respiratory_physiology_lfc.ppt
Document Details
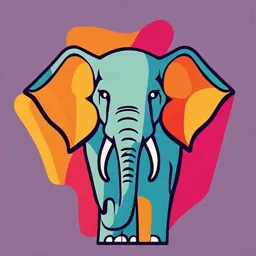
Uploaded by AchievableSalamander
Full Transcript
1 RESPIRATORY PHYSIOLOGY Dr. Samantha Solecki, DC, MS Instructor, Biology Thinker . Learner . Motivator . Lover of Anatomy & Physiology [email protected] © 2019 Pearson Education, Inc. 2 Learning Objectives *Acquired from the Human Anatomy and Physiology Society (HAPS) with personal addit...
1 RESPIRATORY PHYSIOLOGY Dr. Samantha Solecki, DC, MS Instructor, Biology Thinker . Learner . Motivator . Lover of Anatomy & Physiology [email protected] © 2019 Pearson Education, Inc. 2 Learning Objectives *Acquired from the Human Anatomy and Physiology Society (HAPS) with personal additions • Describe the four respiratory processes – ventilation, external respiration, internal • • • • • • • • • • respiration and cellular respiration. Define pulmonary ventilation, inspiration and expiration. Identify the muscles used during quiet inspiration, during forced inspiration and during forced expiration as well as the nerves responsible for stimulating those muscles. Define and state relative values for atmospheric pressure, intrapulmonary pressure, intrapleural pressure and transpulmonary pressure. State Boyle’s law and relate this law to the specific sequence of events (muscle contractions/relaxations and pressure/volume changes) causing inspiration and expiration. Explain how each of the following affect pulmonary ventilation: bronchiolar smooth muscle contractions, lung and thoracic wall compliance and recoil and pulmonary surfactant and alveolar surface tension. Describe the forces that tend to collapse the lungs and those that normally oppose or prevent collapse. Define, identify and determine values for the respiratory volumes (IRV, TV, ERV and RV) and the respiratory capacities (IC, FRC, VC and TLC). Define and calculate values for minute ventilation and alveolar ventilation. Define anatomical dead space and explain the effect of anatomical dead space on alveolar ventilation and on the composition of alveolar and expired air. State Dalton’s Law and Henry’s Law and relate both laws to the events of external and internal respiration and to the amounts of oxygen and carbon dioxide dissolved in plasma. 3 Learning Objectives *Acquired from the Human Anatomy and Physiology Society (HAPS) with personal additions • With respect to external respiration: Describe oxygen and carbon dioxide concentration gradients and net gas movements. Analyze how oxygen and carbon dioxide movements are affected by changes in partial pressure gradients (e.g., at high altitude), surface area, diffusion distance and solubility and molecular weight of the gases. • Describe the mechanisms of ventilation-perfusion coupling and predict the effect that reduced alveolar ventilation has on pulmonary blood flow and the effect that reduced pulmonary blood flow has on bronchiole diameter and alveolar ventilation. • • • With respect to internal respiration: • Describe oxygen and carbon dioxide concentration gradients and net gas movements. • Explain the factors that maintain oxygen and carbon dioxide gradients between blood and tissue cells. • With respect to oxygen transport: Describe the ways in which oxygen is transported in blood and discuss the relative importance of each to total oxygen transport. • State the reversible chemical equation for oxygen binding to hemoglobin and predict how raising or lowering the partial pressure of oxygen will shift the equilibrium. • • With respect to the oxygen-hemoglobin saturation curve: • Interpret the curve at low and high partial pressures of oxygen. • List factors that shift the curve down and to the right and explain how this results in increased oxygen delivery to tissues. • List factors that shift the curve up and to the left and explain how this facilitates oxygen binding to hemoglobin in the lungs. 