethical concerns 1. Designer Babies What Are Designer Babies? 1. The term refers to embryos genetically modified to select or enhance specific traits, such as intelligence, physical appearance, or resistance to certain diseases. 2. This process typically involves techniques like CRISPR gene editing to modify the DNA of embryos before implantation. Ethical Concerns: 1. Equity and Accessibility: Only wealthy individuals or nations may afford this technology, increasing societal inequality and creating a genetic "underclass." 2. Eugenics Fears: Selecting or enhancing specific traits could revive problematic ideologies around genetic superiority and discrimination. 3. Unintended Consequences: Genetic modifications might lead to unforeseen health issues, not just for the individual but for their descendants. 4. Loss of Diversity: A focus on "ideal traits" could diminish genetic and societal diversity, which is vital for adaptation and resilience. 5. Moral Questions: Some believe it is ethically wrong to alter embryos, as it interferes with natural processes and raises concerns about "playing God." 2. Cloning What is Cloning? 1. Cloning involves creating genetically identical organisms, either through somatic cell nuclear transfer (as in Dolly the sheep) or other advanced techniques. 2. Types of Cloning: Reproductive Cloning: Produces a living organism. Therapeutic Cloning: Aims to create tissues or organs for medical use without creating a full organism. Ethical Concerns: 1. Identity and Individuality: Cloning could undermine the concept of individuality, raising psychological and societal challenges for the cloned individual. 2. Exploitation of Clones: Cloned organisms might be treated as "less than human," leading to exploitation or unethical treatment. 3. High Failure Rates and Suffering: Cloning experiments often result in numerous failed attempts, causing suffering to animals and potentially humans involved in the process. 4. Slippery Slope: Advancing cloning for therapeutic purposes might pave the way to widespread reproductive cloning, leading to societal and ethical dilemmas. 5. Religious and Philosophical Objections: Many question whether cloning aligns with spiritual and philosophical understandings of life, creation, and human purpose. LW4: Evolution & Natural Selection Evidence for Evolution Fossil Evidence Fossil evidence provides critical insights into the gradual changes in species over time, offering a window into evolutionary processes. The example of horse evolution is a well-documented case that highlights how adaptations to changing environments shape species. What is Fossil Evidence? Fossils are the preserved remains, impressions, or traces of ancient organisms found in sedimentary rock layers. By analyzing fossil records across different geological periods, scientists can trace how species evolved and adapted, often spanning millions of years. Horse Evolution: A Classic Example The evolutionary history of horses offers compelling evidence of gradual change, showcasing a transition from small, forest-dwelling animals to the large, grassland-dwelling horses we see today. Early Ancestors: 1. Eohippus (Hyracotherium): ○ Lived approximately 55 million years ago during the Eocene Epoch. ○ Small animal (about the size of a dog), with four toes on the front feet and three on the hind feet. ○ Adapted to life in dense forests, feeding on soft leaves. Intermediate Stages: 2. Mesohippus (Middle Horse): ○ Lived approximately 35-25 million years ago during the Oligocene Epoch. ○ Slightly larger, with three toes on both the front and hind legs, showing adaptation to drier and more open environments. ○ Teeth became better suited for grazing on tougher vegetation. 3. Merychippus: ○ Lived approximately 17 million years ago during the Miocene Epoch. ○ Significantly larger than predecessors, standing closer to the height of modern horses. ○ Developed a single hoof, though remnants of side toes were still visible. ○ Teeth adapted further for grazing on grass. Modern Horses: 4. Equus (Current Genus): ○ Emerged approximately 4-5 million years ago during the Pliocene Epoch. ○ Fully developed single hoof and taller stature for running across open grasslands. ○ Teeth optimized for grazing, reflecting the shift to a grassland diet. Significance of Fossil Evidence in Horse Evolution Gradual Adaptations: Fossil evidence demonstrates how changes in body size, limb structure, and teeth correspond to shifts from forest environments to open grasslands. Environmental Influences: The transition to open habitats favored traits like a single hoof for efficient running and teeth adapted to grass consumption. Clear Lineage: Fossils illustrate a direct lineage, providing one of the most complete records of evolutionary change. Comparative Anatomy Comparative anatomy, a cornerstone of evolutionary biology, examines similarities and differences in the structure of organisms. It provides evidence of shared ancestry and adaptive evolution through the concepts of homologous and analogous structures. Homologous Structures 1. Definition: ○ Homologous structures are anatomical features found in different species that share a similar structure and embryonic origin, even if their functions may differ. ○ These similarities arise because the species inherited these structures from a common ancestor. 