Respiratory Physiology PDF
Document Details
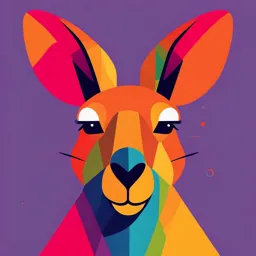
Uploaded by CleanerVision7369
Faculté de Médecine et de Pharmacie de Casablanca
Tags
Summary
This document details respiratory physiology, explaining concepts like respiratory volumes and capacities, pressure, resistance, and airflow in the human respiratory system. The document includes diagrams to support the explanations.
Full Transcript
Respiratory Volumes and Capacities Copyright © The McGraw-Hill Companies, Inc. Permission required for reproduction or display. Maximum possible 6,00 inspiration 0 5,00 0 Inspira...
Respiratory Volumes and Capacities Copyright © The McGraw-Hill Companies, Inc. Permission required for reproduction or display. Maximum possible 6,00 inspiration 0 5,00 0 Inspiratory Inspiratory Vital capacity 4,00 reserve volume capacity 0 Lung volume Tidal 3,00 volume 0 Total lung capacity (mL) 2,00 Expiratory 0 reserve volume Functional residual 1,00 Maximum voluntary capacity 0 Residual expiration volume 0 Figure 22.17b 22-1 Respiratory System: Physiology Pressure, Resistance, and Airflow Respiratory airflow is governed by the same principles of flow, pressure, and resistance as blood flow – The flow of a fluid is directly proportional to the pressure difference between two points – The flow of a fluid is inversely proportional to the resistance Atmospheric pressure drives respiration – The weight of the air above us – 760 mm Hg at sea level, or 1 atmosphere (1 atm) Lower at higher elevations 22-3 Pressure, Resistance, and Airflow Boyle’s law—at a constant temperature, the pressure of a given quantity of gas is inversely proportional to its volume – If the lungs contain a quantity of a gas and the lung volume increases, their internal pressure (intrapulmonary pressure) falls If the pressure falls below atmospheric pressure, air moves into the lungs – If the lung volume decreases, intrapulmonary pressure rises If the pressure rises above atmospheric pressure, air moves out of the lungs 22-4 Inspiration Intrapleural pressure—the slightly negative pressure that exists between the two pleural layers – Recoil of lung tissue and tissues of thoracic cage causes lungs and chest wall to be pulling in opposite directions – The small space between the parietal and visceral pleura is filled with watery fluid, and so these layers stay together – About −5 cm H2O of intrapleural pressure results 22-5 Inspiration The two pleural layers cling together due to the cohesion of water – When the ribs swing upward and outward during inspiration, the parietal pleura follows them – The visceral pleura clings to it by the cohesion of water and it follows the parietal pleura – It stretches the alveoli within the lungs – The entire lung expands along the thoracic cage – As it increases in volume, its internal pressure drops, and air flows in 22-6 Inspiration Another force that expands the lungs is explained by Charles’s law Charles’s law—volume of a gas is directly proportional to its absolute temperature – On a cool day, 16°C (60°F) air will increase its temperature by 21°C (39°F) during inspiration – Inhaled air is warmed to 37°C (99°F) by the time it reaches the alveoli – Inhaled volume of 500 mL will expand to 536 mL and this thermal expansion will contribute to the inflation of the lungs 22-7 Inspiration In quiet breathing, the dimensions of the thoracic cage increase only a few millimeters in each direction – Enough to increase its total volume by 500 mL – Thus, 500 mL of air flows into the respiratory tract 22-8 The Respiratory Cycle Figure 22.16 22-9 Expiration Relaxed breathing – Passive process achieved mainly by elastic recoil of thoracic cage – Recoil compresses the lungs – Volume of thoracic cavity decreases – Raises intrapulmonary pressure to about 1 cm H2O – Air flows down the pressure gradient and out