Subsoil Exploration (Site Investigation) PDF
Document Details
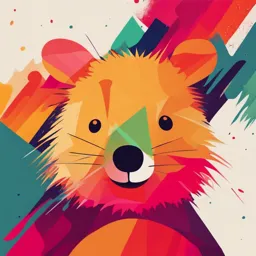
Uploaded by LucrativeDarmstadtium
University of Anbar
2023
Dr. Ahmed HAZIM Abdulkareem Dr. Maher Zuhair Al- Rawi
Tags
Summary
This document is a chapter on subsoil exploration for site investigation. It describes the methods, purpose, and procedures for subsurface exploration, including preliminary information gathering, reconnaissance, types of samplers, and coring of rocks. The chapter covers various aspects of site investigation and soil exploration.
Full Transcript
Chapter Two Site Investigation University of Anbar Engineering College Civil Engineering Department Chapter tWO SUBSOIL EXPLORATION (SITE INVESTIGATION) Lecture Dr. Ahmed HAZIM Abdulkareem...
Chapter Two Site Investigation University of Anbar Engineering College Civil Engineering Department Chapter tWO SUBSOIL EXPLORATION (SITE INVESTIGATION) Lecture Dr. Ahmed HAZIM Abdulkareem Dr. Maher Zuhair Al- Rawi 2023 - 2024 1 Chapter Two Site Investigation 2.1 Introduction To design a foundation that will support adequate structural load, one must understand the natural of the soils that will support the foundation. The process of determining the layers of natural soil deposits that will underlie a proposed structure and their physical properties is generally referred to as subsurface exploration. We will divide these techniques into three categories: 1- Site investigation- includes methods of defining the soil profile and other relevant data and recovering soil samples. 2- Laboratory testing – includes testing the soil samples in order to determine relevant engineering properties. 3- In-site testing – includes methods of testing the soil-in-place, thus avoiding the difficulties associated with recovering samples. 2.2 Purpose of Subsurface Exploration The purpose of subsurface exploration is to obtain information that will aid the geotechnical engineer in following: 1. Selecting the type and depth of foundation suitable for a given structure. 2. Evaluating the load-bearing capacity of the foundation. 3. Estimating the probable settlement of a structure. 4. Determining the nature of soil at the site and its stratification. 5. Determining potential foundation problems (for example, expansive soil, collapsible soil, sanitary landfill, etc…). 6. Determining the location of the water table. 7. Determining the depth and nature of bedrock, if and when encountered. 8. Performing some in situ field tests, such as permeability tests, van shear test, and standard penetration test. 9. Predicting the lateral earth pressure for structures such as retaining walls, sheet pile bulkheads, and braced cuts. 10. Establishing construction methods for changing subsoil conditions. 2 Chapter Two Site Investigation 2.3 Subsurface Exploration Program Subsurface exploration comprises several steps, including the collection of preliminary information, reconnaissance, and site investigation. 2.3.1 Collection of Preliminary Information This step involves obtaining information regarding the type of structure to be built and its general use. For the construction of buildings, the approximate column loads and their spacing and the local building-code and basement requirements should be known. The construction of bridges requires determining the lengths of their spans and the loading on piers and abutments. A general idea of the topography and the type of soil to be encountered near and around the proposed site can be obtained from the following sources: 1. Geological Survey maps. 2. State government geological survey maps. 3. Agriculture’s Soil Conservation Service county soil reports. 4. Agronomy maps published by the agriculture departments of various states. 5. Hydrological information published, including records of stream flow, information on high flood levels, tidal records, and so on. 6. Highway department soil manuals published. The information collected from these sources can be extremely helpful in planning a site investigation. In some cases, substantial savings may be realized by anticipating problems that may be encountered later in the exploration program. 2.3.2 Reconnaissance The engineer should always make a visual inspection (field trip) of the site to obtain information about: 1. The general topography of the site, the possible existence of drainage ditches, and other materials present at the site. 2. Evidence of creep of slopes and deep, wide shrinkage cracks at regularly spaced intervals may be indicative of expansive soil. 3 Chapter Two Site Investigation 3. Soil stratification from deep cuts, such as those made for the construction of nearby highways and railroads. 4. The type of vegetation at the site, which may indicate the nature of the soil. 5. Groundwater levels, which can be determined by checking nearby wells. 6. The type of construction nearby and the existence of any cracks in walls (indication for settlement) or other problems. 7. The nature of the stratification and physical properties of the soil nearby also can be obtained from any available soil-exploration reports on existing structures. 