Consumables Used in Axle Shop (PDF)
Document Details
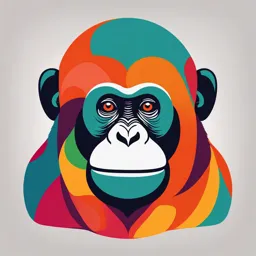
Uploaded by ImaginativePeachTree
Rail Wheel Factory (RWF)
Tags
Related
- Workshop Technology (1) - Engineering Materials - PDF
- Materials for Civil and Construction Engineers 4th Edition PDF
- EVO UT 5.1 PDF - Tecnología de los Materiales (Evaluaciòn) - IC - 2024
- Examen de Tecnología de los Materiales (Evaluaciòn) - IC - 2024 - EVO UT 5.0 - Curso Tarde PDF
- Aceros de Construcción y Herramientas PDF
- Experimental Investigation on Mechanical Properties of Normal Concrete Reinforced with Discarded Steel Fibers PDF
Summary
This document provides details on the steel blooms and forging hammers used in rail manufacturing. It details the specifications, applications, and requirements for these consumables. The document also explains several mechanical properties of materials, including tensile testing.
Full Transcript
57 Consumables used in Axle shop The following consumables used in Rail Wheel Factory are essentially needed to be multi-sourced. The particulars given here under are indicative and are meant to help suppliers for developing and supplying the same to RWF. The axle consumables are: Consum...
57 Consumables used in Axle shop The following consumables used in Rail Wheel Factory are essentially needed to be multi-sourced. The particulars given here under are indicative and are meant to help suppliers for developing and supplying the same to RWF. The axle consumables are: Consumable Name: Steel Blooms for forging Diesel/Electric loco and EMU Driving axles as per IRS R 43 and IRS R 16 Application/ Job requirement: The steel blooms shall be manufactured from steel made by basic electric arc furnace or any other process recommended as equivalent and approved by the purchasing railways. The steel must be degassed under vacuum. Hydrogen content in the liquid steel is determined by standard analysis method on LECO hydrogen analyzer. Hydrogen content of the steel is determined by the standard analysis method on LECO nitrogen analyzer. Consumable Name: Forging Hammer Application/ Job requirement: The forging hammers are used for forging different steel axles and armature shaft for railway use. Forging Hammers are made from DIN 56NiCrMoV7 steel quenched and tempered to 34 HRC. The reclamation process is described in the RWF specification for reclamation. Entire process followed for reclamation must be documented and be made available to inspecting officials. 58 M Lab Properties of materials Every material is known by its set of properties. A variety of tests are conducted to evaluate and compare the mechanical properties of different materials. Some of the popular tests conducted include the tensile test, Compression test, Shear test, Hardness test, Impact test, bending test and so on. Among all the tests tensile test gives maximum information about mechanical properties. MECHANICAL PROPERTIES Yield Strength: 59 It can be defined as the stress at which plastic deformation (yielding) begins without any appreciable increase in load. Yield strength, σy = Py /A0 N/m2 Where Py = load at yield point A0 = original area of cross section. Ductility: It is the ability of the material to undergo plastic deformation under tensile load. A material with high ductility undergoes severe plastic deformation before fracture takes place. Percentage elongation gives a measure of ductility. % elongation = (L – L0) / L0 * 100 Where L = length of specimen after the tensile test. L0 = original length. Malleability: It is the ability of the material to undergo plastic deformation under compressive load. Ultimate tensile strength: After the yield point the specimen undergoes continuous plastic deformation with increase in load. This load reaches a certain maximum value after which the specimen does not take any more load. At the point of ultimate load, the area of cross section of the specimen starts reducing and is known as Necking. Ultimate tensile strength, σu = Pu / A0 N/m 2 Where Pu = ultimate load A0 = original area of cross section 60 Fracture strength: After the ultimate load is reached, the load drops and at a particular load fracture takes place. The stress corresponding to this load where the actual failure takes place is known as Fracture strength of the material. 2 Fracture strength, σf = Pf / A0 N/m Where Pf = fracture load or breaking load A0 = original area of cross section Toughness: The toughness of a material is its ability to withstand both elastic and plastic deformation. In other words, it is the amount of energy a material can withstand before fracture takes place. IRON CARBON EQUILIBRIUM DIAGRAM A study of the constitution and structure of all steels and irons must first start with the Iron carbon equilibrium diagram. Iron is an allotropic metal which means that it can exist in more than one type of crystal structure depending upon temperature. Pure iron exists as BCC structure at room temperature and is called as FERRITE or α- IRON. At 912 degrees Celsius BCC Iron undergoes allotropic changes and becomes FCC structure which is retained till 1394 degree Celsius. FCC Iron is also known as AUSTENITE or γ – IRON. At 1394 degree Celsius it changes back once again to BCC structure called δ – FERRITE and this is retained till its melting point at 1538 degree Celsius. In Iron carbon equilibrium diagram, the left vertical axis represents 100% iron and all the allotropic changes of pure iron with temperature. The right vertical axis does not represent 100% carbon but instead represents only 6.67% C by weight. This is because a maximum amount of 6.67% C can only 61 be added to molten iron at which it becomes saturated. Iron when it contains exactly 6.67% C by weight forms a phase called CEMENTITE or IRON CARBIDE Fe3C. Description of phases in the Fe-c system All the phases that appear on the Fe-c equilibrium diagram are equilibrium phases that is, they are the phases obtained upon very slow cooling at equilibrium conditions. Following are the different equilibrium phases of Fe-C. 62 α – FERRITE: It is nothing but an interstitial solid solution of small amounts of carbon dissolved in BCC iron. The maximum solid solubility of carbon in iron is 0.025% at 727 degrees Celsius and 0.008% at room temperature. It is the softest among all phases of iron and carbon. AUSTENITE It is again an interstitial solid solution of carbon but in FCC iron. The maximum solid solubility is 2.1%C at 1148 degree Celsius. Austenite is not stable below 727 degrees Celsius and will not be normally present in the micro structure at room temperature. It has an FCC structure and it is not magnetic. δ – FERRITE It is also an interstitial solid solution of carbon now BCC iron because austenite changes its structure at 1394 degree Celsius from FCC to BCC. Maximum solid solubility of carbon in δ-iron is 0.1%C at 1495 degree Celsius. CEMENTITE It is also known as iron carbide and has a chemical formula Fe3C. It is considered as chemical compound because it contains fixed carbon percentage but is crystalline in nature. Cementite always contains 6.67%C by weight and has an orthorhombic crystal structure. It is the hardest structure that appears on Fe-C diagram. The presence of cementite greatly enhances the properties of steel. PEARLITE When austenite containing exactly 0.83%C is cooled very slowly to below 727 degrees Celsius, it transforms into pearlite. Pearlite is nothing but lamellar structure of α- ferrite and cementite. It is a fairly soft structure and 63 its hardness lies between that of α-ferrite and cementite. It has a finger print like appearance. LEDEBURITE It is the eutectic lamellar mixture of austenite (light phase) and cementite (dark phase). The eutectic reaction occurs at 4.33%C at 1148 degree Celsius. Austenite in the eutectic mixture of ledeburite is unstable below 727 degrees Celsius and hence transforms into α-ferrite and cementite.