Achalasia Alters Physiological Networks PDF
Document Details
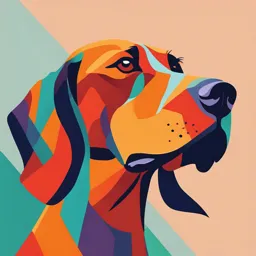
Uploaded by LavishNarwhal7802
Instituto Nacional de Ciencias Médicas y Nutrición Salvador Zubirán
2024
Janette Furuzawa‑Carballeda
Tags
Summary
This scientific report investigates how achalasia, a rare esophageal motility disorder, affects physiological networks in male and female patients. The study analyzes immunological biomarkers, network topology, and potential risk factors such as prior infections. The findings suggest that immunological involvement in achalasia appears localized in men but systemic in women.
Full Transcript
www.nature.com/scientificreports OPEN Achalasia alters physiological networks depending on sex Janette Furuzawa‑Carballeda 1,7, Antonio Barajas‑Martínez 2,7, Paola V. Olguín‑Rodríguez 2,3,...
www.nature.com/scientificreports OPEN Achalasia alters physiological networks depending on sex Janette Furuzawa‑Carballeda 1,7, Antonio Barajas‑Martínez 2,7, Paola V. Olguín‑Rodríguez 2,3, Elizabeth Ibarra‑Coronado 4, Ruben Fossion 2,3, Enrique Coss‑Adame 5, Miguel A. Valdovinos 5, Gonzalo Torres‑Villalobos 6 & Ana Leonor Rivera 2,3* Achalasia is a rare esophageal motility disorder for which the etiology is not fully understood. Evidence suggests that autoimmune inflammatory infiltrates, possibly triggered by a viral infection, may lead to a degeneration of neurons within the myenteric plexus. While the infection is eventually resolved, genetically susceptible individuals may still be at risk of developing achalasia. This study aimed to determine whether immunological and physiological networks differ between male and female patients with achalasia. This cross-sectional study included 189 preoperative achalasia patients and 500 healthy blood donor volunteers. Demographic, clinical, laboratory, immunological, and tissue biomarkers were collected. Male and female participants were evaluated separately to determine the role of sex. Correlation matrices were constructed using bivariate relationships to generate complex inferential networks. These matrices were filtered based on their statistical significance to identify the most relevant relationships between variables. Network topology and node centrality were calculated using tools available in the R programming language. Previous occurrences of chickenpox, measles, and mumps infections have been proposed as potential risk factors for achalasia, with a stronger association observed in females. Principal component analysis (PCA) identified IL-22, Th2, and regulatory B lymphocytes as key variables contributing to the disease. The physiological network topology has the potential to inform whether a localized injury or illness is likely to produce systemic consequences and the resulting clinical presentation. Here we show that immunological involvement in achalasia appears localized in men because of their highly modular physiological network. In contrast, in women the disease becomes systemic because of their robust network with a larger number of inter-cluster linkages. Achalasia is a primary esophageal motor disorder. It is a rare disease with an annual incidence of 1–5 cases and a prevalence of 7–32 cases per 100,000 individuals. Achalasia can occur at any age, but incidence and prevalence increase with age, and the mean age at diagnosis is between 30 and 60 years, without distinction of ethnicity. It is characterized by a failure to relax the lower esophageal sphincter (LES) in response to swallowing. It is accom- panied by aperistalsis of the esophageal body, resulting in severe dysphagia, regurgitation, aspiration, chest pain, and weight l oss1. Some studies have proposed that achalasia may be an autoimmune d isease2, wherein the poten- tial causal agent could be a neurotropic virus that chronically infects the myenteric plexus of the esophagus in genetically susceptible hosts, leading to the breakdown of immunological tolerance3. Proposed infectious agents include herpes simplex virus type 1 (HSV-1, a neurotropic virus with a preference for mucosal squamous epithe- lium, which persists in a latent state in neurons and induces a strong humoral and cellular immune response), varicella-zoster, measles, human papillomavirus, mumps, John Cunningham virus (commonly known as “JC”, a member of the polyomavirus family), and bornavirus (a related disease of psittacine birds that shows many similarities to achalasia)3,4. The susceptibility to develop achalasia has been associated with the HLA-DQβ1 alleles (HLA-DQβ1*03:04, HLA-DQβ1*05:03, HLA-DQβ1*06:01, and DQβ1*0602), HLA-DQα1 (HLA-DQα1*01:03), and HLA-DRβ*127-9. In the mestizo-Mexican population, the HLA class II alleles HLA-DRB1*14:54:01 and DQB1*05:03:01, as well as the extended haplotype, are risk factors for achalasia5. In patients with achalasia, the 1 Department of Immunology and Rheumatology, Instituto Nacional de Ciencias Médicas y Nutrición Salvador Zubirán, 14080 Mexico, Mexico. 2Centro de Ciencias de la Complejidad, Universidad Nacional Autónoma de México, 14060 Mexico, Mexico. 3Instituto de Ciencias Nucleares, Universidad Nacional Autónoma de México, 14060 Mexico, Mexico. 4Departamento de Fisiología, Facultad de Medicina, Universidad Nacional Autónoma de México, 14060 Mexico, Mexico. 