8. High-water marks on nearby buildings and bridge abutments. The nature of the stratification and physical properties of the soil nearby also can be obtained from any available soil-exploration reports on existing structures. 2.3.3 Site Investigation: This phase consists of: 1. Planning (adopting steps for site investigation, and future vision for the site) 2. Making test boreholes. 3. Collecting soil samples at desired intervals for visual observation and laboratory tests. Determining the number of boring: There is no hard-and-fast rule exists for determining the number of borings are to be advanced. For most buildings, at least one boring at each corner and one at the center should provide a start. Spacing can be increased or decreased, depending on the condition of the subsoil. If various soil strata are more or less uniform and predictable, fewer boreholes are needed than in nonhomogeneous soil strata. The following Table 2.1 gives some guidelines for borehole spacing between for different types of structures: 4 Chapter Two Site Investigation Table 2.1 Approximate Spacing of Boreholes Type of project Spacing (m) Multistory building 10–30 One-story industrial plants 20–60 Highways 250–500 Residential subdivision 250–500 Dams and dikes 40–80 Determining the depth of boring: The approximate required minimum depth of the borings should be predetermined. The estimated depths can be changed during the drilling operation, depending on the subsoil encountered (e.g., Rock). To determine the approximate required minimum depth of boring, engineers may use the rules established by the American Society of Civil Engineers (ASCE 1972): 1. Determine the net increase in effective stress (Δσ′) under a foundation with depth as shown in Fig. 2.1. 2. Estimate the variation of the vertical effective stress (σo′) with depth. 3. Determine the depth (D = D1) at which the effective stress increase (Δ𝛔′) is equal to (𝟏/𝟏𝟎) q (q = estimated net stress on the foundation). 4. Determine the depth (D = D2) at which (Δ𝛔′/𝛔𝐨′ ) =𝟎.𝟎𝟓. 5. Determine the depth (D = D3) which is the distance from the lower face of the foundation to bedrock (if encountered). 6. Choose the smaller of the three depths, (D1, D2, and D3), just determined is the approximate required minimum depth of boring. Fig. 2.1 Foundation with depth 5 Chapter Two Site Investigation After determining the value of (D) as explained above the final depth of boring (from the ground surface to the calculated depth) is: Dboring=Df + D Because the Drilling will starts from the ground surface. If the preceding rules are used, the depths of boring for a building with a width of 30 m (100 ft) will be approximately the following, according to Sowers and Sowers (1970): To determine the boring depth for hospitals and office buildings, Sowers and Sowers (1970) also used the following rules. (For light steel or narrow concrete buildings) (2.1) and (For heavy steel or wide concrete buildings) (2.2) where Db= depth of boring S = number of stories Notes: When deep excavations are anticipated, the depth of boring should be at least 1.5 times the depth of excavation. Sometimes, subsoil conditions require that the foundation load be transmitted to bedrock. The minimum depth of core boring into the bedrock is about 3 m (10 ft). If the bedrock is irregular or weathered, the core borings may have to be deeper. 6 Chapter Two Site Investigation The engineer should also take into account the ultimate cost of the structure when making decisions regarding the extent of field exploration. The exploration cost generally should be 0.1 to 0.5% of the cost of the structure. 2.4 Exploratory Borings in the Field Auger boring - is the simplest method of making exploratory boreholes. Fig. 2.2 shows two types of hand auger: the posthole auger and the helical auger. Hand augers cannot be used for advancing holes to depths exceeding 3 to 5 m (10 to 16 ft). However, they can be used for soil exploration work on some highways and small structures. Fig. 2.2 Hand tools: (a) posthole auger; (b) helical auger Portable power- driven helical augers (76 mm to 305 mm in diameter) are available for making deeper boreholes. The soil samples obtained from such borings are highly disturbed. In some non-cohesive soils or soils having low cohesion, the walls of the boreholes will not stand unsupported. In such circumstances, a metal pipe is used as a casing to prevent the soil from caving in. Continuous –flight augers (when power is available), are probably the most common method used for advancing a borehole. The power for drilling is delivered by truck- or tractor-mounted drilling rigs. Boreholes up to about 60 to 70 m can easily be made by this method. Continuous-flight augers are available in sections of 7 Chapter Two Site Investigation about 1 to 2 m with either a solid or hollow stem. Some of the commonly used solid- stem augers have outside diameters of 66.68 mm d, 82.55 mm, 101.6 mm, and 114.3 mm. Common commercially available hollow-stem augers have dimensions of 63.5 mm ID and 158.75 mm OD, 69.85 mm ID and 177.8 OD, 76.2 mm ID and 203.2 OD, and 82.55 mm ID and 