of the lungs Forced breathing – Accessory muscles raise intrapulmonary pressure as high as +40 cm H2O 22-10 Expiration Pneumothorax—presence of air in pleural cavity – Thoracic wall is punctured – Inspiration sucks air through the wound into the pleural cavity – Potential space becomes an air-filled cavity – Loss of negative intrapleural pressure allows lungs to recoil and collapse Atelectasis—collapse of part or all of a lung – Can also result from an airway obstruction as blood absorbs gases from blood 22-11 Alveolar Ventilation Only air that enters alveoli is available for gas exchange Not all inhaled air gets there—about 150 mL fills the conducting division of the airway Anatomic dead space – Conducting division of airway where there is no gas exchange – Can be altered somewhat by sympathetic and parasympathetic stimulation Sympathetic dilation increases dead space but allows greater flow In pulmonary diseases, some alveoli may be unable to exchange gases – Physiologic (total) dead space—sum of anatomic dead space and any pathological alveolar dead space 22-12 Alveolar Ventilation If a person inhales 500 mL of air, and 150 mL stays in anatomical dead space, then 350 mL reaches alveoli Alveolar ventilation rate (AVR) – Air that ventilates alveoli (350 mL) X respiratory rate (12 bpm) = 4,200 mL/min. – This measurement is crucially relevant to the body’s ability to get oxygen to the tissues and dispose of carbon dioxide Residual volume—1,300 mL that cannot be exhaled with maximum effort 22-13 Spirometry—The Measurement of Pulmonary Ventilation Spirometer—a device that recaptures expired breath and records such variables as rate and depth of breathing, speed of expiration, and rate of oxygen consumption Respiratory volumes – Tidal volume: volume of air inhaled and exhaled in one cycle of breathing (500 mL) – Inspiratory reserve volume: air in excess of tidal volume that can be inhaled with maximum effort (3,000 mL) – Expiratory reserve volume: air in excess of tidal volume that can be exhaled with maximum effort (1,200 mL) 22-14 Respiratory Volumes and Capacities Figure 22.17a 22-15 Spirometry—The Measurement of Pulmonary Ventilation (Continued) – Residual volume: air remaining in lungs after maximum expiration (1,300 mL) Allows some gas exchange with blood before next breath of fresh air arrives – Vital capacity: total amount of air that can be inhaled and then exhaled with maximum effort VC = ERV + TV + IRV (4,700 mL) – Important measure of pulmonary health – Inspiratory capacity: maximum amount of air that can be inhaled after a normal tidal expiration IC = TV + IRV (3,500 mL) 22-16 Spirometry—The Measurement of Pulmonary Ventilation (Continued) – Functional residual capacity: amount of air remaining in lungs after a normal tidal expiration FRC = RV + ERV (2,500 mL) – Total lung capacity: maximum amount of air the lungs can contain TLC = RV + VC (6,000 mL) 22-17 Spirometry—The Measurement of Pulmonary Ventilation Spirometry—the measurement of pulmonary function – Aid in diagnosis and assessment of restrictive and obstructive lung disorders Restrictive disorders—those that reduce pulmonary compliance – Limit the amount to which the lungs can be inflated – Any disease that produces pulmonary fibrosis – Black lung disease, tuberculosis 22-18 Spirometry—The Measurement of Pulmonary Ventilation Obstructive disorders—those that interfere with airflow by narrowing or blocking the airway – Make it harder to inhale or exhale a given amount of air – Asthma, chronic bronchitis – Emphysema combines elements of restrictive and obstructive disorders 22-19 Spirometry—The Measurement of Pulmonary Ventilation Forced expiratory volume (FEV) – Percentage of the vital capacity that can be exhaled in a given time interval – Healthy adult reading is 75% to 85% in 1 second Peak flow – Maximum speed of expiration – Blowing into a handheld meter Minute respiratory volume (MRV) – Amount of air inhaled per minute – TV x respiratory rate (at rest 500 x 12 = 6,000 mL/min.) Maximum voluntary ventilation (MVV) – MRV during heavy exercise – May be as high as 125 to 170 L/min 22-20 Variations in the Respiratory Rhythm Eupnea—relaxed, quiet breathing – Characterized by tidal volume 500 mL and the respiratory rate of 12 to 15 bpm Apnea—temporary cessation of breathing Dyspnea—labored, gasping breathing; shortness of breath Hyperpnea—increased rate and depth of breathing in response to exercise, pain, or other conditions Hyperventilation—increased pulmonary ventilation in excess of metabolic demand 22-21 Variations in the Respiratory Rhythm Hypoventilation—reduced pulmonary ventilation leading to an increase in blood CO2 Kussmaul respiration—deep, rapid breathing often induced by acidosis Orthopnea—dyspnea that occurs when person is lying down Respiratory arrest—permanent cessation of breathing Tachypnea—accelerated respiration 22-22 Composition of Air Composition of air – 78.6% nitrogen, 20.9% oxygen, 0.04% carbon dioxide, 0% to 4% water vapor, depending on temperature and humidity, and minor gases argon, neon, helium, methane, and ozone 22-23 Composition of Air Dalton’s law—total atmospheric pressure is the sum of the contributions of the individual gases – Partial pressure: the separate contribution of each gas in a mixture – At sea level 1 atm of pressure = 760 mm Hg – Nitrogen constitutes 78.6% of the atmosphere, thus PN2 = 78.6% x 760 mm Hg = 597 mm Hg PO2 = 20.9% x 760 mm Hg = 159 mm Hg PH2O = 0.5% x 760 mm Hg = 3.7 mm Hg PCO2 = 0.04% x 760 mm Hg = 0.3 mm Hg PN2 + PO2 + PH2O + PCO2 = 760 mmHg 22-24 Alveolar Gas Exchange Alveolar gas exchange—the swapping of O2 and CO2 across the respiratory membrane – Air in the alveolus is in contact with a film of water covering the alveolar epithelium – For oxygen to get into the blood it must dissolve in this water, and pass through the respiratory membrane separating the air from the bloodstream – For carbon dioxide to leave the blood it must pass the other way, and then diffuse out of the water film into the alveolar air 22-25 Alveolar Gas Exchange Gases diffuse down their own gradients until the partial pressure of each gas in the air is equal to its partial pressure in water Henry’s law—at the air–water interface, for a given temperature, the amount of gas that dissolves in the water is determined by its solubility in water and its partial pressure in air – The greater the PO2 in the alveolar air, the more O2 the blood picks up – Since blood arriving at an alveolus has a higher PCO2 than air, it releases CO2 into the air 22-26 Alveolar Gas Exchange Pressure gradient of the gases – Normally: PO2 = 104 mm Hg in alveolar air versus 40 mm Hg in blood PCO2 = 46 mm Hg in blood arriving versus 40 mm Hg in alveolar air – Hyperbaric oxygen therapy: treatment with oxygen at greater than 1 atm of pressure Gradient difference is more, and more oxygen diffuses into the blood Treat gangrene, carbon monoxide poisoning – At high altitudes, the partial pressures of all gases are lower Gradient difference is less, and less oxygen diffuses into the blood 22-27 Changes in Gases Copyright © The McGraw-Hill Companies, Inc. Permission required for reproduction or display. Expired Inspired air air PO2 116 mm PO2 159 mm Hg PCO2 32 mm Hg P CO2 0.3 mm Hg Hg Alveolar gas Alveolar exchange air O2 PO2 104 mm loading Hg PCO2 40 mm CO2 unloading Hg CO O 2 2 Gas transport Pulmonary O2 carried circuit from alveoli to systemic Deoxygenat CO 2 carried tissues ed Oxygenated from blood blood PO2 40 mm PO2 95 mm systemic PHg CO2 46 mm PHg CO2 40 mm tissues to alveoli Hg Hg Systemic circuit Systemic CO O gas 2 2 exchange O2 unloading CO2 loading Tissue fluid PO2 40 mm Figure 22.19 PHg CO2 46 mm Hg 22-28 