Revised Chapter 3 The Cell PDF
Document Details
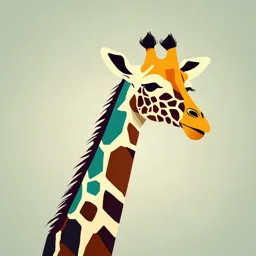
Uploaded by RoomierCosmos3570
CUNY Queensborough Community College
Tags
Summary
This document is a PowerPoint presentation on Chapter 3 The Cell, which covers important topics within microbiology and cell structures. It includes illustrations of cells, figures and some summaries.
Full Transcript
MICROBIOLOGY Chapter 3 THE CELL PowerPoint Image Slideshow FIGURE 3.1 Microorganisms vary visually in their size and shape, as can be observed microscopically; but they also vary in invisible ways, such as in their metabolic capabilities. (credit a, e, f: modification of work by Centers for Dise...
MICROBIOLOGY Chapter 3 THE CELL PowerPoint Image Slideshow FIGURE 3.1 Microorganisms vary visually in their size and shape, as can be observed microscopically; but they also vary in invisible ways, such as in their metabolic capabilities. (credit a, e, f: modification of work by Centers for Disease Control and Prevention; credit b: modification of work by NIAID; credit c: modification of work by CSIRO; credit d: modification of work by “Microscopic World”/YouTube) FIGURE 3.2 Francesco Redi’s experimental setup consisted of an open container, a container sealed with a cork top, and a container covered in mesh that let in air but not flies. Maggots only appeared on the meat in the open container. However, maggots were also found on the gauze of the gauze-covered container. FIGURE 3.3 (a) Francesco Redi, who demonstrated that maggots were the offspring of flies, not products of spontaneous generation. (b) John Needham, who argued that microbes arose spontaneously in broth from a “life force.” (c) Lazzaro Spallanzani, whose experiments with broth aimed to disprove those of Needham. FIGURE 3.4 (a) French scientist Louis Pasteur, who definitively refuted the long-disputed theory of spontaneous generation. (b) The unique swan-neck feature of the flasks used in Pasteur’s experiment allowed air to enter the flask but prevented the entry of bacterial and fungal spores. (c) Pasteur’s experiment consisted of two parts. In the first part, the broth in the flask was boiled to sterilize it. When this broth was cooled, it remained free of contamination. In the second part of the experiment, the flask was boiled and then the neck was broken off. The broth in this flask became contaminated. (credit b: modification of work by “Wellcome Images”/Wikimedia Commons) FIGURE 3.5 Robert Hooke (1635–1703) was the first to describe cells based upon his microscopic observations of cork. This illustration was published in his work Micrographia. Summary: Spontaneous Generation The theory of spontaneous generation states that life arose from nonliving matter. It was a long-held belief dating back to Aristotle and the ancient Greeks. Experimentation by Francesco Redi in the 17th century presented the first significant evidence refuting spontaneous generation by showing that flies must have access to meat for maggots to develop on the meat. Prominent scientists designed experiments and argued both in support of (John Needham) and against (Lazzaro Spallanzani) spontaneous generation. Louis Pasteur is credited with conclusively disproving the theory of spontaneous generation with his famous swan-neck flask experiment. He subsequently proposed that “life only comes from life”. FIGURE 3.6 (a) Rudolf Virchow (1821–1902) popularized the cell theory in an 1855 essay entitled “Cellular Pathology.” (b) The idea that all cells originate from other cells was first published in 1852 by his contemporary and former colleague Robert Remak (1815–1865). FIGURE 3.7 According to the endosymbiotic theory, mitochondria and chloroplasts are each derived from the uptake of bacteria. These bacteria established a symbiotic relationship with their host cell that eventually led to the bacteria evolving into mitochondria and chloroplasts. FIGURE 3.8 Ignaz Semmelweis (1818–1865) was a proponent of the importance of handwashing to prevent transfer of disease between patients by physicians. FIGURE 3.9 (a) Joseph Lister developed procedures for the proper care of surgical wounds and the sterilization of surgical equipment. (b) Robert Koch established a protocol to determine the cause of infectious disease. Both scientists contributed significantly to the acceptance of the germ theory of disease. FIGURE 3.11 (credit “swan-neck flask”: modification of work by Wellcome Images) Summary: Foundations of Modern Cell Theory Although cells were first observed in the 1660’s by Robert Hooke, cell theory was not well accepted for another 200 years. The work of scientists such as Schleiden, Schwann, Remak and Virchow contributed to its acceptance. Endosymbiotic theory states that mitochondria and chloroplasts, organelles found in