Population Ecology PDF
Document Details
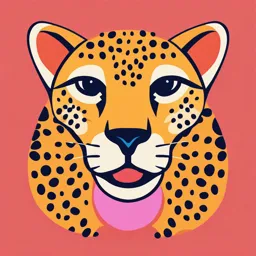
Uploaded by UnquestionableLyre
UPSI
Tags
Summary
This document summarises population ecology, outlining what population ecology is, patterns of distribution, population growth, population regulation, life history, and techniques for estimating population size. The document presents a series of slides containing different topics related to population ecology.
Full Transcript
TOPIC 3 POPULATION ECOLOGY 3.1 What is population ecology? 3.2 Pattern of distribution and dispersion 3.3 Population growth 3.4 Population regulation 3.5 Life history 3.6 Population size estimation 3.1 Population Ecology Population = the total number of individuals...
TOPIC 3 POPULATION ECOLOGY 3.1 What is population ecology? 3.2 Pattern of distribution and dispersion 3.3 Population growth 3.4 Population regulation 3.5 Life history 3.6 Population size estimation 3.1 Population Ecology Population = the total number of individuals of a given biological species found in one place at one time Populations can be described by: Size: How many total individuals there are? Density: How many individuals per unit of area? Dispersion: How are individuals in a population arranged spatially relative to another? Do they occur in clumps or are they evenly spread apart? Occupancy: Does a species or member of a population occur in a given habitat, or is it absent? Population distribution: Where does a population occur in space? Geographic range: What are the furthest geographic limits of where a species occurs? Population Ecology Population ecology Studies the distribution and abundance of organisms Examines how and why populations change over time Aims to understand the spatial and temporal patterns in the abundance and distribution of organisms and of the mechanisms that produce those patterns The science of population dynamics in space and time 3.2 Pattern of distribution and dispersion Dispersion patterns Also known as distribution patterns Summarize the spatial relationship between members of a population within a habitat at a particular point in time Clumped Uniform Random Can occur in plants and is Occurs with dandelion and Occurs in plants that drop thought to result from other plants that have wind- their seeds straight to the competition for below- dispersed seeds that ground, such as oak trees, or ground resources such as germinate wherever they animals that live in groups water, or secretion of happen to fall in a favorable (schools of fish or herds of substances inhibiting the environment. elephants) growth of nearby individuals. In animals like penguins that nest in large colonies, uniform dispersion can occur due to territorial behavior. 3.3 Population Growth Four ways that a population can change in size: Birth Death Change in population abundance Immigration Emigration Arrival of individuals Departure of from outside the individuals from population the population Growth rate = (Birth rate + Immigration rate) - (Death rate + Emigration rate) Age Structure Age structure = The relative numbers of organisms of each age within a population Age determination - annual growth rings - scales in fishes - horns in sheep - condition of dentition Age Structure Age structure affects population size Wide base → many young - High reproduction - Rapid population growth Even age distribution - Births = deaths - Stable population Population Growth: Exponential Growth Exponential growth occurs when a population's growth rate remains constant A population increases by a fixed percent A fixed percent of a large number produces a large increase Population Growth: Exponential Growth Exponential growth cannot be sustained indefinitely It occurs in nature for small populations with ideal conditions Rarely lasts for long Population Growth: Logistic/Sigmoid Growth Logistic growth occurs when density- dependent limiting factors cause population growth to gradually slow before reaching a maximum level at which growth will level off and become stable. Limiting factor Physical, chemical, and biological characteristics that restrain population growth Example: Water, space, food, predators, and disease Slow and stop exponential growth → Stabilizes population size Carrying capacity The maximum population size of a species that its environment can sustain Population Growth: Logistic/Sigmoid Growth 3.4 Population Regulation Population regulation refers to the ecological processes (biotic and abiotic factors) by which the growth of populations is limited due to the effects on birth and death rates. The ecological factors that limit population growth are known as limiting factors. There are two different kinds of limiting factors: Density-dependent limiting factor Density-independent limiting factor In addition, there are two kinds of population regulation: Top-down regulation Bottom-up regulation Density-dependent limiting factor in population regulation Density-dependent limiting factors impact a population’s per capita rate of growth based on the population’s density. These factors will generally cause the growth rate to drop as the population gets larger. Density-dependent limiting factors usually