Microbial Cell Observation - Notes
Document Details
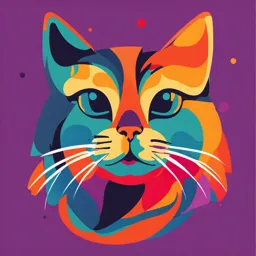
Uploaded by AgreeablePsaltery8661
Tags
Summary
These notes detail the observation of microbial cells, from early magnifying glasses to modern microscopes. They explore human eye limitations and the optics involved in microscopy. The notes provide a foundational understanding of biological observation.
Full Transcript
**[Chapter 1: Observing the Microbial Cell:]** - Introduction - Early Beginnings: - From simple magnifying glasses to 1^st^ century microscopes developed in 17^th^ century - Van Leeuwenhoek's Legacy: - Antonie van Lee. Pioneering use of handcrafted...
**[Chapter 1: Observing the Microbial Cell:]** - Introduction - Early Beginnings: - From simple magnifying glasses to 1^st^ century microscopes developed in 17^th^ century - Van Leeuwenhoek's Legacy: - Antonie van Lee. Pioneering use of handcrafted microscopes to discover and document microscopic life - Impact on Science: - Opened new fields of scientific inquiry, allowing for the exploration of cellular and microbial worlds - Human Eye Anatomy and Vision Limitations: - Components: - Cornea, lens, retina, and optic nerve work together to focus light and transmit images to the brain. - Function: light enters through the cornea, is focused by the lens, and converted to neural signals by the retina (principle: focal length) - Limitations in Observing Microscopic Entities: - Minimum Resolvable Size: - The smallest object visible to the naked eye is about 0.1 mm; microbial cells, typically smaller, cannot be resolved without magnification - Human eyes are designed for movement and not detail - Low Light Adaptation: - Rods vs. Cones: Rods are sensitive to low light but provide low resolution; cones manage color and detail under better lighting conditions. - Color and Motion Detection: - Color Perception: Cones sensitive to red, green, and blue enable color vision, crucial under good lighting. - Motion Sensitivity: Human eyes are better at detecting motion than at resolving static fine details, an evolutionary trait for survival - Optics and Light Properties: - Refraction and Reflection: Light changes direction when entering a different medium (refraction) and bounces off surfaces (reflection), foundational for understanding how lenses manipulate light. - Lens Function in Microscopy: Convex lenses gather and focus light rays to a point, magnifying images and allowing detailed observation of tiny structures beyond the capability of the naked eye. - Link to Microscope Design: These principles guide microscope design, enhancing our ability to see at the cellular and subcellular levels by compensating for the human eye's limitations - Lens and Corrective Optics: - Basics of Lens Function: - Focusing Light: Lenses bend light rays to bring them into focus, with convex lenses converging rays to a focal point to magnify images. - Corrective Optics: - Vision Correction: Eyeglasses and contact lenses correct refractive errors by adjusting the focus of light onto the retina, improving vision clarity. - Microscopy Application: - Enhancing Microscope Vision: Microscope lenses refine light management to enhance the resolution and magnification, allowing detailed observation of microscopic entities. - Interplay of Lenses: - Compound Lenses: Microscopes use systems of multiple lenses to achieve higher magnification and precision, illustrating how combining lenses can compound their effect - Early Microscopes: - First Tools: Early microscopes were simple magnifying glasses developed in the late 16th century. - Antonie van Leeuwenhoek's Impact: - Microbial World Revealed: Using handcrafted microscopes, van Leeuwenhoek was the first to observe and describe bacteria and other microorganisms. Advancements in Microscope Design: - From Simple to Complex: Microscope technology evolved from single-lens designs to advanced compound microscopes with multiple lens systems, significantly improving resolution and magnification. - Scientific Revolution Triggered: - Unlocking New Scientific Fields: The enhancement of microscopes facilitated the detailed exploration of cellular structures and microorganisms, catalyzing advancements in biology, medicine, and materials science. - Bright-Field Microscopy: Fundamentals of Bright-Field Microscopy: - Basic Setup: - Utilizes visible light to illuminate the sample. - Light passes directly through or reflects off the sample into the objective lens, creating a clear image of the specimen against a bright background. - Primary Application: - Widely used for examining fixed and stained samples to observe cellular shapes, structures, and certain details within cells. - - Advantages: - Simple setup and operation, making it ideal for general microscopy education and basic biological research. - Limitations - Limited contrast in unstained or transparent samples, and resolution may not reveal the finest structures within a cell. - - Microbial Size and Shape: - Overview: range from cocci, bacilli, to spirilla, varied morphology in the microbial world. - Shape is important as it alters movement - SA:V ratio differs (cocci are more efficient due to larger ratio) - Diagnostic Importance: Microbial shapes and sizes are key to identifying species, understanding their ecology, and their roles in health and disease - Microscopy Challenges: - Size Range: Most microbes range from 0.2 to 10 microns, requiring advanced microscopy techniques for detailed observation - Advanced Microscopy Techniques: - Introduction to Advanced Techniques: - Beyond Basic Microscopy: fluorescence and super- resolution microscopy allow for detailed visualization beyond traditional light microscopy limits. - Super-Resolution Microscopy: - Breaking the Limit: Techniques like STED and PALM, surpass the diffraction limit of light to provide images with nanometer resolution. - Scientific Impact: ability to view structures at the molecular level, crucial for understanding complex biological mechanisms - Fluorescence Microscopy: - Key Concept: Utilizes fluorescent dyes or proteins to highlight specific parts of a cell under UV light, enabling detailed visualization of structures and processes - Application: Ideal for tracking dynamic processes within cells, identifying specific proteins, or observing cellular interactions. - Research Uses: Critical for studying cellular mechanisms, protein interactions, and genetic expression in real-time. - Diagnostic Capabilities: Enhances the detection of pathogens and cancer cells, improving accuracy and speed in medical diagnostics - Staining Techniques: - Enhances Visibility: Stains add contrast to microscopic samples, making transparent or colorless structures visible under a microscope. - Common Staining Methods: - Gram Staining: Differentiates bacteria into Gram- positive and Gram-negative based on cell wall properties, crucial for bacterial identification and antibiotic treatment decisions. - Methylene Blue: Used for staining cell nuclei and identifying cell structures, often used in clinics to observe blood cells. - Staining and Microscopy: - Complementary Techniques: Stains are used in combination with various microscopy techniques to enhance detail and provide specific insights into the composition and function of cells - Chemical and Super-Resolution Imaging: - Super-resolution and chemical imaging techniques break through the traditional resolution limits of light microscopy, allowing for nanoscale visualization. - Super-Resolution Techniques: - Examples: Techniques like STED (Stimulated Emission Depletion) and PALM (Photoactivated Localization Microscopy) provide detailed views of structures at a molecular level, crucial for understanding cellular dynamics and interactions. Chemical Imaging: - Unique Insights: Combines microscopy with spectroscopic techniques to gather spatial and chemical information, enabling detailed analysis of material compositions and changes within cells - Electron Microscopy: - Powerful Imaging: Uses beams of electrons instead of light to achieve much higher resolutions, allowing scientists to view structures down to the atomic level. - Types of Electron Microscopy: - Transmission Electron Microscopy (TEM): Electrons pass through the sample, revealing internal structures and arrangements. - Scanning Electron Microscopy (SEM): Electrons scan the sample surface, providing detailed topographical maps. - Applications in Science: - Biological and Material Sciences: Critical for studying cellular structures, virus morphology, and material surfaces in nanotechnology and metallurgy - Scanning Probe Microscopy: - Direct Surface Interaction: SPM techniques involve a probe that physically scans the surface of a sample at a very close distance, allowing for the direct measurement of surface properties at the nanoscale. - Types of Scanning Probe Techniques: - Atomic Force Microscopy (AFM): Measures surface roughness, detects mechanical properties, and can manipulate individual atoms or molecules. - Scanning Tunneling Microscopy (STM): Uses quantum tunneling of electrons between the probe and the sample to create atomic-scale images of surfaces. - Applications Across Disciplines: - Material Science and Nanotechnology: Essential for characterizing material surfaces, engineering molecular structures, and developing nanodevices. - Biophysics and Cellular Biology: Used to investigate the mechanics of cell membranes, protein interactions, and other biomolecular structures - X-Ray Crystallography: - Crystal Analysis: X-ray crystallography involves directing X-ray beams at a crystallized sample to produce diffraction patterns that can be analyzed to determine the atomic and molecular structure of materials. - Key Applications: - Structural Biology: Essential for determining the three-dimensional structures of biological molecules, particularly proteins and nucleic acids, which are crucial for understanding their function and interaction - Impact on Drug