many types of organisms, have their origin in bacteria. Significant structural and genetic information support this theory. The miasma theory of disease was widely accepted until the 19th century, when it was replaced by the germ theory of disease thanks to the work of Semmelweis, Snow, Pasteur, Lister and Koch, and others. FIGURE 3.12 A typical prokaryotic cell contains a cell membrane, chromosomal DNA that is concentrated in a nucleoid, ribosomes, and a cell wall. Some prokaryotic cells may also possess flagella, pili, fimbriae, and capsules. FIGURE 3.13 (credit “Coccus” micrograph: modification of work by Janice Haney Carr, Centers for Disease Control and Prevention; credit “Coccobacillus” micrograph: modification of work by Janice Carr, Centers for Disease Control and Prevention; credit “Spirochete” micrograph: modification of work by Centers for Disease Control and Prevention) FIGURE 3.14 FIGURE 3.15 In cells that lack a cell wall, changes in osmotic pressure can lead to crenation in hypertonic environments or cell lysis in hypotonic environments. FIGURE 3.16 In prokaryotic cells, the cell wall provides some protection against changes in osmotic pressure, allowing it to maintain its shape longer. The cell membrane is typically attached to the cell wall in an isotonic medium (left). In a hypertonic medium, the cell membrane detaches from the cell wall and contracts (plasmolysis) as water leaves the cell. In a hypotonic medium (right), the cell wall prevents the cell membrane from expanding to the point of bursting, although lysis will eventually occur if too much water is absorbed. FIGURE 3.17 The nucleoid region (the area enclosed by the green dashed line) is a condensed area of DNA found within prokaryotic cells. Because of the density of the area, it does not readily stain and appears lighter in color when viewed with a transmission electron microscope. FIGURE 3.18 Prokaryotic ribosomes (70S) are composed of two subunits: the 30S (small subunit) and the 50S (large subunit), each of which are composed of protein and rRNA components. FIGURE 3.19 Prokaryotic cells may have various types of inclusions. (a) A transmission electron micrograph of polyhydroxybutryrate lipid droplets. (b) A light micrograph of volutin granules. (c) A phase-contrast micrograph of sulfur granules. (d) A transmission electron micrograph of magnetosomes. (e) A transmission electron micrograph of gas vacuoles. (credit b, c, d: modification of work by American Society for Microbiology) FIGURE 3.20 (a) Sporulation begins following asymmetric cell division. The forespore becomes surrounded by a double layer of membrane, a cortex, and a protein spore coat, before being released as a mature endospore upon disintegration of the mother cell. (b) An electron micrograph of a Carboxydothermus hydrogenoformans endospore. (c) These Bacillus spp. cells are undergoing sporulation. The endospores have been visualized using Malachite Green spore stain. (credit b: modification of work by Jonathan Eisen) FIGURE 3.21 The bacterial plasma membrane is a phospholipid bilayer with a variety of embedded proteins that perform various functions for the cell. Note the presence of glycoproteins and glycolipids, whose carbohydrate components extend out from the surface of the cell. The abundance and arrangement of these proteins and lipids can vary greatly between species. FIGURE 3.22 Simple diffusion down a concentration gradient directly across the phospholipid bilayer. (credit: modification of work by Mariana Ruiz Villareal) FIGURE 3.23 Facilitated diffusion down a concentration gradient through a membrane protein. (credit: modification of work by Mariana Ruiz Villareal) FIGURE 3.24 Active transport against a concentration gradient via a membrane pump that requires energy. (credit: modification of work by Mariana Ruiz Villareal) FIGURE 3.25 Peptidoglycan is composed of polymers of alternating NAM and NAG subunits, which are cross-linked by peptide bridges linking NAM subunits from various glycan chains. This provides the cell wall with tensile strength in two dimensions. FIGURE 3.26 Bacteria contain two common cell wall structural types. Gram-positive cell walls are structurally simple, containing a thick layer of peptidoglycan with embedded teichoic acid external to the plasma membrane. Gram-negative cell walls are structurally more complex, containing three layers: the inner membrane, a thin layer of peptidoglycan, and an outer membrane containing lipopolysaccharide. (credit: modification of work by “Franciscosp2”/Wikimedia Commons) FIGURE 3.27 (a) Some gram-positive bacteria, including members of the Mycobacteriaceae, produce waxy mycolic acids found exterior to their structurally-distinct peptidoglycan. (b) The acid-fast staining protocol detects the presence of cell walls