4 Learning Objectives *Acquired from the Human Anatomy and Physiology Society (HAPS) with personal additions • With respect to carbon dioxide transport: • Describe the ways in which carbon dioxide is transported in blood and discuss the relative importance of each to total carbon dioxide transport. • State the reversible chemical equation for the reaction of carbon dioxide and water to carbonic acid and then to hydrogen ion bicarbonate ion. • Explain the relationship between pH and hydrogen ion concentration. • Predict how changing the partial pressure of carbon dioxide will affect the pH and the concentration of bicarbonate ions in the plasma. • Predict how changing the pH or the concentration of bicarbonate ions will affect the partial pressure of carbon dioxide in the plasma. • State the reversible chemical equation for carbon dioxide binding to deoxyhemoglobin and predict how changing carbon dioxide concentrations will affect deoxyhemoglobin levels in the tissues and the lungs. • Explain how each of the following relates to carbon dioxide transport: carbonic anhydrase, hydrogen ions binding to hemoglobin and plasma proteins, the chloride ion shift, and the oxygenhemoglobin saturation level. • Explain how the respiratory system relates to other body systems to maintain homeostasis. • Predict factors or situations affecting the respiratory system that could disrupt homeostasis. 5 Processes of Respiration • Pulmonary ventilation (breathing)- movement of air into and out Respiratory of lungs • Functions to exchange and change gases system • External respiration-O2 and CO2 exchange between lungs and blood • Transport of Gases-O2 and CO2 in blood • Internal respiration-O2 and CO2 exchange between systemic blood vessels and tissues Circulatory system 6 Respiratory System Figure 22.1 Respiration consists of four processes. Copyright © 2019, 2016, 2013 Pearson Education, Inc. All Rights Reserved 7 The Major Respiratory Organs in Relation to Surrounding Structures Figure 22.2 The major respiratory organs in relation to surrounding structures. Copyright © 2019, 2016, 2013 Pearson Education, Inc. All Rights Reserved Alveoli and the Respiratory Membrane 8 Figure 22.11a Alveoli and the respiratory membrane. Copyright © 2019, 2016, 2013 Pearson Education, Inc. All Rights Reserved Alveoli and the Respiratory Membrane 9 Figure 22.11c Alveoli and the respiratory membrane. Copyright © 2019, 2016, 2013 Pearson Education, Inc. All Rights Reserved 10 BREATHING MECHANICS 11 Mechanics of Breathing • Pulmonary ventilation = breathing • Pulmonary ventilation consists of two phases • Inspiration-gases flow into lungs • Expiration-gases exit lungs 12 Pressure Relationships in the Thoracic Cavity • Atmospheric pressure (Patm) • Pressure exerted by air surrounding body • = 1 atmosphere • Respiratory pressures described relative to Patm • Negative respiratory pressure = less than Patm • Positive respiratory pressure = greater than Patm • Zero respiratory pressure = Patm 13 Intrapulmonary Pressure • Intrapulmonary (intra-alveolar) pressure (Ppul) • Pressure in alveoli • Fluctuates with breathing (, ) • Always eventually equalizes with Patm 14 Intrapleural Pressure • Intrapleural pressure (Pip) • Pressure in pleural cavity • Fluctuates with breathing/Ppul • Always a negative pressure (<Patm and <Ppul) • Fluid level must be minimal • Pumped out by lymphatics • If accumulates positive Pip pressure lung collapse (Atelectasis) 15 Intrapleural Pressure • Negative Pip caused by opposing forces • Two inward forces promote lung collapse • Elastic recoil of lungs decreases lung size • Surface tension of alveolar fluid reduces alveolar size • Surface tension constantly draws inward • One outward force tends to enlarge lungs • Elasticity of chest wall pulls thorax outward • Net result of these opposing forces keeps the lungs inflated • Pleural fluid must be minimal to help keep the Pip negative AND intrapleural space must ave a net negtive pressure 16 Pressure Relationships • If Pip = Ppul or Patm lungs collapse • (Ppul – Pip) = transpulmonary pressure • Keeps airways open • Greater transpulmonary pressure larger lungs Figure 22.12 Intrapulmonary and intrapleural pressure relationships. 17 Atmospheric pressure (Patm) 0 mm Hg (760 mm Hg) Parietal pleura Thoracic wall Visceral pleura Pleural cavity Transpulmonary pressure 4 mm Hg (the difference between 0 mm Hg and −4 mm Hg) –4 0 Lung Diaphragm Intrapulmonary pressure (Ppul) 0 mm Hg (760 mm Hg) Intrapleural pressure (Pip) −4 mm Hg (756 mm Hg) Pneumothorax Figure 22.15 Pneumothorax. 