Pulmonary Alveoli in Health and Disease Copyright © The McGraw-Hill Companies, Inc. Permission required for reproduction or display. (a) Normal Fluid and blood cells Alveolar in alveoli walls thicken ed by (b) edema Pneumonia Conflue nt alveoli Figure 22.21 22-29 (c) Alveolar Gas Exchange Membrane thickness—only 0.5 μm thick – Presents little obstacle to diffusion – Pulmonary edema in left ventricular failure causes edema and thickening of the respiratory membrane – Pneumonia causes thickening of respiratory membrane – When membrane is thicker, gases have farther to travel between blood and air and cannot equilibrate fast enough to keep up with blood flow 22-30 Alveolar Gas Exchange Ventilation–perfusion coupling—the ability to match air flow and blood flow to each other – Gas exchange requires both good ventilation of alveolus and good perfusion of the capillaries – Pulmonary blood vessels change diameter depending on air flow to an area of the lungs Example: If an area is poorly ventilated, pulmonary vessels constrict – Bronchi change diameter depending on blood flow to an area of the lungs Example: If an area is well perfused, bronchodilation occurs 22-31 Ventilation–Perfusion Coupling Copyright © The McGraw-Hill Companies, Inc. Permission required for reproduction or display. Reduced PO2 Decreased Increase Elevated PO2 in airflow d in blood airflow blood Result: vessels Respons Response vessels Blood flow e to matches to increased airflow reduced ventilation Vasoconstriction Vasodilation of ventilatio pulmonary of n vessels pulmonary vessels Decrease Increase d d blood blood flow flow (a) Perfusion adjusted to changes in ventilation 22-32 Figure 22.22a Gas Transport Gas transport—the process of carrying gases from the alveoli to the systemic tissues and vice versa Oxygen transport – 98.5% bound to hemoglobin – 1.5% dissolved in plasma Carbon dioxide transport – In transport: 90% is hydrated to form carbonic acid (dissociates into bicarbonate ions); 5% is bound to proteins; and 5% is dissolved as a gas in plasma – In exchange: 70% of CO2 comes from carbonic acid; 23% comes from proteins; and 7% comes straight from plasma 22-33 Oxygen Arterial blood carries about 20 mL of O2 per deciliter Hemoglobin—molecule specialized for oxygen transport – Four protein (globin) portions Each with a heme group that binds one O2 to an iron atom One hemoglobin molecule can carry up to 4 O2 – 100% saturation Hb with 4 O2 molecules per Hb – 50% saturation Hb with 2 O2 molecules per Hb Oxyhemoglobin (HbO2)—O2 bound to hemoglobin Deoxyhemoglobin (HHb)—hemoglobin with no O2 22-34 Oxyhemoglobin Dissociation Curve Copyright © The McGraw-Hill Companies, Inc. Permission required for reproduction or display. 2 10 0 0 O2 unloaded to 8 1 systemic 0 5 tissues Percentage O2 saturation of 6 mL O2 /dL of 0 1 0 blood 4 hemoglobin 0 5 2 0 0 Figure 22.23 0 2 4 6 8 10 0 0 0 0 0 Systemic Alveo tissues li Partial pressure of O2 (PO2) in mm Hg Relationship between hemoglobin saturation and PO2 is nonlinear 22-35 (binding facilitates loading; ultimate saturation) Carbon Dioxide Carbon dioxide transported in three forms – Carbonic acid, carbamino compounds, and dissolved in plasma 90% of CO2 is hydrated to form carbonic acid – CO2 + H2O → H2CO3 → HCO3- + H+ – Then dissociates into bicarbonate and hydrogen ions 5% binds to the amino groups of plasma proteins and hemoglobin to form carbamino compounds—chiefly carbaminohemoglobin (HbCO2) – Carbon dioxide does not compete with oxygen – They bind to different moieties on the hemoglobin molecule – Hemoglobin can transport O2 and CO2 simultaneously 22-36 Carbon Dioxide (Continued) 5% is carried in the blood as dissolved gas Relative amounts of CO2 exchange between the blood and alveolar air differs – 70% of exchanged CO2 comes from carbonic acid – 23% from carbamino compounds – 7% dissolved