Development: - Pharmaceuticals: The technique\'s ability to elucidate the precise arrangement of atoms within a molecule aids in the rational design of drugs, allowing for the development of medications that can precisely target specific biological pathways - Cryo-Electron Microscopy: - Revolutionary Technique: Cryo-EM freezes biomolecules in their natural state and uses electron microscopy to visualize samples at near-atomic resolution without the need for crystallization. - Advantages Over Traditional Methods: - No Crystallization Needed: Unlike X-ray crystallography, Cryo-EM does not require the sample to be crystallized, making it possible to study large complexes and transient structures that are difficult to crystallize. - Applications in Structural Biology: - Complex Structures: Ideal for studying the structure of large protein complexes, viruses, and other cellular components, providing insights that are critical for understanding biological functions and mechanisms. - Live Cell Imaging: - Dynamic Visualization: Live cell imaging allows scientists to observe and record cellular processes in real time, providing a dynamic view of cellular functions. - Technological Foundations: - Advanced Microscopy: Utilizes techniques such as confocal microscopy, fluorescence microscopy, and time-lapse imaging to capture the ongoing biological activities within living cells. - Biological Insights: - Cellular Interactions and Behaviors: Critical for understanding how cells interact, respond to stimuli, and undergo processes like division, migration, and apoptosis under physiological conditions - Multidisciplinary Contributions: - Collaborative Innovation: Advances in microscopy are driven by collaboration across physics, engineering, chemistry, and biology, enhancing tool capabilities and applications. - Technological Synergies; Combining Expertise: - Physicists and engineers contribute advanced imaging technologies, while biologists and chemists provide insights into sample preparation and molecular interactions - Current Challenges in Microscopy: - Technical Barrier: ongoing efforts to break beyond the diffraction limit of light and the electron beam resolution in electron microscopy. - Complexities in Sample Preparation: - Preservation Challenges: difficulties in preparing samples that do not alter cellular structures or functions, critical for accurate observation. - Operational Complexity: User-Friendly Interface Needs: for more intuitive controls and training to make advanced microscopes accessible to a broader range of researchers - Practical Applications of Microscopy: - Health Sciences: Disease Identification: Microscopy is crucial for diagnosing diseases by identifying pathogens or abnormal cells in clinical samples, such as in tissue biopsies or blood tests. - Ecosystem Monitoring: Use microscopy to study environmental samples, monitoring microorganisms that indicate the health of ecosystems or water quality. - Material Science; Nanotechnology Development: Microscopy aids in the design and analysis of materials at the nanoscale, crucial for developing new materials with enhanced properties like increased strength or conductivity **[Chapter 1: Microbial Life: Origin and Discovery]** - What are Microbes: - Microscopic entities that exist as single cells or cluster. - Includes bacteria, archaea, fungi and some algae and protozoa - Importance: essential for nutrient cycling, env sustainability, human health - Virus: - Acellular entities that consist of genetic materials enclosed in protein coat - Unlike cellular organisms, virus requires host cell to replicate, hijacking host cellular machinery to reproduce - Virus does not meet criteria for living independently - Criteria for living: growth, reproduction, metabolism, response to stimuli, cellular structure - No growth; only assemble and disassemble - No metabolism; lack machinery - Only reproduce by infection into host cell - Introduction to genomic sequencing - Is the process of determining complete DNA sequence of organism's genome at single time. - Significance: transformed ability to diagnose diseases, understand genetic diversity and trace evolutionary histories accelerating advances in med, agriculture and ecology - Power of metagenomics: - Metagenomics extends genomic analysis to entire communities of microbes, directly from env samples without culture. - Captures DNA from millions - Application: particularly valuable in env where vast microbes cannot be cultured in labs (eg: deep sea vents or highly acidic soils) - Over 99% of microbe species cannot be cultured with current methods - Metagenomics revealed genetic material of these, shedding light on their functions and interactions within ecosystems - Speed and Scale of Modern Genomics: - Recent advances: NGS have accelerated pace of genomic data acquisition. Can sequence entire genome in a single day (rather than years like before) - Has made large-scale projects (like earth microbiome project) feasible - Case Studies in Genomic Sequencing: - Medical Microbiology: rapid genome seq of pathogens helps in quickly identifying infection agents during outbreaks timely and effective responses - Env Impact: genomic sequencing identifies microbes that can detoxify pollutants, aiding in bioremediation projects to clean up contaminated sites - Future: - Looking forward, technologies like CRISPR for gene editing and single-cell sequencing are poised to further revolutionize our understanding of microbial functions and interactions. - Intro to History of Microbiology: - Roots extend back several centuries. - History is marked by ground breaking experiments and theories that have shaped understanding of infectious diseases, hygiene and role of microbes in Earth's ecosystem - Disproving Spontaneous Generation: - [Francesco Redi]: in late 1600s, he conducted experiments showing maggots on decaying meat. Significant challenge to notion of spontaneous generation - [Louis Pasteur:] In 1861, he performed famous swan neck flask experiments, demonstrating that microbial growth in nutrient broths came from external contamination and not spontaneity. - Role of Early Microscopy: - Invention of microscope in late 16^th^ century revolutionized biology and allowed first direct observations of microorganism - [Antonie van Leeuwenhoek], using handcrafted microscope, was first to observe and describe bacteria, free-living and parasitic microscopic protists, sperm cells and blood flow in capillaries in 1670s - Florence Nightingale and the Birth of Medical Statistics: - F.N was pioneer figure in nursing; used statistical charts to persuade govt officials about importance of hospital sanitary reform during Crimean War - Impact: Not only demonstrated critical rs between hygiene and patient survival rates but also established use of statistical analysis in studying health outcomes; laid groundwork for epidemiology - Microscopy and its Impact on microbiology - Early microscopes enabled scientists to discover world of micro-orgs, changing medical field by identifying agents causing diseases - Throughout 19^th^ and 20^th^ century, advancements in microscopy (like electron microscope) allowed for greater understanding of microbial structures and their processes at cellular and molecular levels - Medical Microbiology - Focuses on study of pathogens, diseases they cause, how or bodies defend against them - Pivotal in fields of public health, epidemiology and infection control - Koch's Postulates: - These postulates are criteria for establishing causative rs between microbe and disease - Steps: - Micro-org must be found in abundance in all organisms suffering from disease but not found in healthy orgs - Micro-org must be isolated from diseased org and grown in pure culture - Cultured microbe should cause disease when introduced into healthy org - Microbe must be reisolated from inoculated, diseased experimental host and identified as identical to original specific causative agent - Revolution of Vaccination: - Historical context: practice of this dates back hundreds of years but scientific basis was established by Edward Jenner in 1790s with smallpox vaccine - Penicillin: while not a vaccine, discovery of this by Alexander Fleming in 1928 revolutionized treatment of bacterial infections and opened door for development of antibiotics, crucial in fight against bacterial diseases - Modern Vaccine and their Impact: - Has evolved to include inactivated pathogens, attenuated viruses or subunit vaccines. Each designed to safely expose immune system to component of pathogen to build immunity without causing disease - Vaccine has drastically reduced incidence of infectious diseases worldwide (polo, measles, diphtheria) saving millions annually - Challenges and Innovations in Vaccine - Some challenges include: vaccine hesitancy, access issues in low-income countries and need for vaccine against evolving pathogens like influenza virus - Future direction: should find more effective vaccination methods including DNA vaccines and viral vector vaccines - Environmental Microbiology: - Explores roles microbes play in natural and artificial env, focusing on interactions with ecosystem and each other - Winogradsky's column: Microbial Ecosystem model - Simple device for culturing diverse community of micro-orgs. By creating gradients of light, oxygen and nutrients, it mimics natural env and demonstrates microbial interactions and energy flows - Helps understanding lithotrophy-process by which microbes derive energy from inorganic compounds-and its role in geochemical cycling (for sustaining ecosystems) - Human Gut Microbiome: - Complex community that play crucial role in digestion, immunity and even mental health - Helps understand how these communities interact with host, influence health and adapt to changes in diet and env - Beyond earth: - Adaptability and resilience of microbes to extreme env on earth can lead scientists to hypothesize about possibility of microbial