19 Pulmonary Ventilation • Inspiration and expiration • Mechanical processes that depend on volume changes in thoracic cavity • Volume changes pressure changes • Pressure changes gases flow to equalize pressure 20 Boyle's Law • Relationship between pressure and volume of a gas • Gases fill container; if container size reduced increased pressure • Pressure (P) varies inversely with volume (V): P1V1 = P2V2 21 Inspiration • Active process • Inspiratory muscles contract (diaphragm (flatterns and moves inferiorly) and external intercostals (lifts rib cage and pulls the sternum superiorly)) • Thoracic volume increases (500 mL) intrapulmonary pressure drops (to 1 mm Hg) • Lungs stretched and intrapulmonary volume increases • When Ppul < Patm Air flows into lungs, down its pressure gradient, until Ppul = Patm • At this time, Pip drop 6 mmHg below Patm Figure 22.13 Changes in thoracic volume and sequence of events during inspiration and expiration. (1 of 2) Sequence of events Changes in anterior-posterior and superior-inferior dimensions 22 Changes in lateral dimensions (superior view) Inspiratory muscles 1 contract (diaphragm descends; rib cage rises). Inspiration Thoracic cavity volume 2 increases. 3 Lungs are stretched; intrapulmonary volume increases. Ribs are elevated and sternum flares as external intercostals contract. External intercostals contract. 4 Intrapulmonary pressure drops (to –1 mm Hg). Air 5 (gases) flows into lungs down its pressure gradient until intrapulmonary pressure is 0 (equal to atmospheric pressure). Diaphragm moves inferiorly during contraction. Slide 1 23 Expiration • Quiet expiration normally passive process • Inspiratory muscles relax • Thoracic cavity volume decreases • Elastic lungs recoil and intrapulmonary volume decreases pressure increases (Ppul rises to +1 mm Hg) • Air flows out of lungs down its pressure gradient until Ppul = 0 • Note: forced expiration-active process; uses abdominal (oblique and transverse) and internal intercostal muscles • intraabdominal pressure • rib cage Figure 22.13 Changes in thoracic volume and sequence of events during inspiration and expiration. (2 of 2) Sequence of events Changes in anterior-posterior and superior-inferior dimensions 24 Changes in lateral dimensions (superior view) 1 Inspiratory muscles relax (diaphragm rises; rib cage descends due to recoil of costal cartilages). Expiration Thoracic cavity volume 2 decreases. Elastic lungs recoil 3 passively; intrapulmonary Volume decreases. Ribs and sternum are depressed as external intercostals relax. External intercostals relax. Intrapulmonary pressure 4 rises (to +1 mm Hg). 5 (gases) flows out of Air lungs down its pressure gradient until intrapulmonary pressure is 0. Diaphragm moves superiorly as it relaxes. Slide 1 Intrapleural pressure. Pleural cavity pressure becomes more negative as chest wall expands during inspiration. Returns to initial value as chest wall recoils. Volume of breath. During each breath, the pressure gradients move 0.5 liter of air into and out of the lungs. Inspiration +2 Expiration Intrapulmonary pressure 0 –2 –4 –6 –8 Volume (L) Intrapulmonary pressure. Pressure inside lung decreases as lung volume increases during inspiration; pressure increases during expiration. Pressure relative to atmospheric pressure (mm Hg) Figure 22.14 Changes in intrapulmonary and intrapleural pressures during inspiration and expiration. Transpulmonary pressure Intrapleural pressure Volume of breath 0.5 0 5 seconds elapsed 25 26 Pulmonary Ventilation • Nonrespiratory air movements • Many processes can move air into or out of lungs besides breathing • May modify normal respiratory rhythm • Most result from reflex action, although some are voluntary • Examples: coughing, sneezing, crying, laughing, hiccups, and yawns 27 Review... • List the three pressures involved in keeping the lungs inflated. Explain why these pressures exist. • Explain why inspiration is an active process and expiration is a passive process. 