5Department of Gastroenterology, Instituto Nacional de Ciencias Médicas y Nutrición Salvador Zubirán, 14080 Mexico, Mexico. 6Departments of Surgery and Experimental Surgery, Instituto Nacional de Ciencias Médicas y Nutrición Salvador Zubirán, 14080 Mexico, Mexico. 7These authors contributed equally: Janette Furuzawa-Carballeda and Antonio Barajas-Martínez. *email: [email protected] Scientific Reports | (2024) 14:2072 | https://doi.org/10.1038/s41598-024-52273-3 1 Vol.:(0123456789) www.nature.com/scientificreports/ LES muscle exhibits diverse inflammatory infiltrates (known as plexitis), primarily composed of lymphocytes and macrophages, with a lesser presence of polymorphonuclear cells. The characterization of the intraganglionic infiltrate shows a predominance of CD3+, CD4+ (Th22, Th17, Th2, and Th1), CD25+ and CD8+ T lymphocytes, as well as CD20+ B cells, eosinophils, and plasma cells located along the nerve bundles and around the ganglion cells4,6–9. Inflammatory infiltrates enriched for T and B cells generally predominate in tissues with advanced dis- ease (> 10 years of symptomatic history)6. In achalasia, mast cell infiltration is associated with nitrergic nerves, S-100 positive cells, interstitial cells of Cajal, and neuronal d egeneration10–13. An essential feature includes a reduction in the count of ganglion cells and Cajal cells, accompanied by fibrosis, which can account for as much as 21% of the overall tissue c omposition14. Furthermore, the LES muscle demonstrates an accelerated turnover of the extracellular matrix, driven by human gelatinase MMP-9 in its 92-kDa proform and 85-kDa active form. These enzymes contribute to the degradation of PNMA/Ta2 and GAD65, proteins expressed ectopically, which seem to be involved in the development of organ-specific autoimmune diseases, such as achalasia (referred to as REGA or ‘remnant epitopes generated autoimmunity’, mediated by cytokine-regulated tissue proteolysis)14,15. This proteolysis is associated with anti-GAD65 and anti-PNMA/Ta2 tissue-specific serum autoantibodies in patients with achalasia3,14. Proteomic studies and serum profile analysis of patients with achalasia have revealed significantly higher concentrations of pro-inflammatory cytokines (IL-22, IL-17, IL-4, IL-12, IL-6, IFN-γ , TNF- α, etc.), C4B5, C3, cyclin-dependent kinase 5, α2-macroglobulin, and anti-GAD65, anti-PNMA/Ta2, anti-tri- osephosphate isomerase, anti-carbonic anhydrase, and anti-creatinine kinase brain antibodies, when compared to those in healthy controls16–21. Finally, approximately 17–19% of patients with achalasia have autoimmune comorbidity, with 53% having hypothyroidism, and 68% having a family history of autoimmunity. When com- pared to individuals with gastroesophageal reflux disease (GERD), achalasia patients are 3.8 times more prone to autoimmune diseases (95% CI 1.47–9.83), 3.0 times more susceptible to thyroid disorders (95% CI 1.00–9.03), and 3.02 times more inclined to experience chronic inflammatory conditions (95% CI 1.65–6.20)22. However, achalasia is still not fully understood. Exploring the intricate interactions among various individual variables, including genes, proteins, physiological responses, and immunological reactions, to comprehend the emergence of system-wide behaviors is a current and ongoing challenge. Among various methods suitable for studying entire systems, the network physiology approach has yielded promising outcomes, especially by demonstrating the correlation between system-wide behavior, specifically physiological function, and network t opology23,24. For instance, network approaches have previously been theorized to better elucidate the origin of autoimmune diseases14. This study aims to examine the main topological characteristics of a physiological network built from measurements of immunological biomarkers, including biopsy and in situ detection of neurons and immune cells, serum measurements of cytokines and autoantibodies, and flow cytometry classification of white blood cells. Employing this approach, we demonstrate how variations in physiological network topology can predict whether the development of achalasia tends to be localized or systemic in men and women, respectively. Results Childhood exanthems are common in patients with achalasia A total of 189 patients with idiopathic achalasia were included in the study, comprising 116 (61%) females with a mean age of 42 ± 15 years and a disease duration of 24 months (range 1–150 months) at the time of assessment. The most frequent type of achalasia was type II (65%), followed by type I (29%) and type III (6%). The group of 500 healthy donors consisted of 315 females (comprising 63% of the total), with an average age of 36 ± 12 years. Clinical manifestations in patients with achalasia included dysphagia (98%), regurgitation (89%), heart- burn (58%), and weight loss (87%) (Table 1). The prevalence of autoimmune comorbidity was 13% in achalasia patients, and 16% had a history of allergy or asthma. 