Gas Transport PDF
Document Details
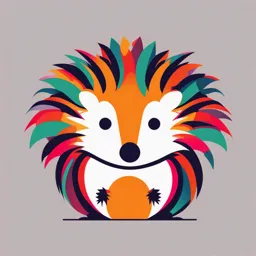
Uploaded by SlickCharoite5520
Babylon Medical College
Dr.Zina H. Mohammed
Tags
Summary
This document provides an overview of gas transport in the human body, focusing on oxygen and carbon dioxide. It describes the processes involved in the transport of these gases, including hemoglobin's role, and factors influencing the process. Various aspects of gas exchange, such as the oxyhemoglobin dissociation curve, are discussed.
Full Transcript
Dr.Zina H. Mohammed Oxygen is carried in the blood in two main forms: 1-Dissolved in Plasma: A small amount of oxygen (about 3% of total oxygen) is dissolved directly in the plasma. The solubility of oxygen in plasma is relatively low, so this form of oxygen transport is less significant than...
Dr.Zina H. Mohammed Oxygen is carried in the blood in two main forms: 1-Dissolved in Plasma: A small amount of oxygen (about 3% of total oxygen) is dissolved directly in the plasma. The solubility of oxygen in plasma is relatively low, so this form of oxygen transport is less significant than the oxygen bound to hemoglobin. However, the dissolved oxygen is still important for maintaining oxygen pressure (partial pressure of oxygen, or PaO2) and facilitating the movement of oxygen into tissues. 2-Bound to Hemoglobin (Hb): The majority of oxygen in the blood is bound to hemoglobin (97% of O2 in blood is transported by combining with Hb ), a protein found in red blood cells. Hemoglobin has four binding sites for oxygen molecules, and when oxygen binds to these sites, it forms oxyhemoglobin. This form of oxygen transport is highly efficient, as hemoglobin picks up oxygen in the lungs and releases it in tissues where the oxygen concentration is lower. These two forms of oxygen allow for efficient oxygen delivery to tissues throughout the body. Each gram of Hb binds to 1.34ml O2, so 1.34 X 15gm = 20.1ml O2 in 100% saturation. So in 97% saturation the blood carries 19.4 ml O2. In tissues, the saturation 40% and in veins (75%) carrying 14.4ml O2 in each 100ml of blood. Each hemoglobin has 4 polypeptide chains and 4 hemes. In the center of each heme group is 1 atom of iron that can combine with 1 molecule O 2 , So each Hb carry 4 O2 molecules. Oxyhemoglobin Dissociation Curve The oxyhemoglobin dissociation curve illustrates the relationship between the partial pressure of oxygen (PO2 ) and the percentage of hemoglobin saturated with oxygen (hemoglobin's oxygen saturation). PO2 is the most important factor determining whether O2 and Hb combine or dissociate according to O2-Hb Dissociation curve (DC) This curve is essential for understanding how hemoglobin binds and releases oxygen in different environments within the body. Shape of the Curve: The oxyhemoglobin dissociation curve has a sigmoidal (S- shaped) form. This shape reflects cooperative binding of oxygen to hemoglobin. When one oxygen molecule binds to hemoglobin, it increases the affinity of the remaining binding sites for oxygen. This phenomenon is known as positive cooperativity. High PO2(e.g., in the lungs): When the PO2 is high (in the lungs, where oxygen concentration is high), the curve is steep, and hemoglobin becomes nearly fully saturated with oxygen. At this point, hemoglobin binds oxygen more easily and releases it less readily. Low PO2 (e.g., in tissues): In tissues, where oxygen is consumed and PO2 is low, hemoglobin releases oxygen more readily. The lower the PO2 , the less hemoglobin is saturated with oxygen. As the curve flattens, hemoglobin's affinity for oxygen decreases, facilitating oxygen unloading. T h e f l at p o s i t i o n o f c u r v e ex te n d s f ro m 100mmHg to 60mmHg. This means, human can tolerate substantial decreases in alveolar PO2 to 60mmHg (e.g. caused by decreases in atmosphere pressure) without compromising the O2-carrying capacity of Hb. Factors that Affect the Curve: Several factors can shift the oxyhemoglobin dissociation curve to the left or right, affecting hemoglobin's affinity for oxygen: Rightward Shift (Decreased affinity for oxygen): Increased temperature (e.g., during exercise) Increased CO2 (carbon dioxide) concentration Decreased pH (acidosis) Decrease PO2 The Bohr effect: a decrease in pH lowers hemoglobin's affinity for oxygen, helping it release oxygen more easily in metabolically active tissues. Increased 2,3-Disphosphoglycerate (2,3-DPG): A byproduct of red blood cell metabolism, which also promotes oxygen unloading. Leftward Shift (Increased affinity for oxygen): Decreased temperature Decreased CO2 Increased pH (alkalosis) Decreased 2,3-DPG Increase PO2 A leftward shift indicates that hemoglobin binds oxygen more tightly and does not release it as easily, which can occur in conditions like hypothermia or respiratory alkalosis. Normal Values: Under normal conditions, arterial blood has a PO2 around 95-100 mmHg and hemoglobin is about 98% saturated with oxygen. In venous blood, where PO2 is around 40 mmHg, hemoglobin is around 75% saturated with oxygen. Dissolved CO2 (7-10%): A small portion of CO2 is dissolved directly in the plasma. It is in equilibrium with carbonic acid, which dissociates into bicarbonate and hydrogen ions. This dissolved CO2 is the form that can diffuse into and out of the blood cells more readily. Bicarbonate (HCO₃ ⁻ ) (70-80%): The majority of CO2 in the blood is converted into bicarbonate ions (HCO₃ ⁻ ) by the enzyme carbonic anhydrase within red blood cells. CO2 reacts