28 Physical Factors Influencing Pulmonary Ventilation • Three physical factors influence the ease of air passage and the amount of energy required for ventilation. • Airway resistance • Alveolar surface tension • Lung compliance 29 Airway Resistance • Friction (drag) is the major nonelastic source of resistance to gas flow; occurs in airways • Relationship between flow (F), pressure (P), and resistance (R) is: • ∆P - pressure gradient between atmosphere and alveoli (2 mm Hg or less during normal quiet breathing) • Gas flow changes inversely with resistance 30 Airway Resistance • Resistance usually insignificant • Large airway diameters in first part of conducting zone • Progressive branching of airways as get smaller, increasing total cross-sectional area • Resistance greatest in medium-sized bronchi • Resistance disappears at terminal bronchioles where diffusion drives gas movement Figure 22.15 Resistance in respiratory passageways. Conducting zone Respiratory zone Resistance Medium-sized bronchi Terminal bronchioles 1 5 10 15 Airway generation (stage of branching) 20 23 31 32 Alveolar Surface Tension • Surface tension • Attracts liquid molecules to one another at gas-liquid interface • Resists any force that tends to increase surface area of liquid • Water (polar) has a high surface tension; coats alveolar walls reduces them to smallest size • Surfactant (by Type II Alveolar cells) decreases the pull of water molecules, helping to prevent collapse 33 Lung Compliance • High compliance = efficient ventilation • Measure of change in lung volume that occurs with given change in transpulmonary pressure • Higher lung compliance easier to expand lungs • Normally high due to • Distensibility of lung tissue • Surfactant, which decreases alveolar surface tension 34 Physical Factors Influencing Pulmonary Ventilation • Lung compliance (cont.) • Can be written mathematically as: VL CL ( Ppul Pip ) • Where CL equals compliance, VL equals change in lung volume, and (Ppul Pip) equals change in transpulmonary pressure • Compliance can be diminished by: • Nonelastic scar tissue replacing lung tissue (fibrosis) • Reduced production of surfactant • Decreased flexibility of thoracic cage 35 ASSESSING VENTILATION 36 Respiratory Volumes • Tidal Volume (TV): Normal amount of air that moves into/out of the lungs during normal, quiet breathing (500mL) • Inspiratory reserve (IRV): amount of air that can be inspired forcibly beyond the TV (2100 – 3200mL) • Expiratory Reserve (ERV): amount of air that can be expelled from the lungs after the TV (1000 – 1200mL) • Residual Volume (RV): amount of air left over in the alveoli even after the TV and ERV; this prevents alveolar collapse (1200mL) 37 Respiratory Capacities • Inspiratory Capacity (IC): total amount of air that can inspired after a normal tidal volume expiration IC = TV + IRV • Functional Residual Capacity (FRC): the amount of air remaining in the lungs after a normal TV FRC = ERV + RV • Vital Capacity (VC): total amount of exchangeable air VC = TV + IRV + ERV • Total Lung Capacity (TLC): sum of all lung volumes (6000mL) TLC = TV + IRV + ERV + RV Figure 22.16a Respiratory volumes and capacities. 38 6000 Milliliters (ml) 5000 Inspiratory reserve volume 3100 ml 4000 Inspiratory capacity 3600 ml 3000 Tidal volume 500 ml 2000 1000 0 Spirographic record for a male Expiratory reserve volume 1200 ml Residual volume 1200 ml Functional residual capacity 2400 ml Vital capacity 4800 ml Total lung capacity 6000 ml 39 Dead Space • The anatomical dead space (ADS) is the space which contains volume of air left over in the conducting zone airways which do NOT contribute to gas exchange • One average ADS is about 150mL • Alveolar dead space also occurs, where some alveoli do not partake in gas exchange • Total Dead Space (TDS) = ADS + AlDS 40 Functional Tests • Spirometer • Used to measure loss in respiratory function • Original, cumbersome clinical tool used to measure patient’s respiratory volumes • Electronic measuring devices used today • Helpful in distinguishing between obstructive diseases and restrictive diseases of the lungs • Obstructive diseases: TLC, FRC and RV all • Restrictive diseases: VC, TLC, FRC and RV all • Forced Vital Capacity (FVC): measures the amount of gas expelled as deep