64% and 31% of the patients had chickenpox or measles during childhood (Table 2). A systematic analysis was conducted to discern sex-based distinctions. Notably, there were no discernible variations in the clinical presentation of achalasia or its medical management between the two sexes. Differences emerged exclusively in hemoglobin concentration, tobacco smoke exposure, alcohol consumption, height, and body weight Local and systemic immune activity in achalasia Several variables, including serum cytokine content and a complete blood count evaluated by fluorescence- activated cell sorting (FACS), were assessed to obtain an immunological overview of achalasia (Tables 3, 4; Fig. 1). Fluorescence-activated cell sorting, immunohistochemistry, and serum cytokine measurements are complemen- tary techniques that provide insights into the number of cells in circulation or infiltrating affected tissues, as well as the levels of cytokines released into the bloodstream (Table 5). For instance, a specific type of immune cell may exhibit elevated levels in the systemic circulation without necessarily infiltrating the affected tissue, while another immune cell type might be highly concentrated in the tissue but not present in significant quantities in the circulation (Table 6). This indicates diapedesis and tissue-specific infiltration. Immunohistochemistry was utilized to identify local biomarkers within the resected tissue following surgical treatments (Table 7). Not all patients in the clinical management group qualified for all follow-up investigations. Consequently, sample sizes for routine procedures were substantial, while data for non-standard procedures were limited. Due to this con- straint, complete blood count measurements were divided into four control groups based on age and sex, while the two achalasia groups were categorized by sex alone. Circulating white blood cell subsets were analyzed via flow cytometry, leading to the creation of two control and two achalasia groups, differentiated by sex. In the case of serum measures, they allowed for a division between healthy subjects and achalasia patients. The concentra- tion of hemoglobin, the width of the distribution of red blood cells, and the number of platelets were measured. Both male and female achalasia patients showed lower platelet counts than younger and older healthy controls. Scientific Reports | (2024) 14:2072 | https://doi.org/10.1038/s41598-024-52273-3 2 Vol:.(1234567890) www.nature.com/scientificreports/ Healthy donors Achalasia (all) Type I Type II Type III (n = 500) (n = 189) (n = 59) (n = 123) (n = 7) Demographics Age (years) Mean ± SD 36 ± 12 42 ± 15 42 ± 15 42 ± 14 50 ± 17 Median 36 41 40.5 40 44 Range 18–63 18–79 18–79 18–77 26–77 Sex female, n (%) 315 (63) 116 (61) 26 (44) 85 (69) 5 (71) Disease evolution (mo), Mean ± SD NA 24 ± 27 27 ± 30 23 ± 27 18 ± 16 Median NA 12 12 12 12 Range NA 1–150 1–150 1–132 4–48 BMI (kg/m 2 ) mean ± SD NA 23 ± 5 23 ± 4 23 ± 5 27 ± 2 Median NA 22 23.4 21.9 26.7 Range NA 14.6–36.8 15.6–33.0 14.6–36.8 25.3–28.1 Overweight, n (%) NA 58 (31) 15 (25) 39 (32) 4 (57) Obesity, n (%) NA 21 (11) 7 (12) 14 (11) 0 (0) Symptoms Dysphagia, (n%) NA 185 (98) 55 (93) 123 (100) 7 (100) Regurgitation, (n%) NA 168 (89) 51 (86) 112 (91) 5 (71) Heartburn, (n%) NA 109 (58) 29 (49) 79 (64) 1 (14) Weight loss, (n%) NA 164 (87) 47 (81) 111 (90) 6 (86) Table 1. Demographic description of the database. Healthy donors (n = 500) Achalasia (all) (n = 189) Type I (n = 59) Type II (n = 123) Type III (n = 7) Personal antecedents Autoimmune disease, (n%) NA 24 (13) 6 (10) 20 (16) 2 (29) Allergy and asthma, (n%) NA 30 (16) 10 (17) 20 (16) 0 (0) Viral exanthematous disease Chickenpox, n (%) NA 121 (64) 35 (59) 83 (67) 3 (43) Rubella, n (%) NA 18 (10) 4 (06) 14 (11) 0 (0) Measles, n (%) NA 58 (31) 22 (37) 34 (28) 2 (29) Environmental exposure Tobacco smoke exposure, n (%) NA 56 (30) 14 (24) 39 (32) 3 (43) Alcohol consumption, n (%) NA 65 (34) 22 (37) 41 (33) 2 (29) Biomass smoke exposure, n (%) NA 39 (21) 12 (20) 25 (20) 2 (29) Table 2. Personal antecedents and exposure. Only male achalasia patients exhibited an increase in the width of the distribution of red blood cells, but without alterations in hemoglobin levels (Table 3). As for the total white blood cell count, no significant differences were observed between groups, except for a slight decrease in the number of leukocytes in female patients with achalasia compared to their healthy counter- parts (Fig. 2). Cell counts of neutrophils and eosinophils were similar. A decline in the number of monocytes was observed with increasing age, and among the groups, young males and females exhibited the highest monocyte counts. Both basophil and lymphocyte cell counts were decreased in both men and women with achalasia, yet there was no significant difference in the neutrophil-to-leukocyte ratio (NLR). Flow cytometry was used to divide lymphocytes into distinct subsets. Patients with achalasia had increased circulating CD4+ T cells, CD25- T cells, CD161+ T cells, FOXP3+ Tregs, IDO-expressing regulatory plasmacytoid dendritic cells (pDCregs), and IL-10-expressing regulatory B cells (Bregs) compared to healthy individuals (Fig. 3). Finally, only IL-10 was significantly increased in achalasia patients (Fig. 4). Lymphocyte response as the main contributor to the immune response in achalasia We have previously hypothesized that achalasia is an autoimmune disease2. Therefore, the strategy of the pre- sent contribution included the collection of a variety of immune-related physiological data from local (Table 6) and systemic tissues (Table 6). Applying principal component analysis (PCA) to the data