in the plasma Blood gives up the dissolved CO2 gas and CO2 from the carbamino compounds more easily than CO2 in bicarbonate 22-37 Carbon Monoxide Poisoning Carbon monoxide (CO)—competes for the O2 binding sites on the hemoglobin molecule Colorless, odorless gas in cigarette smoke, engine exhaust, fumes from furnaces and space heaters Carboxyhemoglobin—CO binds to iron of hemoglobin (Hb) – Binds 210 times as tightly as oxygen and ties up Hb for a long time – Nonsmokers: less than 1.5% of Hb occupied by CO – Smokers: 10% of Hb occupied by CO in heavy smokers – Atmospheric concentration of 0.2% CO is quickly lethal Adjustment to the Metabolic Needs of Individual Tissues Hemoglobin unloads O2 to match metabolic needs of different states of activity of the tissues Four factors adjust the rate of oxygen unloading to match need: ambient PO2, temperature, the Bohr effect, concentration of biphosphoglycerate (BPG) – Ambient PO2 Active tissue has ↓ PO2; O2 is released from Hb – Temperature Active tissue has ↑ temp; promotes O2 unloading 22-39 Adjustment to the Metabolic Needs of Individual Tissues Adjustment of oxygen unloading (Continued) – Bohr effect Active tissue has ↑ CO2, which lowers pH of blood; promoting O2 unloading – Bisphosphoglycerate (BPG) RBCs produce BPG which binds to Hb; O2 is unloaded ↑ body temp (fever), thyroxine, growth hormone, testosterone, and epinephrine all raise BPG and promote O2 unloading Rate of CO2 loading also adjusted to meet needs – Haldane effect—low level of oxyhemoglobin enables the blood to transport more CO2 22-40 Effects of Temperature on Oxyhemoglobin Dissociation Copyright © The McGraw-Hill Companies, Inc. Permission required for reproduction or display. 10 0 10º 90 C 20º C 38º 80 C 43º C 70 Percentage saturation of 60 Normal 50 body temperature 40 30 hemoglobin 20 10 0 0 2 4 6 80 10 12 0 0 0 0 0 PO2 (mm 22-41 (a) Effect of Hg) Figure 22.26a temperature Effects of pH on Oxyhemoglobin Dissociation Copyright © The McGraw-Hill Companies, Inc. Permission required for reproduction or display. 10 0 90 pH 7.60 80 pH 7.40 Percentage saturation of 70 (normal blood pH) 60 pH 7.20 50 40 hemoglobin 30 20 10 0 0 20 40 60 80 100 120 PO2 (mm Hg) Figure 22.26b (b) Effect of pH 22-42 Bohr effect: release of O2 in response to low pH Blood Gases and the Respiratory Rhythm Rate and depth of breathing adjust to maintain levels of: – pH 7.35 to 7.45 – PCO2 40 mm Hg – PO2 95 mm Hg Brainstem respiratory centers receive input from central and peripheral chemoreceptors that monitor composition of CSF and blood Most potent stimulus for breathing is pH, followed by CO2, and least significant is O2 22-43 Hydrogen Ions Pulmonary ventilation is adjusted to maintain pH of the brain – Central chemoreceptors in medulla produce about 75% of the change in respiration induced by pH shift CO2 crosses blood-brain-barrier and reacts with water in CSF to produce carbonic acid The H+ from carbonic acid strongly stimulates central chemoreceptors, since CSF does not contain much protein buffer Hydrogen ions also stimulate peripheral chemoreceptors which produce 25% of the respiratory response to pH changes 22-44 Hydrogen Ions Acidosis—blood pH lower than 7.35 Alkalosis—blood pH higher than 7.45 Hypocapnia—PCO2 less than 37 mm Hg (normal 37 to 43 mm Hg) Most common cause of alkalosis Hypercapnia—PCO2 greater than 43 mm Hg Most common cause of acidosis 22-45 Hydrogen Ions Respiratory acidosis and respiratory alkalosis—pH imbalances resulting from a mismatch between the rate of pulmonary ventilation and the rate of CO 2 production Hyperventilation can be a corrective homeostatic response to acidosis – “Blowing off” CO2 faster than the body produces it – Pushes reaction to the left: CO2 (expired) + H2O ← H2CO3 ← HCO3- + ↓ H+ – Reduces H+ (reduces acid), raises blood pH toward normal 22-46 Hydrogen Ions