2. Examples: ○ Human Hands, Bat Wings, and Whale Flippers: All have the same underlying bone structure (e.g., humerus, radius, ulna, and phalanges) but serve different functions: Human hands are adapted for grasping and manipulation. Bat wings are specialized for flight. Whale flippers aid in swimming. ○ Forelimbs of Vertebrates: Similar skeletal arrangements can also be observed in horses, frogs, and lizards. 3. Significance: ○ Homologous structures provide strong evidence for divergent evolution, where species with a common ancestor evolve to adapt to different environments. Analogous Structures 1. Definition: ○ Analogous structures are anatomical features that perform a similar function but have different structural origins and evolutionary pathways. ○ These arise due to convergent evolution, where unrelated species adapt to similar environmental pressures. 2. Examples: ○ Bird Wings and Insect Wings: Both are used for flight but are structurally different: Bird wings are supported by bones and covered with feathers. Insect wings are membranous structures with no internal bones. ○ Shark Fins and Dolphin Fins: Both serve as stabilizers and aid in swimming, but their structural composition and evolutionary origins differ. 3. Significance: ○ Analogous structures highlight how similar environmental challenges can lead to the evolution of comparable adaptations in unrelated species. Comparison Between Homologous and Analogous Structures Aspect Homologous Structures Analogous Structures Origin Common ancestor Different ancestors Structure Similar underlying anatomy Different structural organization Function Functions may vary Functions are typically similar Evolutionary Pathway Result of divergent evolution Result of convergent evolution Fossil Record & Earth’s Age Fossil Dating Methods Fossil dating methods are essential tools for determining the age of fossils and understanding Earth's history. Here’s an expanded look at Relative Dating and Radiometric Dating: 1. Relative Dating What It Is: ○ Relative dating determines the relative age of a fossil by examining its position within sedimentary rock layers (strata). ○ It does not provide an exact numerical age but instead places fossils in a sequence, identifying which are older or younger in comparison to others. Principles Used in Relative Dating: ○ Law of Superposition: In undisturbed layers of rock, the oldest layers are at the bottom, and the youngest are at the top. ○ Principle of Fossil Succession: Fossils appear in a consistent order within rock layers, allowing scientists to correlate rocks from different locations. ○ Index Fossils: Fossils of species that existed for a short time but were widely distributed are used as markers to date rock layers. Limitations: ○ It cannot provide a specific age for fossils. ○ It relies on the assumption that rock layers have remained undisturbed, which might not always be the case. 2. Radiometric Dating What It Is: ○ Radiometric dating provides an absolute age for rocks and fossils by measuring the decay of radioactive isotopes within the material. ○ This method calculates the time elapsed since the formation of the rock or fossil. How It Works: ○ Radioactive Decay: Radioactive isotopes (unstable atoms) decay at a constant rate into stable daughter isotopes. For example, Carbon-14 decays into Nitrogen-14, and Uranium-238 decays into Lead-206. ○ Half-Life: The half-life is the time it takes for half of the radioactive isotope in a sample to decay. For instance, Carbon-14 has a half-life of about 5,730 years, making it suitable for dating fossils up to 50,000 years old. Examples of Radiometric Dating Methods: ○ Carbon-14 Dating: Used for organic material like wood, bone, or shells. ○ Potassium-Argon Dating: Used for volcanic rock; effective for fossils older than 100,000 years. ○ Uranium-Lead Dating: Often used for dating ancient rocks and determining Earth's age. Advantages: ○ Provides an exact numerical age for fossils and rocks. ○ Useful for dating very old materials, sometimes billions of years old. Limitations: ○ Requires specific materials to date (e.g., organic material for Carbon-14). ○ Accuracy depends on assumptions about initial conditions and decay rates. Earth's Age & Evolutionary Timeline Earth's Age: Approximately 4.5 Billion Years 1. Formation of Earth: ○ Earth formed about 4.5 billion years ago from a massive cloud of gas and dust in the early solar system. ○ Gravity caused particles to coalesce, eventually forming the Earth as a molten, rocky planet. Over millions of years, the surface cooled to form a solid crust. 