life on other planets - Astrobiology: microbial extremophiles provide models for types of life that might exist on planets or moons where conditions are harsh but potentially habitable -- -- -- -- -- -- -- -- -- -- **[\ ]** **[Chapter 3: Cell Structure and Function:]** - Bacterial Cell: - Core Components: - envelope includes: outer membrane, cell wall and periplasm, and inner cell membrane with an embedded chemoreceptor array. - Cytoplasm contains: enzymes, mRNA extending out the nucleoid, and ribosomes - Nucleoid contains chromosomal DNA wrapped around binding proteins  - Cell membrane: - Structure: composed of lipid bilayer with embedded proteins - Function: acts as barrier controlling entry and exit of substances; selective permeability - Different than human cell membrane since it contains ATP Synthase - Transport Across membrane: - Passive transport: movement of substances without energy, including diffusion and osmosis (Eg: O~2~) - Active Transport: requires E; moves substances against conc. gradient - Transport Proteins: Integral in facilitating movement of molecules - Method to Study Bacterial Cells: - Microscopy: key took for visualizing cell structures, including light (for overall structure) and electron microscopy (detailed view of internal components) - Staining Techniques: enhance contrast and detail in bacterial cells - Genetic and Biochemical Methods: used to analyze bacterial components and functions - Fractionation of Gram -ve Cells: - Periplasm is filled with sucrose; lysozyme breaks down cell wall. Dilution in water causes osmotic shock to outer membrane; periplasmic proteins leak out - Subsequent centrifugation steps separate the proteins of periplasm, cytoplasm and inner and outer membranes - EDTA: ethylenediaminetetraacetic acid; OMP = outer membrane protein - Bacterial Cell Wall: - Structure: Comprised mainly of peptidoglycan, gives wall its rigidity (providing strength and shape) - Function: protects against osmotic pressure and env stress - Hypertonic: higher conc. of solutes compared to another solution - Isotonic: equal conc. of solutes compared to another solution - Hypotonic: lower conc. of solutes compared to another solution - Gram Staining: differentiates bacteria based on cell wall structure - Gram +ve: Firmicutes have thick cell wall with multiple layers of peptidoglycan, threaded by teichoic acids. - Gram -ve: single layer of peptidoglycan covered by outer membrane. Cell membrane is called inner membrane. Outer membrane liposaccharides (LPS) are cross-linked by positively charged magnesium ions - Disaccharide unit of glycan attached to peptide chain of 4-6 a.a cross linked together strong network that protects cell, especially in env where osmotic pressure is a stress on the cell - Antibiotics (eg: penicillin) target synthesis of peptidoglycan cell death - Bacterial Envelope: - Multi-layer Protection: consists of cell membrane, cell wall, and outer membrane (in gram -ve bacteria); - Lipopolysacc: defending against substances like antibiotics in gram -ve bacteria - Role in Defense: Protects against harmful substances and environmental threats - Periplasmic Space: contains enzymes and transport proteins; critical zone for nutrients processing and degradation - Bacterial Cytoskeleton: - Structural support: adds rigidity to maintain cell shape and aids in cellular processes like division - Role in cell division: critical in guiding division process and septum formation - Components: Includes proteins like FtsZ and MreB (structural/shaping proteins) - FtsZ: maintains cell diameter by FtsZ polymerization to form the Z ring - Z ring: acts as scaffold that recruits proteins to form division machinery - MreB: allows rod shape cell to elongate (polymerizes around E.coli cell) and direct cell wall synthesis - CreS: crescentin; polymerizes along inner curve of crescent. Fused with Green Fluro Protein (CreS-GFP) localizes to inner curve of Caulobacter crescenrus. Membrane-specific stain FM4-64 (red fluorescence) localizes to the membrane around the cell - Bacterial Cell Division: - Binary Fission: main method of bacterial reproduction, involving DNA replication and cell splitting - Nucleoid Role: DNA must be accurately replicated and partitioned into daughter cell - Division Machinery: Includes divisome and FtsZ ring - Divisome Complex: protein complex that orchestrates cell division - Septation Process: formation of septum to separate daughter cells - Coordination with DNA Replication: ensures division occurs only after DNA is fully replicated - FtsZ: recruits other proteins to make septum - Bacillus subtilis septum grows from outer ring inward: - Cells were pulse-labelled with different colored fluorescent d-alanine mol incorporated in peptidoglycan and catalyzed by penicillin binding proteins. - HADA, blue (first 60 minutes); BADA, green (next 5 minutes); TADA, red (final 30 seconds). - Cell Polarity and Signaling: - Cell Polarity: involves asymmetric distribution of cellular