Hypoventilation can be a corrective homeostatic response to alkalosis – Allows CO2 to accumulate in body fluids faster than we exhale it – Shifts reaction to the right: CO2 + H2O → H2CO3 → HCO3- + H+ – Raising the H+ concentration, lowering pH to normal 22-47 Hydrogen Ions Ketoacidosis—acidosis brought about by rapid fat oxidation releasing acidic ketone bodies (seen in diabetes mellitus) – Induces Kussmaul respiration: hyperventilation that reduces CO2 concentration and compensates (to some degree) for the acidity of ketone bodies 22-48 Carbon Dioxide CO2 has strong indirect effects on respiration – Through pH, as described previously Direct effects – ↑ CO2 at beginning of exercise may directly stimulate peripheral chemoreceptors and trigger ↑ ventilation more quickly than central chemoreceptors 22-49 Oxygen PO2 usually has little effect on respiration Chronic hypoxemia, PO2 less than 60 mm Hg, can significantly stimulate ventilation – Hypoxic drive: respiration driven more by low PO2 than by CO2 or pH – Emphysema, pneumonia – High elevations after several days 22-50 Respiration and Exercise Causes of increased respiration during exercise – When the brain sends motor commands to the muscles It also sends this information to the respiratory centers They increase pulmonary ventilation in anticipation of the needs of the exercising muscles – Exercise stimulates proprioceptors of muscles and joints They transmit excitatory signals to brainstem respiratory centers Increase breathing because they are informed that muscles are moving Increase in pulmonary ventilation keeps blood gas values at their normal levels in spite of the elevated O2 consumption and CO2 generation by the muscles 22-51 Oxygen Imbalances Hypoxia—a deficiency of oxygen in a tissue or the inability to use oxygen – A consequence of respiratory diseases Hypoxemic hypoxia—state of low arterial PO2 – Usually due to inadequate pulmonary gas exchange – Oxygen deficiency at high elevations, impaired ventilation: drowning, aspiration of a foreign body, respiratory arrest, degenerative lung diseases Ischemic hypoxia—inadequate circulation of blood – Congestive heart failure 22-52 Oxygen Imbalances Anemic hypoxia—due to anemia resulting from the inability of the blood to carry adequate oxygen Histotoxic hypoxia—metabolic poisons such as cyanide prevent tissues from using oxygen Cyanosis—blueness of the skin – Sign of hypoxia 22-53 Oxygen Imbalances Although safe to breathe 100% oxygen at 1 atm for a few hours, oxygen toxicity develops when pure O2 breathed at 2.5 atm or greater – Generates free radicals and H2O2 – Destroys enzymes – Damages nervous tissue – Leads to seizures, coma, death Hyperbaric oxygen – Formerly used to treat premature infants, caused retinal damage, was discontinued 22-54 Chronic Obstructive Pulmonary Diseases Chronic obstructive pulmonary disease (COPD)—long- term obstruction of airflow and substantial reduction in pulmonary ventilation Major COPDs are chronic bronchitis and emphysema – Almost always associated with smoking – Other risk factors include: air pollution, occupational exposure to airborne irritants, hereditary defects 22-55 Chronic Obstructive Pulmonary Diseases Chronic bronchitis – Severe, persistent inflammation of lower respiratory tract – Goblet cells enlarge and produce excess mucus – Immobilized cilia fail to remove mucus – Thick, stagnant mucus – ideal for bacterial growth – Smoke compromises alveolar macrophage function – Develop chronic cough to bring up sputum (thick mucus and cellular debris) – Symptoms include hypoxemia and cyanosis 22-56 Chronic Obstructive Pulmonary Diseases Emphysema – Alveolar walls break down Lung has fewer and larger spaces Much less respiratory membrane for gas exchange – Lungs fibrotic and less elastic Lungs become flabby and cavitated with large spaces – Air passages