228.6 mm OD. The tip of the auger is attached to a cutter head (Fig. 2.3). During the drilling operation (Fig. 2.4), section after section of auger can be added and the hole extended downward. The flights of the augers bring the loose soil from the bottom of the hole to the surface. The driller can detect changes in the type of soil by noting changes in the speed and sound of drilling. When solid-stem augers are used, the auger must be withdrawn at regular intervals to obtain soil samples and also to conduct other operations such as standard penetration tests. Hollow-stem augers have a distinct advantage over solid-stem augers in that they do not have to be removed frequently for sampling or other tests. As shown schematically in Fig. 2.5, the outside of the hollow-stem auger acts as a casing. The hollow-stem auger system includes the following components: Outer component: (a) hollow auger sections, (b) hollow auger cap, and (c) drive cap Inner component: (a) pilot assembly, (b) center rod column, and (c) rod-to-cap adapter The auger head contains replaceable carbide teeth. During drilling, if soil samples are to be collected at a certain depth, the pilot assembly and the center rod are removed. The soil sampler is then inserted through the hollow stem of the auger column. Fig. 2.3 Carbide-tipped cutting head on auger flight (Courtesy of Braja M. Das, Henderson, Nevada) 8 Chapter Two Site Investigation Fig. 2.4 Drilling wit continuous-flight augers \ Fig 2.5 Hollow-stem auger components (After ASTM, 2001) (Based on ASTM D4700-91: Standard Guide for Soil Sampling from the Vadose Zone.) 9 Chapter Two Site Investigation Wash boring - is another method of advancing boreholes. In this method, a casing about 2 to 3 m long is driven into the ground. The soil inside the casing is then removed by means of a chopping bit attached to a drilling rod. Water is forced through the drilling rod and exits at a very high velocity through the holes at the bottom of the chopping bit (Fig. 2.6). The water and the chopped soil particles rise in the drill hole and overflow at the top of the casing through a T connection. The washwater is collected in a container. The casing can be extended with additional pieces as the borehole progresses; however, that is not required if the borehole will stay open and not cave in. Wash borings are rarely used now in the United States and other developed countries. Fig 2.6 Wash boring Rotary drilling is a procedure by which rapidly rotating drilling bits attached to the bottom of drilling rods cut and grind the soil and advance the borehole. There are several types of drilling bit. Rotary drilling can be used in sand, clay, and rocks (unless they are badly fissured). Water or drilling mud is forced down the drilling rods to the bits, and the return flow forces the cuttings to the surface. Boreholes with diameters of 50 to 203 mm can easily be made by this technique. The drilling mud is a slurry of water and bentonite. Generally, it is used when the soil that is encountered 11 Chapter Two Site Investigation is likely to cave in. When soil samples are needed, the drilling rod is raised and the drilling bit is replaced by a sampler. With the environmental drilling applications, rotary drilling with air is becoming more common. Percussion drilling is an alternative method of advancing a borehole, particularly through hard soil and rock. A heavy drilling bit is raised and lowered to chop the hard soil. The chopped soil particles are brought up by the circulation of water. Percussion drilling may require casing. 2.5 Procedures for Sampling Soil There are two types of samples: Disturbed Samples - These types of samples are disturbed but representative, and may be used for the following types of laboratory soil tests: Grain size analysis. Determination of liquid and plastic limits. Specific gravity of soil solids. Determination of organic content. Classification of soil. But disturbed soil samples cannot be used for consolidation, hydraulic conductivity, or shear tests, because these tests must be performed on the same soil of the field without any disturbance (to be representative). The major equipment used to obtain disturbed sample is (Split Spoon) which is a steel tube has inner diameter of 34.93 mm and outer diameter of 50.8mm. Undisturbed Samples - These types of samples are used for the following types of laboratory soil tests: Consolidation test. Hydraulic Conductivity test. Shear Strength tests. 11 Chapter Two Site Investigation These samples are more complex and expensive, and it’s suitable for clay, however in sand is very difficult to obtain undisturbed samples. The major equipment used to obtain undisturbed sample is (Thin-Walled Tube). 