components: - Role in Function: critical for processes like motility, cell division and differentiation - Signaling Pathway: coordinate cellular activities and responses to environmental cues. - Protein TipN: - appears at pole of Caulobacter stalk cell, visualized as TipN-GFP. - As cell grows, TipN delocalizes and localizes again at septum. Septation yields two daughter cells with TipN at pole of each (shows that proteins shuffles around to change polarity) - Lack of TipN protein makes mistakes, flagella grows out of stalk or at pole - Membrane Vesicles and Nanotubes: - Membrane vesicles: small spherical structures that transport materials between cells - Nanotubes: long, tubular structures (that connects cells); used for intercellular communication and material transfer - Significance: Play roles in pathogenesis, biofilm formation and horizontal gene transfer (one bacteria to another unrelated one) - Specialized Bacteria Structures: - Flagella and Pili: involved in motility and adhesion to surfaces - Storage Granules: reserve materials like glycogen and polyphosphate - Environmental Adaptations: enable bacteria to survive in diverse environments - Organelles of phototrophs: - contains photosynthetic double membranes called thylakoids. - Carboxysomes are polyhedral, protein-covered bodies packed with rubisco enzyme for CO~2~ fixation - Intracellular sulfur globules: dot the cytoplasm of T.namibiensis (anaerobic, thermophilic bacterium the gains energy by oxidizing H~2~S S - Bacterial Cell Shape and Function: - Morphological Diversity: shapes like cocci, bacilli and spirilla serve different functions - Rod: higher SA:V quick absorption of nutrients and expelling antib - Cocci: more efficient at forming biofilms (protect by forming barrier) - Spiral: move through env more efficiently (can invade better) -- -- -- -- -- -- -- -- -- -- -- -- -- -- -- -- -- -- -- -- **[Chapter 4: Bacterial Culture, Growth, and Development:]** - Microbial Nutrition: - Growth requirements: C, N, Energy, and water - Energy Source: phototrophs use light for E; chemotrophs use chemical reactions for organic and inorganic compounds - Carbon Sources: autotrophs use CO~2~; heterotrophs rely on organic compounds - Water: solvent for biochm reactions and maintaining cell structure - - Macronutrients: required in large amounts; essential for life - Making polymers - - C: all macromolecules - O: cellular metabolism - H: redox reactions and pH balance - N: a.a + DNA+ A - P: ATP, DNA, cell memb. - S: a.a - Cofactors - Mg^2+^:cofactor for many enzymes (DNA rep, protein synt. And ATP metabolism) and ribosome + N.a stabilization - Fe^2+^: redox reactions and ETC; key component in enzymes - K^+^ - Ca^2+^: stabilizing cell wall; regulating cell process like spore formation - Micronutrients: needed in small amounts; needed for enzyme function - - Cobalt: component of vit B12 ( metabolism of certain a.a and nucleotides) - Copper: enzymes in redox reactions and detoxifying O radicals - Manganese: cofactor of enzymes in detox of reactive oxygen species and photosynthesis - Molybdenum - Nickel - Zinc: Enzyme activity for DNA synthesis and transcription - Growth factors: some microbes require specific vitamins or amino acids - Vitamins: coenzymes - A.a : essential a.a that must be obtained from env (not synthesized) - Purines and pyrimidines: some bacteria cant synthesize these - Nutrient supplies limit microbial growth: limited by compound in least amount (ratio will be off) - Can trigger adaptations like siderophore production for iron acquisition or biofilm formation (help capture limited resource) - Energy and ATP Synthesis: - ATP as Energy: ATP powers cellular processes, produced through various pathways - Substrate-level phosphorylation: direct transfer of phosphate to ADP (PEP + ADP ATP) during glycolysis - Occurs in cytoplasm during glycolysis and mitochondrial matrix during kerbs cycle - Oxidative Phosphorylation: ATP is generated through ETC and chemiosmosis - ATP in each cycle in fermentation - - Glycolysis - Pyruvate oxidation - Krebs cycle - Oxidative phosphorylation - - Alternative Energy Gen Pathways: - Photophosphorylation: ATP generated by converting light E into chm E in thylakoid of chloroplasts (EK) or plasma memb (PK) - Fermentation: substrate-level phosphorylation - Nutrient Transport across membranes: - Passive transport: simple diffusion and facilitated diffusion allow molecules to pass without E - Active transport: requires E to move mol against their conc gradient - Mechanisms: - Symport/Antisymport: symport moves two substances at same direction; anti moves in opposite directions (eg of antiport: NA/K pumps) - Energy requirement: active transport uses ATP or Proton motive force - Examples: lactose uptake via proton symport in bacteria - Transport Proteins: Permease and transporters are critical for nutrient uptake - Phosphotransferase System: PTS - Effective mechanism used by bacteria to transport sugars across cell memb - Sugar