cause populations to reach a maximum level (at the population’s carrying capacity). At this point, the population size will level off and usually, but not always, become stable. This is known as logistic/sigmoid growth. When a population's growth rate remains constant, no matter its size, it will continue to grow larger at an exponential rate. This is known as exponentialgrowth. Density-dependent limiting factor Most density-dependent limiting factors are biotic. Examples: Intra- and interspecific competition Increased spreading of disease Parasitism In prey species, higher population densities may also result in higher predation rates. Individuals from a population that has reached carrying capacity may also wander out in search of new habitat that is not yet at The logistic/sigmoid growth model capacity. Density-dependent limiting factor Example: Saltwater crocodile (Crocodylus porosus) By the early 1970s, populations in Australia’s Northern Territory were nearing extinction. Following protective efforts over decades, the population recovered to the point that most rivers are believed to have reached carrying capacity. The density-dependent limiting factors include competition (e.g., finite prey availability and territoriality) and habitat limitations (e.g., breeding habitat and climatic restrictions) prevent the crocodile population from continued expansion. Density-dependent limiting factor Population cycles Populations experiencing density- dependent limiting factors often experience instability at carrying capacity, even without the effects of density-independent limiting factors. These populations may experience cycles of growth followed by a reduction in size in oscillating patterns called cyclical oscillations. Under specific circumstances, usually involving multiple species, these oscillations are driven by density-dependent limiting factors such as predation and resource abundance. Density-independent limiting factor in population regulation Density-independent factors impact the per capita population growth rate regardless of the population’s density. Since these factors do not depend upon the population’s size, their impact does not amount to the “correction” that density-dependent factors bring to a population. In other words, density-independent factors can be potentially catastrophic to smaller populations, particularly populations of a species with a limited geographic range. Density-independent factors can be abiotic, and perhaps the best example of a density-independent factor would be a natural disaster, such as a forest fire. A natural disaster may kill a significant fraction of the population living in the area, regardless of how large that population was, to begin with. Density-dependent limiting factor Example, black bear (Ursus americanus) populations are known to be affected by wildfires by way of decreased cub survival. If the population is limited to only a small area, a single natural disaster could even push a species to extinction. Top-down population regulation Top-down population regulation refers to situations where species at higher trophic levels (e.g., apex predators at the top of the food chain) control the populations of species at lower trophic levels (e.g., prey). Due to this, it is also called "predator-controlled" regulation. Typically, the population size and density of the apex predator at the top of the food chain is much lower than that of its prey, which is usually quite abundant. For example, mountain lions (Puma concolor) may control mule deer (Odocoileus hemionus) populations, but the mule deer may control the populations of certain plant species. Bottom-up population regulation Bottom-up population regulation is dependent on the resources of an ecosystem. Since all of the higher trophic levels are dependent on the continued presence of those below them, when those resources at the lower level are diminished or absent, all trophic levels a re a ffected. For example, if vegetation experiences a mass die-off, this may result in a decline in the mule deer population due to starvation. This, in turn, may also result in a reduction in the mountain lion population due to a lack of prey. Population regulation in humans Fifty years ago, in 1972, the human population consisted of around 3.9 billion people. Toda y that number has grown to over7.9 billion. Thus, the human population has more than doubled, growing more in the last half-century than in the entirety of human existence (at least 200,000 years). This exponential growth is largely due to better technology, food availability, medicine, and more, which have allowed humans to artificially increase their carrying capacity. However, this exponential cannot persist indefinitely, as the methods used to increase our carrying capacity are being outpaced by many density- dependent limiting factors. For humans, these limiting factors include widespread resource depletion (food, water, gas), climate change, increased spread of disease, and pollution. Population regulation in humans Indeed, the consequences of these density- dependent factors have always been present, but their impact will continue to be amplified as the population further exceeds the natural carrying capacity. Density-independent factors also affect human populations, with some notable recent examples including earthquakes, hurricanes, and tsunamis that cause large-scale damage to infrastructure and high mortality. To counteract this growth and mitigate consequences, artificial methods of human population regulation have been proposed, including increased access to contraception, family planning, and increased education. Human population growth since 1800, including future high and low projections. 