that are rich in mycolic acid. Acid- fast cells are stained red by carbolfuschin. (credit a: modification of work by “Franciscosp2”/Wikimedia Commons; credit b: modification of work by Centers for Disease Control and Prevention) FIGURE 3.28 The outer membrane of a gram-negative bacterial cell contains lipopolysaccharide (LPS), a toxin composed of Lipid A embedded in the outer membrane, a core polysaccharide, and the O side chain. FIGURE 3.29 (a) Capsules are a type of glycocalyx composed of an organized layer of polysaccharides. (b) A capsule stain of Pseudomonas aeruginosa, a bacterial pathogen capable of causing many different types of infections in humans. (credit b: modification of work by American Society for Microbiology) FIGURE 3.30 Bacteria may produce two different types of protein appendages that aid in surface attachment. Fimbriae typically are more numerous and shorter, whereas pili (shown here) are longer and less numerous per cell. (credit: modification of work by American Society for Microbiology) FIGURE 3.31 The basic structure of a bacterial flagellum consists of a basal body, hook, and filament. The basal body composition and arrangement differ between gram-positive and gram- negative bacteria. (credit: modification of work by “LadyofHats”/Mariana Ruiz Villareal) FIGURE 3.32 Flagellated bacteria may exhibit multiple arrangements of their flagella. Common arrangements include monotrichous, amphitrichous, lophotrichous, or peritrichous. FIGURE 3.33 Bacteria achieve directional movement by changing the rotation of their flagella. In a cell with peritrichous flagella, the flagella bundle when they rotate in a counterclockwise direction, resulting in a run. However, when the flagella rotate in a clockwise direction, the flagella are no longer bundled, resulting in tumbles. FIGURE 3.34 Without a chemical gradient, flagellar rotation cycles between counterclockwise (run) and clockwise (tumble) with no overall directional movement. However, when a chemical gradient of an attractant exists, the length of runs is extended, while the length of tumbles is decreased. This leads to chemotaxis: an overall directional movement toward the higher concentration of the attractant. Summary: Unique Characteristics of Prokaryotic Cells Prokaryotic cells differ from eukaryotic cells in that their genetic material is contained in a nucleoid rather than a membrane-bound nucleus. In addition, prokaryotic cells generally lack membrane-bound organelles. Prokaryotic cells of the same species typically share a similar cell morphology and cellular arrangement. Most prokaryotic cells have a cell wall that helps the organism maintain cellular morphology and protects it against changes in osmotic pressure. Outside of the nucleoid, prokaryotic cells may contain extrachromosomal DNA in plasmids. Prokaryotic ribosomes that are found in the cytoplasm have a size of 70S. Some prokaryotic cells have inclusions that store nutrients or chemicals for other uses. Some prokaryotic cells are able to form endospores through sporulation to survive in a dormant state when conditions are unfavorable. Endospores can germinate, transforming back into vegetative cells when conditions improve. In prokaryotic cells, the cell envelope includes a plasma membrane and usually a cell wall. Bacterial membranes are composed of phospholipids with integral or peripheral proteins. The fatty acid components of these phospholipids are ester-linked and are often used to identify specific types of bacteria. The proteins serve a variety of functions, including transport, cell-to-cell communication, and sensing environmental conditions. Archaeal membranes are distinct in that they are composed of fatty acids that are ether-linked to phospholipids. Some molecules can move across the bacterial membrane by simple diffusion, but most large molecules must be actively transported through membrane structures using cellular energy. Prokaryotic cells walls may be composed of peptidoglycan (bacteria) or pseudopeptidoglycan (archaea). Gram-positive cell walls are characterized by a thick peptidoglycan layer, whereas gram-negative bacterial cells are characterized by a thin peptidoglycan layer surrounded by an outer membrane. Some bacterial cells produce glycocalyx coatings, such as capsules and slime layers, that aid in attachment to surfaces and/or evasion of the host immune response. Some prokaryotic cells have fimbriae or pili, filamentous appendages that aid in attachment to surfaces. Pili are also used in the transfer of genetic material between cells. Some prokaryotic cells use one or more flagella to move through water. Peritrichous bacteria, which have numerous flagella, use runs and tumbles to move purposefully in the direction of a chemical attractant. FIGURE 3.35 An illustration