as in previous contributions24–26, we identified the most important variables contributing to the first two principal compo- nents (PC1 and PC2), i.e., those two mutually perpendicular vectors that capture the majority of variance within the sample. This method allowed us to identify how white blood cell subpopulations (Th1, Th2, Th17, Tregs, Scientific Reports | (2024) 14:2072 | https://doi.org/10.1038/s41598-024-52273-3 3 Vol.:(0123456789) www.nature.com/scientificreports/ Healthy donors Achalasia (all) Type I Type II Type III (n = 500) (n = 189) (n = 59) (n = 123) (n = 7) Laboratory variables Hemoglobin (g/dL) mean ± SD 15 ± 1 15 ± 2 15 ± 2 15 ± 2 15 ± 1 Median 15.3 14.8 15.2 14.5 15.7 Range 13.3–18.6 10.0–18.2 10.0–17.8 10.1–18.2 13.9–16.8 Platelets (103 cells/mL) mean ± SD 275 ± 52 231 ± 60 231 ± 53 234 ± 64 176 ± 46 Median 271 223 226.5 226 163 Range 144–460 128.0–427.0 145.0–392.0 135.0–427.0 128.0–258.0 Leukocytes (103 cells/mL) mean ± SD 7±1 6±2 6±2 7±2 6±2 Median 6.9 6.2 6 6.5 5.5 Range 3.6–11.2 2.9–12.6 3.0–12.6 2.9–11.2 4.0–7.9 Lymphocytes (%) mean ± SD 33 ± 7 30 ± 9 31 ± 8 30 ± 10 33 ± 7 Median 32.6 30.1 31.2 29.5 32.3 Range 19.9–55.9 5.5–54.9 10.9–47.0 5.5–54.9 24.5–42.5 Monocytes (%) mean ± SD 7±2 7±2 7±2 7±2 7±1 Median 7.6 7.1 7.6 6.8 6.6 Range 3.8–14.3 1.0–13.6 4.4–13.6 1.0–13.0 5.7–8.6 Neutrophils (%) mean ± SD 55 ± 8 59 ± 10 58 ± 10 60 ± 11 58 ± 7 Median 55.3 59.9 59 60 58.7 Range 37.9–71.5 30.0–92.4 37.0–78.4 30.0–92.4 47.1–68.0 Eosinophils (%) mean ± SD 2±2 3±2 3±2 2±2 1.6 ± 0.5 Median 2 2.3 2.3 1.6 1.6 Range 0.2–9.5 0.6–13.0 0.4–13.0 0.0–14.5 1.0–2.4 Basophils (%) mean ± SD 1.0 ± 0.3 0.6 ± 0.5 0.7 ± 0.6 0.6 ± 0.5 0.6 ± 0.4 Median 1 0.5 0.6 0.5 0.5 Range 0.2–2.1 0.0–5.0 0.0–3.7 0.0–5.0 0.3–1.3 NLR mean ± SD 1.8 ± 0.6 2±2 2±1 3±2 1.9 ± 0.6 Median 1.7 2 1.9 2 1.8 Range 0.6–4.8 0.6–15.7 0.9–7.2 0.6–15.7 1.1–2.8 CRP (mg/dl) mean ± SD NA 0.4 ± 0.7 0.5 ± 0.8 0.4 ± 0.7 0.4 ± 0.2 Median NA 0.2 0.2 0.2 0.3 Range NA 0.0–4.8 0.0–3.5 0.0–4.8 0.1–0.6 Table 3. Complete blood count and C reactive protein. Bregs and pDCregs) and cytokines (IL-22) relate to achalasia (Fig. 5). The second component PC2 is centered on the detection and quantitative assessment of interstitial cells of Cajal (c-Kit+ cells), ganglion cells (VIP+), gelatinase MMP-9-2D9+ cells, as well as the percentages of circulating lymphocytes and neutrophils within the tissue (Fig. 5). Moreover, we adjusted the previous method to increase the readability of the data structure. We utilized Hoeffding’s D, a measure of statistical dependency, instead of correlation between pairs of variables. Whereas correlations can be positive or negative, statistical dependence tends to be positive. As a result, variables were clustered more closely. Neutrophils and lymphocytes contributed significantly to PC2, albeit in opposite ways. Consequently, these measures are the same component when statistical dependence is assessed. Their contributions to the whole dataset became evident and prominent, and consequently they are positioned along the first component (Fig. 5). Additionally, we observed that subsets of Th1, Th2, Th17, IL-22, Bregs, pDCregs, and the cell number of MMP-9-3G12 positive cells, interstitial cells of Cajal, and ganglion cells are linked. Vari- ables regarding the clinical history of the patients are now prominent and shown along the second component. In conclusion, the data-driven strategy separated the most significant contributions from the data structure into three categories: first, neutrophil and lymphocyte variables of the complete blood count; second, variables related to circulating cell subsets, serum cytokines, and tissue biomarkers; and third, clinical data, including fundoplication and Heller myotomy. Topology of physiological networks differs between men and women with achalasia Given the physiological distinctions between men and women25, particularly in their immunological responses, we conducted separate analyses for each sex. This division remains clear in the correlation matrix of patients with achalasia (Fig. 6). Men need only four principal components to summarize and reconstruct the matrix, whereas women need at least eighteen components (Fig. 6). The difference in the number of necessary compo- nents associated with the modularity of each matrix highlights a significant distinction: correlations between variables in men are notably distinct and independent, contrasting with those in women. It is worth noting that the majority of the physiological characteristics obtained for this study are related to the immune system. Scientific Reports | (2024) 14:2072 | https://doi.org/10.1038/s41598-024-52273-3 4 Vol:.