2.6 Type of Samplers 2.6.1 Split –Spoon Sampling Split-spoon samplers can be used in the field to obtain soil samples that are generally disturbed, but still representative. A section of a standard split-spoon sampler is shown in Fig. 2.7a. The tool consists of a steel driving shoe, a steel tube that is split longitudinally in half, and a coupling at the top. The coupling connects the sampler to the drill rod. The standard split tube has an inside diameter of 34.93 mm and an outside diameter of 50.8 mm ; however, samplers having inside and outside diameters up to 63.5 mm and 76.2 mm , respectively, are also available. When a borehole is extended to a predetermined depth, the drill tools are removed and the sampler is lowered to the bottom of the hole. The sampler is driven into the soil by hammer blows to the top of the drill rod. The standard weight of the hammer is 622.72 N, and for each blow, the hammer drops a distance of 0.762 m. The number of blows required for a spoon penetration of three 152.4-mm (6-in.) intervals are recorded. The number of blows required for the last two intervals are added to give the standard penetration number, N, at that depth. This number is generally referred to as the N value (American Society for Testing and Materials, 2014, Designation D- 1586-11). The sampler is then withdrawn, and the shoe and coupling are removed. Finally, the soil sample recovered from the tube is placed in a glass bottle and transported to the laboratory. This field test is called the standard penetration test (SPT). Fig. 2.8a and b show a split-spoon sampler unassembled before and after sampling. 12 Chapter Two Site Investigation Fig. 2.7 (a) Standard split-spoon sampler; (b) spring core catcher Fig. 2.8 (a) Unassembled split-spoon sampler; (b) after sampling (Courtesy of Professional Service Industries, Inc. (PSI), Waukesha, Wisconsin) The degree of disturbance for a soil sample is usually expressed as (2.3) 13 Chapter Two Site Investigation where AR = area ratio (ratio of disturbed area to total area of soil) Do = outside diameter of the sampling tube Di = inside diameter of the sampling tube When the area ratio is 10% or less, the sample generally is considered to be undisturbed. For a standard split-spoon sampler, Hence, these samples are highly disturbed. Split-spoon samples generally are taken at intervals of about 1.5 m. When the material encountered in the field is sand (particularly fine sand below the water table), recovery of the sample by a split-spoon sampler may be difficult. In that case, a device such as a spring core catcher may have to be placed inside the split spoon (Fig. 2.7b). At this juncture, it is important to point out that several factors contribute to the variation of the standard penetration number N at a given depth for similar soil profiles. Among these factors are the SPT hammer efficiency, borehole diameter, sampling method, and rod length (Skempton, 1986; Seed, et al., 1985). The two most common types of SPT hammers used in the field are the safety hammer and donut hammer. They are commonly dropped by a rope with two wraps around a pully. on the basis of field observations, it appears reasonable to standardize the field penetration number as a function of the input driving energy and its dissipation around the sampler into the surrounding soil, or (2.4) where N60 = standard penetration number, corrected for field conditions N = measured penetration number H = hammer efficiency (%) B = correction for borehole diameter 14 Chapter Two Site Investigation S = sampler correction R = correction for rod length Variations of H, B, S, and R, based on recommendations by Seed et al. (1985) and Skempton (1986), are summarized in Table 2.5. Table 2.5 Variations of H, B, S, and R Correlations for N60 in Cohesive Soil Besides compelling the geotechnical engineer to obtain soil samples, standard penetration tests provide several useful correlations. For example, the consistency of clay soils can be estimated from the standard penetration number, N60. In order to achieve that, Szechy and Vargi (1978) calculated the consistency index (CI) as (2.5) where w = natural moisture content (%) LL = liquid limit PL = plastic limit The approximate correlation between CI, N60, and the unconfined compression strength (qu) is given in Table 2.6. 15 Chapter Two Site Investigation Table 2.6 Approximate Correlation between CI, N60, and qu Hara, et al. (1971) also suggested the following correlation between the undrained shear strength of clay (cu) and N60. (2.6) where pa = atmospheric pressure (< 100 kN/m2; < 2000 lb/in2). The overconsolidation ratio, OCR, of a natural clay deposit can also be correlated with the standard penetration number. On the basis of the regression analysis of 110 data points, Mayne and Kemper (1988) obtained the relationship (2.7) where 'o = effective vertical stress in MN/m2. It is important to point out that any correlation between cu, OCR, and N60 is only approximate. Stroud (1974) suggested that cu = K N60 (2.8) K= constant = 3.5 – 6.5 kN/m2 N60 = standard penetration number obtained from the field 16 Chapter Two Site Investigation Correction for N60 in Granular Soil In granular soils, the value of N60 is affected by the effective overburden pressure, 'o. For that reason, the value of N60 obtained from field exploration under different effective overburden pressures should be changed to correspond to a standard value of 'o. That is, (2.9) where (N1)60 = value of N60 corrected to a standard value of 'o = pa [