molecules (glucose, mannose, mannitol and fructose) are phosphorylated during transport into cell - Energy efficiency: Conserves E by linking transport with phosphorylation - Regulation: prioritizes certain sugars based on env conditions and traps sugars in cells - Common in bacteria: important for glucose and mannose transport - PEP: source of P; attaches to sugar to hold it in cell - Enzymes: - E I: first enzyme in the chain, which accepts the phosphate group from PEP. - HPr: phosphoryl group is transferred from E1 to this, acts as carrier - E II: three domains: IIA, IIB and then P is transferred to sugar through IIC - Pathogens use this system to utilize host-derived sugars - Culturing and Counting Bacteria: - Pure Culture: isolating bacteria to study specific species under lab conditions - Dilution Streaking: technique to separate individual cells on agar plates - Can be used to see if sample is pure - Counting method: Viable plate counts and turbidity measurements - Agar: polysaccharide obtained from algae; tendency to solidify in specific temperatures; used to grow colonies - Broth culture: liquid culture; allows org to grow in 3D space grow more quickly - Indirect turbidity: measure per m^2^/ml - Broth: Turbidity; less light passes through more growth occurred - Adv: quick; non-invasive; measure bacterial growth real-time - Disadv: measure both living and dead; requires calibration curve - Assumption: microbe is culturable - Agar: measure per square - Serial dilute and spread sample on agar; count and multiply by dilution factor - Disadv: cant find details about microbes; time consuming - Adv: accurate; allows differentiation - Streak Plate method: bacteria picked up by sterile lop and streaked in pattern - Adv: simple and effective for obtaining pure cultures - Molecular tools: take portion of DNA and measure how many different types and how much of each type - Adv: doesn't depend on capturability - If bacteria is removable, can measure mass (dry weight measurement) - Adv: accurate measure of biomass - Disadv: time consuming and not suitable for small scale cultures - Bacterial Growth Curves: - Lag phase: period of adaptation before growth starts; no increase in cell \# - Log phase: rapid exponential growth where cells divide at their fastest rate - Required in culturing large quantities (nutrients constantly added and metabolism - Stationary phase: Growth plateaus as nutrients deplete ; cells form survival structures - Used in alcohol prod (or any fermentation production) - Used in antibiotic production - To maintain stress: add little nutrients - Death Phase: cells die off as conditions worsen - Strategists: - R: fast growing compared to other domains - K: slow growing compared to other domains - If not disturbed; K strategists can reach carrying capacity (plateau) and has longer life span - Batch and Continuous Cultures: - Batch culture: closed system where nutrients are limited, leading to distinct growth phases - Continuous Cultures: chemostats provide constant nutrient supply and waste removal allowing for steady growth - Applications: used in industrial fermentation and microbial research - Disturbance vs Stress: - Disturbance: mainly predation that removes biomass - Top-down factor in food web (above) - Stress: limiting resources due to competition (both inter and intra); anything that's further away from optimum (anything to do with external environment) - Bottom-up factor in food web (below) - Biofilm Formation: - Structure: layers of microbial cells attached to surface and embedded in matrix - EPS Matrix: extracellular polymeric substances; combination of protein and carbohydrates; stabilizes their community; protects cells and helps bacteria communicate - Importance: protects microbes from env stress and antibiotics - Stages: 1. Initial Attachment: to monolayer by flagella (by forces such as VWF or weak hydrophobic int) 2. Microcolonies: attachment is irreversible; bacteria start to secrete EPS for anchoring 3. Exopolysaccharide (EPS production): starts forming micro-colonies (starts dividing within) Cells communicate through quorum sensing 4. Mature Biofilm: complex (has channels to transfer nutrients and O~2~); phenotypic heterogeneity is high (different phenotype due to gradient of nutrient) and antib resistance (physical barrier) 5. Dissolution and Dispersal: Dissolution and Dispersal: conditions maybe better or could be poor opening of biofilm where they stay in local area or disperse - Quorum Sensing in Biofilms: - Quorum sensing: bacteria communicate by releasing and detecting signaling molecules called autoinducers - Different molecules are used by Gram +ve and -ve bacteria - Population density: once a threshold is reached, coordinated gene expression occurs, influencing biofilm formation - Biofilm advantages for genetic exchange including antibiotic resistance - - Industry: - Water systems: clog pipes - Present on surface of foods - Bioremediation - Human health: - Medical devices