of a generalized, single-celled eukaryotic organism. Note that cells of eukaryotic organisms vary greatly in terms of structure and function, and a particular cell may not have all of the structures shown here. FIGURE 3.36 Eukaryotic cells come in a variety of cell shapes. (a) Spheroid Chromulina alga. (b) Fusiform shaped Trypanosoma. (c) Bell-shaped Vorticella. (d) Ovoid Paramecium. (e) Ring-shaped Plasmodium ovale. (credit a: modification of work by NOAA; credit b, e: modification of work by Centers for Disease Control and Prevention) FIGURE 3.37 Eukaryotic cells have a well-defined nucleus. The nucleus of this mammalian lung cell is the large, dark, oval-shaped structure in the lower half of the image. FIGURE 3.38 In this fluorescent microscope image, all the intermediate filaments have been stained with a bright green fluorescent stain. The nuclear lamina is the intense bright green ring around the faint red nuclei. FIGURE 3.39 (a) The nucleolus is the dark, dense area within the nucleus. It is the site of rRNA synthesis and preribosomal assembly. (b) Electron micrograph showing the nucleolus. FIGURE 3.40 The endomembrane system is composed of a series of membranous intracellular structures that facilitate movement of materials throughout the cell and to the cell membrane. FIGURE 3.41 The rough endoplasmic reticulum is studded with ribosomes for the synthesis of membrane proteins (which give it its rough appearance). FIGURE 3.42 A transmission electron micrograph (left) of a Golgi apparatus in a white blood cell. The illustration (right) shows the cup-shaped, stacked disks and several transport vesicles. The Golgi apparatus modifies lipids and proteins, producing glycolipids and glycoproteins, respectively, which are commonly inserted into the plasma membrane. FIGURE 3.43 A transmission electron micrograph (left) of a cell containing a peroxisome. The illustration (right) shows the location of peroxisomes in a cell. These eukaryotic structures play a role in lipid biosynthesis and breaking down various molecules. They may also have other specialized functions depending on the cell type. (credit “micrograph”: modification of work by American Society for Microbiology) FIGURE 3.44 The cytoskeleton is a network of microfilaments, intermediate filaments, and microtubules found throughout the cytoplasm of a eukaryotic cell. In these fluorescently labeled animal cells, the microtubules are green, the actin microfilaments are red, the nucleus is blue, and keratin (a type of intermediate filament) is yellow. FIGURE 3.45 (a) A microfilament is composed of a pair of actin filaments. (b) Each actin filament is a string of polymerized actin monomers. (c) The dynamic nature of actin, due to its polymerization and depolymerization and its association with myosin, allows microfilaments to be involved in a variety of cellular processes, including ameboid movement, cytoplasmic streaming, contractile ring formation during cell division, and muscle contraction in animals. FIGURE 3.46 (a) Intermediate filaments are composed of multiple strands of polymerized subunits. They are more permanent than other cytoskeletal structures and serve a variety of functions. (b) Intermediate filaments form much of the nuclear lamina. (c) Intermediate filaments form the desmosomes between cells in some animal tissues. (credit c “illustration”: modification of work by Mariana Ruiz Villareal) FIGURE 3.47 (a) Microtubules are hollow structures composed of polymerized tubulin dimers. (b) They are involved in several cellular processes, including the movement of organelles throughout the cytoplasm. Motor proteins carry organelles along microtubule tracks that crisscross the entire cell. (credit b: modification of work by National Institute on Aging) FIGURE 3.48 (a) A centrosome is composed of two centrioles positioned at right angles to each other. Each centriole is composed of nine triplets of microtubules held together by accessory proteins. (b) In animal cells, the centrosomes (arrows) serve as microtubule-organizing centers of the mitotic spindle during mitosis. FIGURE 3.49 Each mitochondrion is surrounded by two membranes, the inner of which is extensively folded into cristae and is the site of the intermembrane space. The mitochondrial matrix contains the mitochondrial DNA, ribosomes, and metabolic enzymes. The transmission electron micrograph of a mitochondrion, on the right, shows both membranes, including cristae and the mitochondrial matrix. (credit “micrograph”: modification of work by Matthew Britton; scale-bar data from Matt Russell) FIGURE 3.50 Photosynthesis takes place in chloroplasts, which have an outer membrane and an inner membrane. Stacks of thylakoids called grana form a third membrane layer. FIGURE 3.51 The eukaryotic plasma membrane is composed