(1234567890) www.nature.com/scientificreports/ Healthy donors (n = 500) Achalasia (all) (n = 189) Type I (n = 59) Type II (n = 123) Type III (n = 7) Circulating CD4+ T cell subpopulations CD4+/CD161-/IL-22+ (%) mean 2.3 ± 0.1 8.5 ± 0.4 9.4 ± 0.7 7.9 ± 0.5 10 ± 2 ± SD Median 2.51 7.74 9.05 6.98 11.7 Range 0.35–3.90 2.75–19.9 5.79–19.9 2.75–15.6 6.93–13.0 CD4+/CD161+/IL-17A+ (%) 2.0 ± 0.1 7.6 ± 0.3 7.9 ± 0.5 7.3 ± 0.3 9.8 ± 0.8 mean ± SD Median 2.12 7.61 8.11 7.55 10.5 Range 0.41–3.47 2.68–14.8 2.68–13.5 2.95–14.8 8.05–10.7 CD4+/CD25-/IL-4+ (%) mean 2.0 ± 0.1 7.1 ± 0.3 6.6 ± 0.4 7.5 ± 0.5 5.7 ± 1.7 ± SD Median 2.08 6.41 6.33 6.54 5.92 Range 0.23–3.93 2.72–17.1 2.81–10.2 2.90–17.1 2.72–8.58 CD4+/CD25-/IFN-γ + (%) mean 2.0 ± 0.1 6.9 ± 0.3 6.8 ± 0.5 6.9 ± 0.4 7±3 ± SD Median 1.91 6.17 6.31 6.12 4.9 Range 0.29–4.65 2.23–13.4 2.44–11.1 2.99–13.4 2.23–12.8 CD4+/CD25hi/Foxp3+ (%) 6.5 ± 0.3 9.2 ± 0.3 9.5 ± 0.4 8.9 ± 0.4 10 ± 1 mean ± SD Median 6.83 8.9 9.08 8.87 10.1 Range 1.34–9.20 1.36–15.6 6.26–13.5 1.36–15.6 8.07–12.3 CD19+/CD24+/CD38+/IL-10+ 8±3 10 ± 3 9±3 10 ± 3 16 ± 1 (%) mean ± SD Median 8.4 10.8 9.3 11 15.8 Range 2.0–13.7 4.1–17.4 4.1–14.0 4.1–15.9 14.6–17.4 CD123+/CD196+/IDO+ (%) 16.9 ± 0.6 37± 1 32 ± 2 38 ± 2 46 ± 2 mean ± SD Median 17.2 36 31.6 38.1 45.7 Range 6.79–28.1 16.8–62.0 21.5–47.4 16.8–62.0 43.1–49.3 Table 4. Fluorescence-activated cell sorting. Healthy donors (n = 500) Achalasia (all) (n = 189) Type I (n = 59) Type II (n = 123) Type III (n = 7) Serum interleukins IL-1β , mean ± SD 13 ± 3 37 ± 13 32 ± 17 38 ± 18 42 ± 20 Median 1 1 1 1 17.3 Range 1.00–64.1 1.00–1002 1.00–324 1.00–1002 1.00–131 IL-6, mean ± SD 30 ± 5 49 ± 10 21 ± 5 40 ± 9 148 ± 45 Median 13.8 22.6 16.6 21.4 115 Range 1.42–156 1.42–354 1.95–76.0 1.42–354 35.0–324 IL-10, mean ± SD 9±2 23 ± 3 30 ± 11 23 ± 3 9.8 ± 0.5 Median 7.1 11.4 15.9 11.6 9.5 Range 0.90–28.0 0.32–105 2.39–105 0.32–74.2 8.00–11.6 IL-17A mean ± SD 32 ± 8 120 ± 40 59 ± 16 109 ± 60 220 ± 51 Median 24 30.7 51 17.4 300 Range 0.23–137 2.86–979 20.9–106 2.86–979 57.3–303 IL-25 mean ± SD 7±4 25 ± 9 26 ± 16 13 ± 4 13 ± 83 Median 1 1 1 1 1 Range 1.00–105 1.00–506 1.00–248 1.00–166 1.00–506 TNF-α mean ± SD 2.3 ± 0.3 3.7 ± 0.4 4.5 ± 1.1 3.2 ± 0.4 6.3 ± 1.4 Median 2.15 2.4 2.73 1.87 6 Range 2.10–7.46 0.2–19.8 0.8–19.8 0.2–14.1 1.4–11.8 Table 5. Serum cytokines concentrations. Scientific Reports | (2024) 14:2072 | https://doi.org/10.1038/s41598-024-52273-3 5 Vol.:(0123456789) www.nature.com/scientificreports/ Healthy donors Achalasia (all) Type I Type II Type III (n = 500) (n = 189) (n = 59) (n = 123) (n = 7) Tissue cell populations IL-22-expressing cells (%) mean ± SD 3±1 14 ± 7 7±4 15 ± 4 29 ± 6 Median 3 15 6 16 28 Range 2.0–5.5 4.0–35.0 4.0–18.0 7.0–22.0 21.0–35.0 IL-17A-expressing CD4 T cells (%) mean ± SD 1.1 ± 0.9 12 ± 5 12 ± 3 8±1 19 ± 3 Median 1 10.3 12 8 19 Range 0.0–2.0 7.0–22.0 9.0–19.5 7.0–11.0 16.0–22.0 IL-4-expressing CD4 T cells (%) mean ± SD 1.6 ± 0.7 7±6 4±1 3.5 ± 0.6 17.8 ± 0.8 Median 1.5 4 4 3.5 18 Range 1.0–3.0 2.0–19.0 3.0–5.0 2.0–4.0 17.0–19.0 IFN-γ -expressing CD4 T cells (%) mean ± SD 2±1 9±7 14 ± 2 4.8 ± 0.5 18 ± 6 Median 1.5 5.7 13 6 15 Range 0.5–3.5 3.5–29.0 12.5–16.0 3.5–6.0 13.0–29.0 Foxp3-expressing CD25 T cells (%) mean ± SD 4.4 ± 0.6 7±3 6±3 7±3 10 ± 2 Median 4.5 8 7.5 7.5 10 Range 3.5–5.0 3.0–13.5 2.3–9.0 2.0–13.5 8.0–13.0 IL-10-expressing CD20 B cells (%) mean ± SD 1.7 ± 0.5 6±3 14 ± 7 4.5 ± 0.8 10 ± 2 Median 1.5 4.5 15 4.5 10 Range 1.0–2.5 3.0–12.0 4.0–35.0 3.5–6.5 7.0–12.0 IDO-expressing CD123 cells (%) mean ± SD 3.8 ± 0.7 7±2 3.7 ± 0.6 3.7 ± 0.6 10 ± 2 Median 3.8 6 4 4 10 Range 3.0–4.0 4.0–13.0 3.0–4.0 3.0–4.0 9.0–13.0 GAD65-expressing cells (%) mean ± SD 0.3 ± 1.3 39 ± 22 44 ± 19 31 ± 10 70 ± 12 Median 0 33.2 44.3 32 68.3 Range 0.0–5.3 6.3–83.0 16.0–67.0 6.3–63.3 58.7–83.0 PNMa/Ta2-expressing cells (%) mean ± SD 0.3 ± 1.1 24 ± 12 22 ± 8 22 ± 5 47 ± 20 Median 0 21.7 12.6 19.9 43.3 Range 0.0–4.3 0.0–74.7 0.0–74.7 0.0–60.7 28.7–68.7 S100-expressing cells (%) mean ± SD 9±7 22 ± 4 20 ± 4 20 ± 4 52 ± 20 Median 10 18 18 13.3 55.7 Range 0.0–19.3 0.0–69.7 0.0–51.3 0.0–54.0 30.3–69.7 P Substance-expressing cells (%) mean ± SD 1±2 1.7 ± 0.5 2.2 ± 0.7 1.6 ± 0.9 0.6 ± 0.4 Median 0.2 0.6 1 0 0.3 Range 0.0–7.3 0.0–16.3 0.0–7.7 0.0–16.3 0.3–1.0 VIP cells (ganglion cells)/cm 2 (%) mean ± SD 60 ± 21 1.4 ± 0.6 0.9 ± 0.4 0.4 ± 0.4 0.0 ± 0.0 Median 55.9 1 0 0 0 Range 35.0–105.3 1.0–14.0 0.0–4.0 0.0–7.8 0.0–0.0 Interstitial cells of Cajal (c-Kit)/cm 2 (%) mean ± SD 338 ± 144 96 ± 22 127 ± 42 98 ± 29 4±4 Median 314.7 52.2 71.5 49 5 Range 190.9–776.2 0.0–608.4 7.0–608.4 7.8–479.0 0.0–7.0 MMP-9 3G12-expressing cells (%) mean ± SD 4±2 11 ± 9 14 ± 10 11 ± 1 5±2 Median 4.2 9.8 14.5 9.5 6.2 Range 0.0–5.7 0.7–4.7 4.3–46.3 0.7–40.7 3.0–6.3 MMP-9 2D9-expressing cells (%) mean ± SD 0.4 ± 0.9 0.9 ± 0.2 0.7 ± 0.3 1.2 ± 0.4 0.8 ± 0.2 Median 0 0.3 0.2 0.3 0.7 Range 0.0–3.0 0.0–4.7 0.0–4.3 0.0–4.7 0.7–1.0 Table 6. Tissue cell populations. Consequently, we primarily observe the behavior of the immunological variables. According to a network analy- sis of the correlations, men and women exhibit distinct interactions (Fig. 7). To build clusters of physiological variables, we employed three different methods: first, we established topological clusters using an energy-model layout in which node position is governed by edge density; a second technique involved optimizing a modularity function to generate node-containing clouds; and a third method implied optimizing the description length of a random walker, an information criterion, to color the border of each node. Similarly to what was found by PCA, physiological networks in men may be summarized by only three nodes while in women ten nodes are necessary Scientific Reports | (2024) 14:2072 | https://doi.org/10.1038/s41598-024-52273-3 6 Vol:.