like catheters, - Chronic infections (CF) - Antibiotic resistance - - Endospore Formation: - Endospores: dormant, highly resistant structures formed by some bacteria under stress - Not reproductive structures; help bacterium survive extreme conditions - Sporulation: triggered by nutrient depletion or harsh env conditions - Survival Mechanism: endospore can survive extreme heat, radiation and desiccation - Key features: - Thick protective layer - Dehydrated core (resistant to heat and radiation) - Small Acid-soluble proteins: protect DNA from damage - Dipicolinic acid and Ca^2+^: stabilize proteins and DNA - Alternative Cell Differentiation Strategies: - Myxospores: differentiation strategy used by Myxobacteria to form multicellular fruiting bodies - Gram -ve bacteria has multi-cellular lifecycle - Active bacteria outside and inside bacteria is resistant - Heterocyst: specialized cells in cyanobacteria that fix nitrogen in low-nitrogen env - The cyanobacterium Anabaena. The expression of genes in heterocyst is different from their expression in other cells. All cells in the figure contain a cyanobacterial gene to which the gene for green fluorescent protein (GFP) has been spliced. Only cells that have formed heterocyst are expressing the fused gene, which makes the heterocyst cell fluoresce bright green - Thick cell wall to limit oxygen diffusion. Cells do not divide or reproduce - Survival: These differentiation strategies allow bacteria to survive in extreme conditions - Stalked and swarmer cells: - Swamer cells: motile (flagella) involved in dispersal - Stalked cell: non-motile; attached by stalk; involved in nutrient acquisition and reproduction - Persister Cells: - metabolically dormant variants of regular cells that are highly tolerant to antibiotics - temporarily hault growth to survive - Microbial Growth in Nature: - Environmental Conditions: microbial growth in nature is influenced by factors like nutrient availability, temperature and competition - Biofilms and Aggregates: most microbes grow in biofilms or as part of larger microbial communities (nutrient hot spot) - Adaptation to stress: in nature, microbes face frequent stress such as limited nutrients or exposure to toxins **[Chapter 5: Environmental Influences and Control of Microbial Growth]** - Niche: - Multi-dimensional; abiotic environmental conditions an organism can grow in - Two organisms cannot occupy one niche (competitive exclusion principle)  - Bacteria vs Fungi: - Water - F: terrestrial; can be drought tolerant - B: aquatic; vulnerable to drought - Eukaryotes (protists): prone to drying (most are aquatic) - Temperature: - F: tend to have less adaptations to extreme temps - B: can adapt to most temp (present in both tropical and desert biomes) - Environmental Limits on Growth: - Microbial growth in influenced by environmental factors such as temperature, pressure and osmolarity - Different microbes have evolved to thrive in specific environmental conditions - Studying these organism provide insights into microbial survival strategies - Cost of specialist compared to generalist: can't do well in non-optimum conditions - Temperature and Microbial Growth: - Classifications - Hyperthermophiles: optimal growth above 80°C - Thermophiles: grow between 50°C-80°C - Mesophiles: between 15°C-45°C - Psychrophiles: below 15°C - Temperature extremes require specific adaptations like protein stability - Temp effects enzyme activity and membrane fluidity - Temp increases fluidity increases; compensation: change in comp. of lipids (increase saturation of lipids) - Growth rate increases linearly with temp up to a point - Deviation from the optimal temperature rapidly decreases growth rate - Arrhenius equation models temp effects on microbial growth rate - Predicts growth rate as function of temperature (at moderate ranges of temp only) - At extreme temps, mech allow them to deviate from predictions - Pressure and Barophiles: - Barophiles thrive at pressure greater than 380atm (deep sea env) - Extreme pressure influences cell membrane integrity and enzyme function - Membrane fluidity decreases as pressure increases - Mariana Trench as example of high-pressure environments - Deepest part of ocean. Pressure (110MPa) 1000x greater than land pressure (0.1MPa) - Osmolarity and Halophiles: - Halophiles require high salt concentrations (above 2M NaCl) for growth - Halotolerant organisms can survive both high and low salt environments - Osmotic stress (imbalance in salt cont. in and out of cell) is managed through aquaporins and compatible solutes - Accumulate compatible salt (K+ or organic mol) within cell to maintain balance with external salt conditions (balance osmotic pressure and retain water) - Aquaporins: - These proteins allow cells to rapidly adjust to changes in surrounding osmolarity, maintaining cell integrity - pH and Microbial Growth: - Classification: - Alkaliphiles: thrive in env with pH\>9 - Neutrophiles: between pH 5-8 - Acidophiles: prefer pH \