2. Evidence Supporting Earth's Age: ○ Radiometric Dating: Scientists use the decay of radioactive isotopes in rocks, such as uranium-lead dating of zircon crystals, to estimate Earth's age. ○ Oldest Rocks: The oldest terrestrial rocks, found in Canada (Acasta Gneiss) and Greenland, are about 4 billion years old. ○ Meteorites and Moon Rocks: Dating meteorites and lunar samples, which formed around the same time as Earth, also confirms the 4.5-billion-year age estimate. First Life Forms: Around 3.5 Billion Years Ago 1. Origin of Life: ○ Life began approximately 3.5 billion years ago, likely in Earth's early oceans, under conditions vastly different from today. ○ Early life forms were simple, single-celled organisms known as prokaryotes, which lacked a nucleus. 2. Evidence of Early Life: ○ Stromatolites: Fossilized microbial mats, found in ancient rocks, provide direct evidence of some of the earliest known life forms. ○ Biochemical Signatures: Chemical traces of life, such as specific isotopic patterns in ancient rocks, further support the timeline for life's origins. 3. Significance: ○ These primitive organisms began producing oxygen through photosynthesis around 2.5 billion years ago, leading to the Great Oxygenation Event, which transformed Earth's atmosphere and paved the way for more complex life forms. Natural Selection & Population Changes Darwin’s Theory of Natural Selection Charles Darwin’s theory of natural selection, introduced in his groundbreaking book On the Origin of Species (1859), is a cornerstone of evolutionary biology. It explains how species evolve over time through the survival and reproduction of organisms best adapted to their environment. Core Principles of Natural Selection 1. Variation: ○ Within a population, individuals show differences in traits (e.g., size, color, strength) due to genetic variation. ○ These differences arise through mutations, genetic recombination, and other factors. 2. Overproduction: ○ Most organisms produce more offspring than can survive to adulthood due to limited resources like food, water, and shelter. ○ This creates competition within the population. 3. Struggle for Existence/competition: ○ Individuals must compete for resources to survive. ○ Environmental pressures such as predators, climate, and disease further challenge survival. 4. Differential Survival and Reproduction: ○ Organisms with traits that provide an advantage in their environment (favorable traits) are more likely to survive and reproduce. ○ These traits increase an individual’s fitness—its ability to pass on its genes to the next generation. 5. Inheritance of Favorable Traits: ○ Favorable traits are passed onto offspring, becoming more common in the population over generations. ○ Over time, these adaptations can lead to the development of new species. Example: Peppered Moths Before the Industrial Revolution in England, light-colored peppered moths were more common as they blended in with tree bark. During the Industrial Revolution, pollution darkened tree bark with soot. Dark-colored moths now had a survival advantage, as they were better camouflaged from predators. Over time, the population shifted to predominantly dark-colored moths, illustrating natural selection in action. Significance of Darwin’s Theory Natural selection provided a scientific explanation for evolution, replacing earlier ideas like Lamarckism (inheritance of acquired traits). It emphasizes the role of environmental pressures in shaping the diversity of life. Examples of Natural Selection 1. Bacteria Evolving Antibiotic Resistance Mechanism: ○ Within a population of bacteria, there may be a few individuals with genetic variations that allow them to survive antibiotic exposure. ○ When antibiotics are used, these resistant bacteria survive while the rest die. ○ Over time, they reproduce, passing on their resistant genes, leading to an entire population that is resistant. Example: ○ Methicillin-Resistant Staphylococcus aureus (MRSA): This bacterium has become resistant to methicillin and other antibiotics due to overuse or misuse of these drugs. ○ Resistance mechanisms include changes in bacterial cell walls or enzymes that break down antibiotics. Impact: ○ Antibiotic resistance poses significant challenges for healthcare systems, requiring the development of new treatments and stricter antibiotic use protocols. 