(1234567890) www.nature.com/scientificreports/ Healthy donors Achalasia (all) Type I Type II Type III (n = 500) (n = 189) (n = 59) (n = 123) (n = 7) Auto-antibodies GAD65, (%) NA 83 89 80 80 PNMA2, (%) NA 90 89 87 100 Recovering, (%) NA 0 0 0 0 Yo, (%) NA 0 0 0 0 Zic4, (%) NA 7 0 13 0 Titin, (%) NA 3 0 7 20 SOX1, (%) NA 3 11 7 0 Hu, (%) NA 3 11 0 0 Ri, (%) NA 3 11 0 0 Amphiphysin, (%) NA 3 0 0 20 Antinuclear antibodies n, (%) NA 78 (41) 19 (32) 54 (44) 5 (71) Table 7. Autoantibodies presence. atasets25,26, the network for women has a higher edge density (Fig. 7). Some (Fig. 7C,D). As shown for other d associations appear confined to a specific sex when searching for specific distinctions between the sexes. For instance, biomass exposure and eosinophil concentration correlate with clinical presentation only in females (Fig. 8), while immunological factors in males appear to be more strongly interconnected. Discussion Idiopathic achalasia is an archetypal esophageal motor disorder caused by persistent inflammation at the Auer- bach plexus, resulting from a loss of inhibitory neurons of the myenteric esophageal plexus. The etio- and physi- opathogenic mechanism for achalasia is thought to involve a repetitive insult from a neurotropic infectious agent. Progression to the disease only occurs in infected individuals with a genetic predisposition for an aggressive, chronic inflammatory response. In most cases, pathology is accompanied by neuronal antibodies that contrib- ute to the destruction of the myenteric plexus. For achalasia, multiple reports are associated with autoimmune diseases such as polyglandular autoimmune syndrome Type II27,thyroid disease28, vitiligo29, and common vari- able immunodeficiency30. In previous studies, and also the present contribution, several systemic biomarkers of immune response were detected at higher levels in patients with achalasia (Fig. 5), particularly those involved with white blood cell subtypes. Nonetheless, these biomarkers encompass various physiological systems, includ- ing age, sex, innate and adaptive immune cell subsets, autoantibodies, clinical characteristics, etc. This growing array of available biomarkers lacks an explanatory framework on how and why homeostasis becomes altered by achalasia. Using PCA, we showed that these systemic biomarkers are the main drivers of achalasia (Fig. 5), inducing the variance of the physiological measures of the patients. Moreover, we showed how, exclusively for women, the history of previous childhood exanthemata infections (mumps, measles, chickenpox and rubella) is a leading influencer and mediator within the physiological network (Fig. 8). Indeed, even if traditional (univariate) statistical approaches fail to detect differences in average values between groups for a variety of immunological variables, there are notable differences in how these variables are positioned within the corresponding (multivari- ate) network (Fig. 8). It is not preposterous to propose a viral etiology in achalasia because previous studies have established a link between viral infection and the trigger of the disease. For example, a longitudinal analysis has shown compellingly that Epstein–Barr virus infection is the main trigger of multiple s clerosis31,an autoimmune disease that until the last decade had no clear cause. An important environmental factor must be deduced for some autoimmune diseases due to a high percentage of disease-discordant monozygotic twin p airs32.There are several theoretical mechanisms by which infections may act as triggers of a utoimmunity32–34. In the present study, it was found that men and women with achalasia were equally infected in childhood by exanthemata viral infections, and there were no meaningful differences in immune physiological variables between sexes. However, these diseases had a significant contribution to the immune landscape in which achalasia occurs. This suggests that, although no single viral infection may be responsible for achalasia, vaccination against preventable diseases is a priority for women. One limitation of the study is its cross-sectional nature, which makes it difficult to deduce causal connections. The biomarkers employed were chosen based on their availability and accessibility. Another significant limitation of the study is that some measures, such as FACS, are expensive and therefore not widely available for clinical practice, while others, such as a biopsy of the LES, cannot be justified in healthy subjects. Despite these limitations, the correlational approach enabled us to show how physiological variables behave differently in each group. Sex-dependent differences in network topology25 may determine how the disease presents and develops. The male physiological network is more adaptable and modular, making it more vulnerable to pathologies that attack multiple systems simultaneously, such as COVID-1926. In contrast, female networks are more robust and have multiple inter-cluster links, making them more likely to spread out the impact of localized diseases (Figs. 7, 8). Scientific Reports | (2024) 14:2072 | https://doi.org/10.1038/s41598-024-52273-3 7 Vol.:(0123456789) www.nature.com/scientificreports/ Conclusion From a network perspective, it is possible that the robust (densely connected) physiological network of women makes them more susceptible to long-term immune changes after various childhood viral exanthems, while these viral exanthems are not as influential in the modular network of