3.5 Life History Strategies An individual organism’s life history is the events that characterize its life cycle, such as birth, growth, maturation, reproduction, and death. Looking at these characteristics can tell us important information about how that organism survives and reproduces. Life history theory was born from the idea that natural selection and evolutionary factors (i.e., competition for resources) influence the life history of an organism. Life history theory seeks to understand how adaptations that organisms have or develop increase their fitness, otherwise known as their survival and reproduction. The definition of life history strategy is how an organism decides to invest its energy and resources. An organism has trade-offs between its individual survival and reproduction (the survival of the next generation). These trade-offs, which are influenced by the abiotic and biotic components of its environment, lead the organism to develop a life history strategy. Life History Traits Ecologists measure these life history strategies by looking at the life history traits of groups of organisms. Life history traits are influenced by an organism’s interactions with its environment. Life history traits include: Size of orga nisms Growth rate of orga nisms Age at first reproduction Length of time when reproduction is possible (number of reproductive events) Number of offspring produced at each reproductive event Avera ge length of lifespan Life History Traits Horseshoe crabs are like living fossils because they have gone almost unchanged for about 400 million years, and their life-history traits have been well-tracked. Horseshoe crabs take almost ten years to reach sexual maturity and when they do they can reproduce for a number of years, living up to 20 years old! Every year a female can lay up to 88,000 eggs, which are independent and receive no care from the parents. Many eggs do not make it because of predators (birds, turtles, etc.) that find the eggs an important food source, so it's important that the female horseshoe crabs lay so many. Life Table Life history traits are the demographic events that scientists use to create life tables Life tables Look at particular cohorts or groups of organisms born in the same time frame Include information such as the age class (age of organisms at a certain point) or stages in their life cycle Information delivered in a life table Fecundity Definition: The number of females each female organism produces at each age class or life stage Fecundity represents the reproductive success of the organism Mortality Definition: How many individuals have died By looking at mortality, scientists know the number of survivors from the cohort at each life stage, also known by the term survivorship (Table 1) Life Table Table 1. A hypothetical life table for an insect showing the life stages, number surviving at each stage, and mortality rates. Life History Examples: Using Survivorship Curves Survivorship curves Are the graphic representation of the data that a life table helps track Show the number of individual organisms surviving at each stage in the organism's life cycle There are three types of survivorship curves that allow scientists to observe three different life history strategies: Type I Type II Type III Type I. Survivorship curves show a low mortality rate earlier in life and tend to decline rapidly when organisms reach an older age. This curve represents organisms that have an overall low fecundity (offspring produced) but parents give their offspring a high amount of care. Humans, whales, and large mammals display this survivorship curve. Type II. Survivorship curves are linear, meaning that an organism faces a similar chance of survival (or mortality) at any point in their lives. Organisms that often show this survivorship type include birds, rodents, and reptiles. Type III. Survivorship curves show organisms with high mortality rates at early life stages, but have a higher chance of survival once they make it past the early life stages. These organisms usually have many offspring (high fecundity) and provide little parental care. Examples of organisms that display type 3 survivorships include annual plants and insects. Life History Strategies in Ecology Survivorship curves typically show life history strategies in ecology and allow ecologists to see trends that may characterize different types of life history strategies graphically. By studying how different organisms use their energy between reproduction and individual survival, ecologists have identified two life history strategies that organisms may use. Life history strategies: r-strategists k-strategists R-strategists versus k-strategists R-strategists Tend to be organisms that reproduce lots of offspring, be smaller, and focus less on maternal care. They are often also semelparous, which means that they reproduce only once throughout their lives. K-strategists Organisms that will invest more in parental care and are slower to mature to their reproductive age. They often have more than one reproductive event throughout their lifetimes, making them iteroparous. Comparison of life history traits between r-strategists and k-strategists Examples of an r-strategist (grasshopper) and k-strategists (whale). 