2. Insects Developing Pesticide Resistance Mechanism: ○ Within a population of insects, some individuals may have genetic mutations that allow them to survive exposure to pesticides. ○ These resistant insects survive and reproduce, passing on their resistance traits. ○ Repeated pesticide use accelerates the spread of resistance within the insect population. Example: ○ Mosquitoes and DDT: Initially effective, DDT became less useful as mosquitoes evolved resistance due to mutations in their nervous system or detoxification enzymes. ○ Colorado Potato Beetle: This agricultural pest has developed resistance to multiple insecticides, making it a challenge for crop management. Impact: ○ Resistance affects crop yields and increases the reliance on alternative pest control strategies, such as crop rotation or integrated pest management. Role of Genes & Environment in Survival Genetic Influence on Traits Genes play a fundamental role in determining physical traits and characteristics by encoding the proteins that influence an organism's development and functionality. How Genes Determine Traits 1. Genetic Basis of Traits: ○ Genes are sequences of DNA located on chromosomes, and they contain instructions for making proteins, which are the building blocks of cells and tissues. ○ These proteins influence the physical and functional aspects of an organism, such as fur color, beak shape, or eye color. 2. Inheritance of Traits: ○ Traits are passed from parents to offspring through genetic material. Each organism inherits one set of genes from its mother and one set from its father. ○ Variations in genes, called alleles, contribute to differences in traits among individuals. 3. Dominant and Recessive Genes: ○ Traits are determined by the interplay of dominant and recessive alleles. ○ Dominant alleles express their trait even if only one copy is present, while recessive alleles require two copies to be expressed. Examples of Genetic Influence 1. Fur Color in Animals: ○ Specific genes control pigmentation, leading to variations in fur color. ○ For example, in mice, the presence of a dominant allele might result in black fur, while two recessive alleles might produce white fur. 2. Beak Shape in Birds: ○ Genes determine the size and shape of a bird’s beak, which are adaptations to its feeding habits and environment. ○ Darwin’s finches are a classic example, where variations in beak shape evolved due to differences in diet, driven by genetic changes. 3. Eye Color in Humans: ○ Genes regulating the production and distribution of melanin in the iris contribute to eye color variations such as brown, blue, or green. Environmental and Genetic Interactions While genes provide the blueprint for traits, the environment can influence how these traits are expressed. For instance, the color of a Siamese cat’s fur is genetically determined but influenced by temperature during development. Environmental Influence Environmental factors play a crucial role in shaping the traits of populations over time, often driving evolution through natural selection. The peppered moths during the Industrial Revolution provide a compelling example of this process: Peppered Moth Example 1. Pre-Industrial Revolution: ○ Light-Colored Moths: Before industrialization, light-colored moths (Biston betularia) were more common as they blended in with the pale, lichen-covered tree trunks. ○ Dark-Colored Moths (Melanic Form): These were rare, as their darker coloration made them more visible to predators, such as birds. 2. Industrial Revolution Impact: ○ Pollution: Factories released large amounts of soot, darkening tree trunks and killing lichens. ○ Environmental Shift: This change created an advantage for dark-colored moths, which now blended better with the soot-covered trees, while light-colored moths became more conspicuous and preyed upon. 3. Natural Selection in Action: ○ Survival Advantage: The dark-colored moths had a survival advantage in polluted areas, allowing them to reproduce and pass on their traits. ○ Evolution Over Generations: Over time, the population shifted, with dark-colored moths becoming more prevalent. When environmental pollution was later reduced, the trend reversed, favoring light-colored moths again. Broader Implications The peppered moth example illustrates how environmental pressures can favor certain traits, leading to shifts in population characteristics. This principle is at the heart of natural selection and explains the evolution of traits in response to changing environments, such as beak adaptations in Darwin’s finches or fur color changes in Arctic animals.