inspiration and expiration occurs • Forced Expiratory Volume (FEV): measures the amount of air expelled in relation to time (1st second) 41 Review... • List the three factors involved in air flow. • Explain airway resistance. • Demonstrate the differences between Tidal Volume, Inspiratory Reserve Volume, Expiratory Reserve Volume & Residual Volume 42 GAS EXCHANGE & TRANSPORT 43 Basic Properties of Gases • External Respiration : oxygen enters/CO2 leaves the lungs via simple diffusion at the lungs • Influenced by • Thickness and surface area of respiratory membrane • Partial pressure gradients and gas solubilities • Ventilation-perfusion coupling • Internal Respiration: at the body tissues 44 BASIC PROPERTIES OF GASES • DALTON’S LAW : total pressure exerted by a mix of gases is the sum of the pressure exerted independently by each gas in the mix; the partial pressure is directly proportional to the % of gas in the mix • HENRY’S LAW: when a gas is in liquid the gas will dissolve in the liquid in proportion to its partial pressure • How much dissolves also depends on temperature and solubility 45 Basic Properties of Gases • Total atmospheric pressure equals 760 mm Hg • Nitrogen makes up ~78.6% of air; therefore, partial pressure of nitrogen, PN2, can be calculated: 0.786 760 mm Hg 597 mm Hg due to N2 • Oxygen makes up 20.9% of air, so PO2 equals: 0.209 760 mm Hg 159 mm Hg • Air also contains 0.04% CO2, 0.5% water vapor, and insignificant amounts of other gases • At high altitudes, partial pressures declines, but at lower altitudes (under water), partial pressures increase significantly 46 Comparison of Gas Partial Pressures and Approximate Percentages in the Atmosphere and in the Alveoli Table 22.4 Comparison of Gas Partial Pressures and Approximate Percentages in the Atmosphere and in the Alveoli. 47 Thickness and Surface Area of the Respiratory Membrane • Respiratory membranes • 0.5 to 1 m thick • Large total surface area (40 times that of skin) for efficient gas exchange • The greater the surface area, the better the gas exchange • Thicken if lungs become waterlogged and edematous gas exchange inadequate • Reduced surface area in emphysema (walls of adjacent alveoli break down), tumors, inflammation, mucus 48 Partial Pressure Gradients and Gas Solubilities • Steep partial pressure gradient for O2 in lungs helps drive diffusion of O2 and CO2 • Venous blood Po2 = 40 mm Hg • Alveolar Po2 = 104 mm Hg • Equilibrium reached across respiratory membrane in 0.25 seconds, about 1/3 time a red blood cell is in pulmonary capillary • Adequate oxygenation even if blood flow increases 3X Figure 22.18 Oxygenation of blood in the pulmonary capillaries at rest. 49 2 PO (mm Hg) 150 100 PO 104 mm Hg 2 50 40 0 0 Start of capillary 0.25 0.50 Time in the pulmonary capillary (s) 0.75 End of capillary 50 Partial Pressure Gradients and Gas Solubilities • Partial pressure gradient for CO2 in lungs less steep • Venous blood Pco2 = 45 mm Hg • Alveolar Pco2 = 40 mm Hg • Though gradient not as steep, CO2 diffuses in equal amounts with oxygen because CO2 is 20 times more soluble in plasma than oxygen Figure 22.17 Partial pressure gradients promoting gas movements in the body. Inspired air: PO2 160 mm Hg Alveoli of lungs: PO 104 mm Hg PCO 0.3 mm Hg PCO2 40 mm Hg 2 2 External respiration Pulmonary arteries Alveoli Pulmonary veins (PO 2 100 mm Hg) Blood leaving lungs and entering tissue capillaries: PO2 100 mm Hg PCO 40 mm Hg Blood leaving tissues and entering lungs: PO2 40 mm Hg PCO 45 mm Hg 2 2 Heart Systemic veins Systemic arteries Internal respiration Tissues: PO2 less than 40 mm Hg PCO greater than 45 mm Hg 2 51 52 Ventilation-Perfusion Coupling • Perfusion-blood flow reaching alveoli • Ventilation-amount of gas reaching alveoli • Ventilation and perfusion must match (couple) for efficient gas exchange • Never balanced for all alveoli due to • Regional variations due to effect of gravity on blood and air flow • Some alveolar ducts plugged with mucus 53 Ventilation-Perfusion Coupling • Both ventilation and perfusion are controlled but localized autoregulatory factors that are specialized to constantly respond to local conditions • Po2 controls