men. Prevention of viral infections must be prioritized as part of medical care particularly, in women. Further longitudinal population studies are required to determine whether changes in several viral infections reflect incidence changes in achalasia. Methods Design This was an exploratory, observational, and cross-sectional study conducted at a tertiary referral care center (Instituto Nacional de Ciencias Médicas y Nutrición Salvador Zubirán) between April 2015 and December 2021. Participants included 189 consecutive patients with idiopathic achalasia (Type I: n = 58; Type II: n = 124; and Type III: n = 7) and 500 healthy control donors. Not all medical procedures are justified for healthy control donors, hence tissue biopsies, flow cytometry and auto-antibody determination were not performed extensively in the control group. Patients All patients were diagnosed by high-resolution manometry (HRM), upper endoscopy, and esophagogram. Patients aged ≥ 18 years were enrolled in the study. Exclusion criteria included Chagas disease, esophageal stricture, esophageal scleroderma, gastric or esophageal cancer, peptic stricture, other esophageal motility dis- orders, pregnancy, hematologic disease, cancer, severe renal or liver disease, ongoing infection, or use of aspirin or steroid treatment. Clinical records of patients were carefully reviewed according to a pre-established protocol. Data were collected retrospectively for each study participant from the medical records of the hospital, includ- ing demographic features, type of achalasia, family history of autoimmunity, and current diagnosis of organ or systemic autoimmunity. When a concurrent autoimmune diagnosis was identified, we extracted all pertinent information, including the date of diagnosis, symptoms at the time of diagnosis, clinical and laboratory confirma- tory test results, and administered treatment. Finally, chronic inflammatory conditions (i.e., asthma, allergic rhinitis, gout, and rosacea) were also extracted. Complete blood count (CBC) parameters were the latest labora- tory findings recorded before surgical intervention. For comparison, 500 healthy controls who volunteered at the blood bank were recruited for the study to obtain healthy CBC parameters (Table 1). None of the included controls had cardiovascular, metabolic, inflammatory, or neoplastic disease. Demographic, clinical, and labora- tory information were also collected. Laboratory information All CBC analyses were performed with an automatic hematologic analyzer (Beckmancoulter DxH 800 Hematol- ogy Analyzer). Hemoglobin (Hb), white blood cell (WBC), neutrophils, lymphocytes, monocytes, eosinophils, and platelet counts were obtained before surgical treatment. Blood samples were collected in di-potassium ethylenediaminetetraacetic acid tubes. Flow cytometry Peripheral blood mononuclear cells (PBMCs) were obtained by gradient centrifugation on Lymphoprep (Axis- Shield PoC AS, Oslo, Norway). The cell pellet was resuspended in 1 mL RPMI at 1–2 ×106 cell/mL. The cell suspension was treated with 2 µL of a cell activation cocktail of phorbol-12myristate 13-acetate (40.5 mM) and ionomycin (669.3 mM) in DMSO (500×) and brefeldin A (BioLegend Inc., San Diego, CA) for 6 h at 37 ◦ C in a CO2 incubator. PBMCs were incubated with 5 mL of Human TruStain FcXTM (BioLegend Inc.) per million cells in 100 mL PBS for 10 min. Then they were labeled with 5 mL of anti-human CD3-FITC-labeled, anti- human CD4-PeCy5-labeled, and anti-human CD161- APC-conjugated monoclonal antibodies (BD Biosciences, San Jose, CA); anti-human CD3-FITC-labeled, anti-human CD4-PeCy5-labeled and anti-human CD25-APC- conjugated monoclonal antibodies (BD Biosciences) in separated tubes during 20 min at 37 ◦ C in the dark. Cells were permeabilized with 200 mL of cytofix/cytoperm solution (BD Biosciences) at 4 ◦ C for 30 min. Intracel- lular staining was performed with an anti-human IL-22-PE-, IL-17A-PE-, IL-4-PE-, IFN-γ -PE-labeled mouse monoclonal antibodies (BD Biosciences) for 30 min at 4 ◦ C in the dark. An electronic gate was made for CD3+/ CD4+/CD161- cells, CD3+/CD4+/CD161+ cells, and CD3+/CD4+/CD25-cells (Fig. 1). Results are expressed as the relative percentage of IL-22+, IL-17A+, IL-4+, and IFN-g+ expressing cells in each gate (Fig. 2). As isotype control, IgG1-FITC/IgG1-PE/CD45-PeCy5 mouse IgG1 kappa (BD Tritest, BD Biosciences) was used to set the threshold and gates in the cytometer. We ran an unstained (autofluorescence control) and permeabilized PBMCs sample. Autofluorescence control was compared to single-stained cell positive controls to confirm that the stained cells were within the normal range for each parameter. Additionally, BD Calibrate 3 beads were used to adjust instrument settings, set fluorescence compensation, and check instrument sensitivity (BD calibrates, BD Biosciences). Fluorescence minus one (FMO) control was stained in parallel using the panel of antibodies with the sequential omission of one antibody, except for the anti-IL-22, anti-IL-17A, anti-IL-4, anti-IFN-γ , which was replaced by an isotype control rather than omitted. Finally, T subsets were analyzed by flow cytometry with an Accuri C6 (BD Biosciences). 500,000 to 1,000,000 events were recorded for each sample and analyzed with the FlowJo X software (Tree Star, Inc.). Scientific Reports | (2024) 14:2072 | https://doi.org/10.1038/s41598-024-52273-3 8 Vol:.