3.6 Population Size Population size (abundance, N) = The total number of organisms in a population How to determine population size? 1. Complete census 2. Population size 3. Population index estimation (sampling Counting each individual method) Use of data (other than present within the number of individuals) to population Involves mathematical represent actual Can be difficult, if not and statistical models abundance impossible Examples: Example of data: − Capture-recapture Frequency of animal tracks − Camera trapping or scat − Sampling: Quadrat and Can be biased and less transect accurate Capture-Recapture For mobile animals Also allow the estimate of birth rate and death rate Petersen method Involves only two sampling events Time interval between the two events is short Capture of animals Mark Release Recapture Total population size (N) = Total captured (1st sampling) x Total recaptured (2nd sampling) Total marked recaptured (2nd sampling) Capture-Recapture The validity of this technique depends on a number of assumptions: 1. The marking technique has no effect on the mortality of individuals 2. The marking technique does not affect the probability of being recapture 3. There is no immigration or emigration of marked or unmarked individuals in the interval t1 to t2 4. There is no mortality or reproduction in the interval t1 to t2 Capture-Recapture Calculation example: 1. 100 animals were captured and marked 2. When recaptured, the following data obtained Number of marked animals = 25 Number of unmarked animals = 50 Total population size = 100 x 75 25 = 300 animals Camera Trapping Camera trapping is used to estimate animal density Especially useful for studying rare and nocturnal animals Ab Razak, M. H. S., Hambali, K., Amaludin, N. A., Rak, A. E., & Malek, N. H. A. (2019, July). Density of Clouded Leopard (Neofelis nebulosa) in Gunung Basor-Stong Utara Forest Reserve, Kelantan, Malaysia. In IOP Conference Series: Earth and Environmental Science (Vol. 269, No. 1, p. 012001). IOP Publishing. Sampling Sampling is a technique used to find a small proportion of a population and use this to small section in order to draw conclusions regarding the rest of the population. Quadrat Transect Quadrat Sampling Quadrat Sampling Count all the individuals on several quadrats of known size and then extrapolate the average count to the whole area. Estimates require: The area of each quadrat must be known The population of each quadrat examined must be determined accurately The quadrats must be representative of the whole area i.e. achieved by random sampling Quadrat Sampling Steps in quadrat sampling Select quadrat shape Select quadrat size Determine number of quadrats Decide placement of quadrats Quantify organisms in quadrats Detailed steps: https://online.anyflip.com/kykv/nyjy/mobile/ Transect Sampling Transect sampling Involves use of single line which stretched over the area to be studied Used to sample changes along an environmental gradient – Example: geological, climatic or altitudinal gradients The length of a transect depends on the gradient under investigation – Rocky shore transects may only stretch 1–200 meters – Montane transects may exceed several kilometers Transect Sampling Types of transect 1. Intercept line transect 2. Point intercept Transect Sampling Types of transect 3. Belt transect 4. Ladder transect Population Index Involves the use of data that are correlated with actual abundance An index should be validated by checking its correlation with rigorous estimates of population size Examples of data: - Number of fecal pellets - Vocalization frequency - Pelt records - Catch per unit fishing effort Fecal pellet Pelt record - Number of artifacts Catch per unit Artifact fishing effort Population Density Population density = The number of individuals per unit area It is a measure of relative abundance Can be converted to a rough estimate population size through simple multiplication o 1 hectare of good habitat = 1.4 pairs of birds Population density o Size of habitat = 2000 hectares o Total number of bird pairs (N) = 1.4 pairs x 2000 hectares = 2800 pairs Population size Population Density Generally, larger organisms have lower population densities because they need more resources High densities make it easier to find mates, but increase competition and vulnerability to predation Low densities make it harder to find mates, but individuals enjoy plentiful resources and space Reduced resources can lead to overcrowding, disease, predation, parasitism, and extinction