perfusion by changing arteriolar diameter • PCO2 controls ventilation by changing bronchiolar diameter 54 Ventilation-Perfusion Coupling • Perfusion • Changes in Po2 in alveoli cause changes in diameters of arterioles • Where alveolar O2 is high, arterioles dilate • Where alveolar O2 is low, arterioles constrict • Directs most blood where alveolar oxygen high 55 Ventilation-Perfusion Coupling • Changes in Pco2 in alveoli cause changes in diameters of bronchioles • Where alveolar CO2 is high, bronchioles dilate • Where alveolar CO2 is low, bronchioles constrict • Allows elimination of CO2 more rapidly Figure 22.19 Ventilation-perfusion coupling. Ventilation less than perfusion 56 Ventilation greater than perfusion Mismatch of ventilation and perfusion ventilation and/or perfusion of alveoli causes local P CO2 and P O2 Mismatch of ventilation and perfusion ventilation and/or perfusion of alveoli causes local P CO2 and P O2 O2 autoregulates arteriolar diameter O2 autoregulates arteriolar diameter Pulmonary arterioles serving these alveoli constricts Pulmonary arterioles serving these alveoli dilate Match of ventilation and perfusion ventilation, perfusion Match of ventilation and perfusion ventilation, perfusion 57 Internal Respiration • Capillary gas exchange in body tissues • Partial pressures and diffusion gradients reversed compared to external respiration • Tissue Po2 always lower than in systemic arterial blood oxygen from blood to tissues • CO2 from tissues to blood • Venous blood Po2 40 mm Hg and Pco2 45 mm Hg Figure 22.17 Partial pressure gradients promoting gas movements in the body. Inspired air: PO2 160 mm Hg Alveoli of lungs: PO 104 mm Hg PCO 0.3 mm Hg PCO2 40 mm Hg 2 2 External respiration Pulmonary arteries Alveoli Pulmonary veins (PO 2 100 mm Hg) Blood leaving lungs and entering tissue capillaries: PO2 100 mm Hg PCO 40 mm Hg Blood leaving tissues and entering lungs: PO2 40 mm Hg PCO 45 mm Hg 2 2 Heart Systemic veins Systemic arteries Internal respiration Tissues: PO2 less than 40 mm Hg PCO greater than 45 mm Hg 2 58 59 TRANSPORT OF RESPIRATORY GASES BY BLOOD 60 O2 Transport • Molecular O2 carried in blood • 1.5% dissolved in plasma • 98.5% loosely bound to each Fe of hemoglobin (Hb) in RBCs • 4 O2 per Hb 61 O2 and Hemoglobin • Oxyhemoglobin (HbO2)-hemoglobin-O2 combination • Reduced hemoglobin (deoxyhemoglobin) (HHb)-hemoglobin that has released O2 62 O2 and Hemoglobin • Loading and unloading of O2 facilitated by change in shape of Hb • As O2 binds, Hb affinity for O2 increases • As O2 is released, Hb affinity for O2 decreases • Fully saturated (100%) if all four heme groups carry O2 • Partially saturated when one to three hemes carry O2 63 O2 and Hemoglobin • Rate of loading and unloading of O2 regulated to ensure adequate oxygen delivery to cells • Po2 • Temperature • Blood pH • Pco2 • Concentration of BPG–produced by RBCs during glycolysis; levels rise when oxygen levels chronically low 64 Influence of Po2 on Hemoglobin Saturation •Oxygen-hemoglobin dissociation curve •Demonstrates how local Po2 influences O2 loading and unloading from hemoglobin •Hemoglobin saturation plotted against Po2 not linear; S-shaped curve • Binding and release of O2 influenced by Po2 Figure 22.20 The amount of oxygen carried by hemoglobin depends on the P O2 (the amount of oxygen) available 65 locally. (1 of 3) In the lungs, where PO2 is high (100 mm Hg), Hb is almost fully saturated (98%) with O2. This axis tells you how much O2 is bound to Hb. At 100%, each Hb molecule has 4 bound oxygen molecules. Hemoglobin 100 • Oxygen If more O2 is present, more O2 is bound. However, because of Hb’s properties (O2 binding strength changes with saturation), this is an S-shaped curve, not a straight line. Percent O2 saturation of hemoglobin 80 60 40 20 • 0 0 20 40 60 80 100 P O2 (mm Hg) This axis tells you the relative Amount (partial pressure) of O2 disslolved in the fluid Surrounding the Hb. In the tissues of other organs, Where PO2 is low (40 mm Hg), Hb is less saturated (75%) with O2. Figure 22.20 The amount of oxygen carried by hemoglobin depends on the P O2 (the amount of oxygen) available 66 locally. (2 of 3) At sea level, there is lots of O2. In the lungs At a PO2 in the lungs of 100 mm Hg, Percent O2 saturation of hemoglobin 100 Hb is 98% saturated. 