collapse Obstructs outflow of air Air trapped in lungs; person becomes barrel-chested – Weaken thoracic muscles Spend three to four times the amount of energy just to breathe 22-57 Chronic Obstructive Pulmonary Diseases COPD reduces vital capacity COPD causes: hypoxemia, hypercapnia, and respiratory acidosis – Hypoxemia stimulates erythropoietin release from kidneys, and leads to polycythemia Cor pulmonale – Hypertrophy and potential failure of right heart due to obstruction of pulmonary circulation 22-58 Smoking and Lung Cancer Lung cancer accounts for more deaths than any other form of cancer – Most important cause is smoking (at least 60 carcinogens) Squamous-cell carcinoma (most common form) – Begins with transformation of bronchial epithelium into stratified squamous from ciliated pseudostratified epithelium – Dividing cells invade bronchial wall, cause bleeding lesions – Dense swirls of keratin replace functional respiratory tissue 22-59 Smoking and Lung Cancer Adenocarcinoma – Originates in mucous glands of lamina propria Small-cell (oat cell) carcinoma – Least common, most dangerous – Named for clusters of cells that resemble oat grains – Originates in primary bronchi, invades mediastinum, metastasizes quickly to other organs 22-60 Smoking and Lung Cancer 90% originate in mucus membranes of large bronchi Tumor invades bronchial wall, compresses airway; may cause atelectasis Often first sign is coughing up blood Metastasis is rapid; usually occurs by time of diagnosis – Common sites: pericardium, heart, bones, liver, lymph nodes, and brain Prognosis poor after diagnosis – Only 7% of patients survive 5 years 22-61 Smoking and Lung Cancer 22-62 Figure 22.27 Respiration and health Upper respiratory tract infections Sinusitis – blockage of sinuses Otitis media – infection of the middle ear Tonsillitis – inflammation of the tonsils Laryngitis – infection of the larynx that leads to loss of voice Respiration and health Lower respiratory tract disorders Pneumonia – infection of the lungs with thick, fluid build up Tuberculosis – bacterial infection that leads to tubercles (capsules) Pulmonary fibrosis – lungs lose elasticity because fibrous connective tissue builds up in the lungs usually because of inhaled particles Emphysema – chronic, incurable disorder in which alveoli are damaged and thus the surface area for gas exchange is reduced Asthma – bronchial tree becomes irritated causing breathlessness, wheezing, and coughing Lung cancer – uncontrolled cell division in the lungs that is often caused by smoking and can lead to death Some Diseases and Disorders of the Respiratory System Copyright © The McGraw-Hill Companies, Inc. Permission required for reproduction or display. mucus Pneumonia Bronchitis Alveoli fill with pus and fluid, Airways are inflamed due making gas exchange difficult. to infection (a cute) or due to an irritant (chronic). Coughing brings up mucus and pus. asbestos tubercle body Pulmonary Fibrosis Pulmonary Tuberculosis Emphysema Asthma Fibrous connective tissue Tubercles encapsulate Alveoli burst and fuse into Airways are inflamed due builds up in lungs, bacteria, and elasticity of enlarged air spaces. Surface to irritation, and bronchioles reducing lungs is reduced. area constrict due to muscle their elasticity. for gas exchange is reduced. spasms. 9.7 Respiration and health Health focus: Things you should know about tobacco and health All forms of tobacco can cause damage Smoking increases a person’s chance of lung, mouth, larynx, esophagus, bladder, kidney, pancreatic, stomach, and cervix cancers. The 5-year survival rate for people with lung cancer is only 13% Smoking also increases the chance of chronic bronchitis, emphysema, heart disease, stillbirths, and harm to an unborn child Passive smoke can increase a nonsmoker’s chance of pneumonia, bronchitis, and lung cancer