(1234567890) www.nature.com/scientificreports/ Antibody Origin Antigen retrieval Dilution Origin VIP Mouse monoclonal Citrate buffer of pH 6 1:150 Santa Cruz Biotechnology, c-kit (CD117) Rabbit monoclonal Citrate buffer of pH 6 1:500 BioSB, Santa Barbara, CA GAD65 Rabbit polyclonal Citrate buffer of pH 6 1:100 Abcam PNMa Rabbit polyclonal EDTA buffer of pH 8 1:100 Abcam S100 Mouse monoclonal Citrate buffer of pH 6 1:3000 Abcam P Substance Mouse monoclonal Citrate buffer of pH 6 1:3000 Abcam REGA-3G12 Mouse monoclonal Citrate buffer of pH 6 1:100 Rega Institute REGA-2D9 Mouse monoclonal Citrate buffer of pH 6 1:100 Rega Institute Table 8. Antibodies for immunohistochemistry. COMPLETE BLOOD COUNT FLUORESCENCEACTIVATED CELLSORTING Erythrocytes Platelets Leukocytes Basophils Monocytes Lymphocytes Neutrophils Eosinophils Th1 Tregs Th22 Th17 Th2 Bregs pDC IDO IL-10 IL-4 IL-17 INF- SERUM CYTOKINES IL-22 FOXP3 Other cytokine sources Figure 1. Circulating components of the immune system. Through an incremental approach we performed a complete blood count which differentiates leukocytes from lymphocytes, monocytes, basophils, eosinophils and neutrophils. Then the lymphocytes were furthed sorted by multiple fluorescent markers into Th1, Th2, Tregs, Bregs, Th17, Th22 and pDCs. Finally, serum cytokines were measured in circulation by luminometry. COMPLETE BLOOD COUNT a Erythrocytes b Platelets c Leukocytes Erythrocytes Platelets Leukocytes Basophils Monocytes Lymphocytes Neutrophils Eosinophils d Neutrophils e Lymphocytes f Monocytes g Basophils h Eosinophils Figure 2. Differences in blood count composition between healthy participants and achalasia patients. Box and whisker plots are used to display the median, interquartile range, and range for hemoglobin concentration and all cellular blood components, including leukocytes includes neutrophils, lymphocytes, monocytes, basophils, and eosinophils. Statistical significance is summarized by levels and represented by colored letters (a–e), where groups with the same letter have comparable significance levels. Scientific Reports | (2024) 14:2072 | https://doi.org/10.1038/s41598-024-52273-3 9 Vol.:(0123456789) www.nature.com/scientificreports/ Th1 Tregs Th22 LEUKOCYTES a b c Neutrophils Eosinophils Basophils Monocytes Th1 Tregs Th22 Th17 Th2 Bregs pDC IDO IL-10 IL-4 IL-17 INF- IL-22 FOXP3 d Th17 e Th2 f Bregs g pDC Figure 3. Differences in lymphocyte subtypes between healthy individuals and patients with achalasia. Box and whisker plots show the median, interquartile range, and range of the percentages of fluorescence-activated cell sorting of lymphocytes in men and women with and without achalasia, identified by biomarkers. Statistical significance is summarized by levels and represented by colored letters a-d, where groups with the same letter have comparable significance levels. a b c (pg/mL) (pg/mL) (pg/mL) FLUORESCENCEACTIVATED CELLSORTING Th1 Tregs Th22 Th17 Th2 Bregs pDC IDO IL-10 IL-4 IL-17 INF- IL-22 d e f FOXP3 (pg/mL) (pg/mL) (pg/mL) Figure 4. Cytokine serum concentration in healthy controls and achalasia patients. Box and whisker plots illustrate the median, interquartile range, and range of serum cytokine concentrations. Statistical significance is summarized by levels and represented by colored letters a,b, where groups with the same letter have comparable significance levels. Scientific Reports | (2024) 14:2072 | https://doi.org/10.1038/s41598-024-52273-3 10 Vol:.(1234567890) www.nature.com/scientificreports/ a b Mixed correlation matrix PCA Hoeffding's D matrix PCA fundoplication 0.2 c−Kit Blood transfusions Heller miotomy VIP NLR 0.03 Hypothyroidism Thyroid autoimmunity VIP c MM−2 Alergies Neutrophils N ttrr p Esophageal dilations −K Kitt c c−Kit Neutrophils ph s (10³/mL) (1 Gastroduodenal sseries e CD8 / CD28− VIP area Measles es a a H ig Height Leukocytes e ko oc D1 CD24hi/CD38hi CD19/ /C C 38 8 stt P Sust Bregs GADD 65 6 CD4 / CD161− CD8 / CD28−/ FOXP3 c−Kit area 0.1 FO CD + CD4+/CD25− 5−− /IL−4+ /IL 0.00 T Treg Tregs CD CD4+/ CDD D1 CD161−/ IL L2 IL22+ Reflux Lymphocytes Ly Ly p (10³/mL) ( ) Leukocytes Monocytes Mon on (10³/mL) L)) ia Heller Dysphagia Ale le ergie er Alergies efo ore orre sickness BMI before sick Neutrophils (10³/mL) ler miotomy to omy PC PCR cos2 cos2 C 9 Esophageal sop opp ea dilations lat at ns n RDW RD DW (%) ((%P latelets Pla ate at CD4+/ CD161+/ IL−17+ CD19 CD P [ ]/cm² VIP ]/c cm² m² PC2 (10.7%) CD4D4 D 4 CD D −// N CD4/CD25−/INF− NFF− F− CD4 4 0.03 Platelets P atte at s Sust elets st P 0.005 PC2 (5.2%) Blood Blood d trttransfusions raanssf sfu f MMP9 3G12 VIP Eosinophils (%) CD4 /CD25− fundoplication fu fun nd plicatiio ndo on o n 0.06 Chicago ag aggo GAD 0.010 S1000 Pyrosis Pyr Pyroosis i G 65 Neutrophils Neutrop p (%) 0.09 Tregs 0.015 pDCs Rubella Mumps Mum Muumps ps 0.12 CD4D4 D 4 / CD161− D 0.020 CD4/CD161 1 C4 C4 H. H pylori py Lymphocytes (%) C3 Ag A g ge Age e NLR CD4 /CD25hi CD25hi /FOXP3X CD19 /CD24hi h CD19/ CD24hi/CD38hi/IL−10 24 h 24 8h 8 0.0 CD19/ CD24hi/CD38hi/IL−10 Basophils B hilils hils Ottth O lss t Otherhe her h e s k O er smoke smo sm Overweight contrib contrib PNM PNNMa NM PNMa Ma M a Eossin Eosinophils (10³/mL) −0.03 Gastroduodenal series eries es CD C D123 CD123 6 16