98% 80 60 40 20 0 0 20 40 60 PO2 (mm Hg) 80 100 At high PO2, large changes in PO2 cause only small changes in Hb saturation. Notice that the curve is relatively flat here. Hb’s properties produce a safety margin that ensures that Hb is almost fully saturated even with a substantial PO 2 decrease. As a result, Hb remains saturated even at high altitude or with lung disease. 95% At high altitude, there is less O2. At a PO in the lungs of only 80 2 mm Hg, Hb is still 95% saturated. 67 Other Factors Influencing Hemoglobin Saturation • Right-ward vs Left-ward shifts • Increases in temperature, H+, Pco2, and BPG • Modify structure of hemoglobin; decrease its affinity for O2 • Occur in systemic capillaries • Enhance O2 unloading from blood • Shift O2-hemoglobin dissociation curve to right • Decreases in these factors shift curve to left • Decreases oxygen unloading from blood, increases Hb affinity for O2 The Bohr Effect A physiological phenomenon describing Hb’s oxygen binding affinity is inversely related to the acidity and the concentration of CO2 Figure 22.21 Effect of temperature, PCO , and blood pH on the oxygen-hemoglobin dissociation curve. 68 Percent O2 saturation of hemoglobin 2 100 10ºC 20ºC 80 38ºC 43ºC 60 40 Normal body temperature 20 0 Percent O2 saturation of hemoglobin (a) 100 Decreased carbon dioxide (PCO2 20 mm Hg) or H+ (pH 7.6) 80 Normal arterial carbon dioxide (PCO 40 mm Hg) 60 2 or H+ (pH 7.4) 40 Increased carbon dioxide (PCO 80 mm Hg) 2 20 0 or H+ (pH 7.2) 20 40 60 80 PO (mm Hg) 2 (b) 100 69 Factors that Increase Release of O2 by Hemoglobin • As cells metabolize glucose and use O2 • Pco2 and H+ increase in capillary blood • Declining blood pH and increasing Pco2 • Bohr effect - Hb-O2 bond weakens oxygen unloading where needed most • Heat production increases directly and indirectly decreases Hb affinity for O2 increased oxygen unloading to active tissues 70 Homeostatic Imbalance • Hypoxia • Inadequate O2 delivery to tissues cyanosis • Anemic hypoxia–too few RBCs; abnormal or too little Hb • Ischemic hypoxia–impaired/blocked circulation • Histotoxic hypoxia–cells unable to use O2, as in metabolic poisons • Hypoxemic hypoxia–abnormal ventilation; pulmonary disease • Carbon monoxide poisoning–especially from fire; 200X greater affinity for Hb than oxygen 71 CO2 Transport • CO2 transported in blood in three forms • 7 to 10% dissolved in plasma • 20% bound to globin of hemoglobin (carbaminohemoglobin) • 70% transported as bicarbonate ions (HCO3–) in plasma 72 Transport and Exchange of CO2 • CO2 combines with water to form carbonic acid (H2CO3), which quickly dissociates • Occurs primarily in RBCs, where carbonic anhydrase reversibly and rapidly catalyzes reaction 73 Transport and Exchange of CO2 • In systemic capillaries • HCO3– quickly diffuses from RBCs into plasma • Chloride shift occurs • Outrush of HCO3– from RBCs balanced as Cl– moves into RBCs from plasma Figure 22.22a Transport and exchange of CO 2 and O2. Tissue cell 74 Interstitial fluid (dissolved in plasma) Slow Binds to plasma proteins Fast Chloride shift (in) via transport protein Carbonic anhydrase (Carbaminohemoglobin) Red blood cell (dissolved in plasma) Oxygen release and carbon dioxide pickup at the tissues Blood plasma 75 Transport and Exchange of CO2 • In pulmonary capillaries • HCO3– moves into RBCs (while Cl- move out); binds with H+ to form H2CO3 • H2CO3 split by carbonic anhydrase into CO2 and water • CO2 diffuses into alveoli Figure 22.22b Transport and exchange of CO 2 and O2. Alveolus 76 Fused basement membranes (dissolved in plasma) Slow Chloride shift (out) via transport protein Fast Carbonic anhydrase (Carbaminohemoglobin) Red blood cell (dissolved in plasma) Oxygen pickup and carbon dioxide release in the lungs Blood plasma 77 Influence of CO2 on Blood pH • Changes in respiratory rate and depth affect blood pH • Slow, shallow breathing increased CO2 in blood drop in pH • Rapid, deep breathing decreased CO2 in blood rise in pH • Changes in ventilation can adjust pH when disturbed by metabolic factors