of a lipid bilayer with many embedded or associated proteins. It contains cholesterol for the maintenance of membrane, as well as glycoproteins and glycolipids that are important in the recognition other cells or pathogens. FIGURE 3.52 Three variations of endocytosis are shown. (a) In phagocytosis, the cell membrane surrounds the particle and pinches off to form an intracellular vacuole. (b) In pinocytosis, the cell membrane surrounds a small volume of fluid and pinches off, forming a vesicle. (c) In receptor-mediated endocytosis, the uptake of substances is targeted to a specific substance (a ligand) that binds at the receptor on the external cell membrane. (credit: modification of work by Mariana Ruiz Villarreal) FIGURE 3.53 The extracellular matrix is composed of protein and carbohydrate components. It protects cells from physical stresses and transmits signals arriving at the outside edges of the tissue to cells deeper within the tissue. FIGURE 3.54 (a) Eukaryotic flagella and cilia are composed of a 9+2 array of microtubules, as seen in this transmission electron micrograph cross-section. (b) The sliding of these microtubules relative to each other causes a flagellum to bend. (c) An illustration of Trichomonas vaginalis, a flagellated protozoan parasite that causes vaginitis. (d) Many protozoans, like this Paramecium, have numerous cilia that aid in locomotion as well as in feeding. Note the mouth opening shown here. (credit d: modification of work by University of Vermont/National Institutes of Health) Summary: Unique Characteristics of Eukaryotic Cells Eukaryotic cells are defined by the presence of a nucleus containing the DNA genome and bound by a nuclear membrane (or nuclear envelope) composed of two lipid bilayers that regulate transport of materials into and out of the nucleus through nuclear pores. Eukaryotic cell morphologies vary greatly and may be maintained by various structures, including the cytoskeleton, the cell membrane, and/or a cell wall. The nucleolus, located in the nucleus of eukaryotic cells, is the site of ribosomal synthesis and the first stages of ribosome assembly. Eukaryotic cells contain 80S ribosomes in the rough endoplasmic reticulum (membrane-bound ribosomes) and cytoplasm (free ribosomes). They contain 70s ribosomes in mitochondria and chloroplasts. Eukaryotic cells have evolved a endomembrane system, containing membrane-bound organelles involved in transport. These include vesicles, the endoplasmic reticulum, and the Golgi apparatus. The smooth endoplasmic reticulum plays a role in lipid biosynthesis, carbohydrate metabolism, and detoxification of toxic compounds. The rough endoplasmic reticulum contains membrane-bound 80S ribosomes that synthesize proteins destined for the cell membrane. The Golgi apparatus processes proteins and lipids, typically through the addition of sugar molecules producing glycoproteins or glycolipids, component of the plasma membrane that are used in cell-to-cell communication. Lysosomes contain digestive enzymes that break down small particles ingested by endocytosis, large particles that are ingested by phagocytosis, and damaged intracellular components. The cytoskeleton, composed of microfilaments, intermediate filaments, and microtubules, provides structural support in eukaryotic cells and serves as a network for transport of intracellular materials. Centrosomes are microtubule-organizing centers important in the formation of the mitotic spindle in mitosis. Mitochondria are the site of cellular respiration. They have two membranes: an outer membrane and an inner membrane with cristae. The mitochondrial matrix, within the inner membrane, contains the mitochondrial DNA, 70S ribosomes, and metabolic enzymes. The plasma membrane of eukaryotic cells is structurally similar to that found in prokaryotic cells, and membrane components move according to the fluid mosaic model. However, eukaryotic membranes contain sterols, which alter membrane fluidity, as well as glycoproteins and glycolipids, which help the cell recognize other cells and infectious particles. In addition to active transport and passive transport, eukaryotic cell membranes can take material into the cell via endocytosis, or expel matter from the cell via exocytosis. Cells of fungi, algae, plants, and some protists have cell walls, whereas cells of animals and some protozoans have a sticky extracellular matrix that provides structural support and mediates cellular signaling. Eukaryotic flagella are structurally distinct from prokaryotic flagella but serve a similar purpose (locomotion). Cilia are structurally similar to eukaryotic flagella, but shorter; they may be used for locomotion, feeding, or movement of extracellular particles. This file is copyright 2016, Rice University. All Rights Reserved.