with water to form carbonic acid (H₂ CO₃ ), which quickly dissociates into bicarbonate ions and hydrogen ions. The bicarbonate is then transported in the plasma, with chloride ions exchanged in a process called the chloride shift to maintain electrical neutrality. The H+ remains in RBC where it will be buffered by deoxyhemoglobin and is carried in venous blood in this Electrical Neutrality: The chloride shift ensures that the RBCs maintain a balance of electrical charge (anions and cations) as bicarbonate leaves and chloride enters. This process is crucial for maintaining the proper ionic balance during CO₂ transport. When blood reaches the venous end of the capillaries Hb is conveniently in its deoxygenated form (i.e. it has released its O2 to the tissues). There is a useful reciprocal relationship between the buffering of H+ by deoxyhemoglobin and the Bohr effect. Thus the H+ generated from the tissue CO2 causes hemoglobin to release O2 more readily to the tissues. In turn, deoxgenation of Hb makes it better buffer for H+. In the Lungs: In the lungs, the process is reversed. Bicarbonate ions enter the red blood cells again, where they combine with hydrogen ions (H⁺ ) to form carbonic acid. Carbonic anhydrase then converts carbonic acid back into CO₂ and water, and the CO₂ is expelled from the body when exhaled. This entire process is vital for maintaining acid-base balance and efficiently transporting carbon dioxide out of tissues and into the lungs for exhalation. When RBC enters pulmonary capillaries, O2 diffuses into cell and combines with Hb, which releases CO2 (Haldane effect). Called revers chloride shift. Carbamino compounds (20-23%): CO2 also binds to amino groups on proteins, particularly hemoglobin, to form carbaminohemoglobin. This occurs primarily in red blood cells and helps to carry CO2 back to the lungs for exhalation. The binding of CO2 to hemoglobin is affected by factors like the concentration of oxygen, a phenomenon known as the Haldane effect. These three forms collectively allow the body to efficiently transport CO2 from tissues to the lungs for elimination. Chloride shift Hypoxia Hypoxia refers to a condition where there is a deficiency of oxygen in the tissues of the body, despite normal blood circulation. This lack of sufficient oxygen can affect the functioning of various organs and tissues. Types of Hypoxia: 1-Hypoxic Hypoxia: Caused by a lack of oxygen in the air (e.g., high altitudes) or poor oxygenation of the blood in the lungs (e.g., respiratory diseases like COPD, asthma, or pneumonia). 2-Anemic Hypoxia: Occurs when there is inadequate hemoglobin or red blood cells to carry oxygen to tissues, even if the amount of oxygen in the blood is normal. This can be caused by anemia or blood loss. 3-Circulatory (Stagnant) Hypoxia: Occurs when blood flow is impaired, such as in heart failure, shock, or when blood vessels are blocked or narrowed, leading to inadequate oxygen delivery to tissues. 4-Histotoxic Hypoxia: Occurs when tissues are unable to utilize oxygen effectively, even though the oxygen supply is adequate. This can be caused by toxins (e.g., cyanide poisoning), metabolic disorders, or cellular dysfunction. High-altitude High-altitude physiology refers to how the human body responds to the environmental changes encountered at elevated altitudes, typically starting around 2,500 meters above sea level. These changes include decreased atmospheric pressure, lower oxygen levels, and cooler temperatures. The body's responses to these conditions can be acute, chronic, or even pathological, depending on the altitude, duration of exposure, and individual adaptation. 1. Reduced Atmospheric Pressure and Oxygen Availability Atmospheric Pressure: As altitude increases, the air pressure decreases, which means there are fewer air molecules in a given volume, including oxygen molecules. Oxygen Partial Pressure: Although the percentage of oxygen in the air remains constant at 21%, the reduced atmospheric pressure results in a lower partial pressure of oxygen. Immediate Physiological Responses (Acute Adaptation) When exposed to high altitudes, the body immediately begins compensating for the reduced oxygen availability: Increased Ventilation (Hyperventilation): The body responds to low oxygen by increasing the rate and depth of breathing. This occurs rapidly within minutes to hours of exposure and helps raise oxygen levels in the blood. Hypoxia: Reduced oxygen leads to a state known as hypoxia.The body senses this and triggers mechanisms like the release of erythropoietin (EPO), a hormone that stimulates the production of red blood cells to improve oxygen-carrying capacity. Cardiovascular Adjustments: The heart rate increases to improve the circulation of oxygenated blood. The stroke volume may also increase in the short term. Decrease affinity of Hb to O2: low O2 of RBCs stimulates 2-3 DPG production and decreases the affinity of Hb to oxygen. 3. Long-Term Adaptations (Chronic Adaptation) With prolonged exposure to high altitude, the body undergoes additional physiological changes: Acclimatization: Over days to weeks, the body adapts to the lower oxygen levels. The primary mechanism is the increase in red blood cell count due to the elevated levels of erythropoietin. This adaptation enhances the blood's capacity to carry oxygen. Increased Capillary Density: The body may develop more capillaries in tissues (like muscles) to increase oxygen delivery and exchange. Deep-sea diving Deep-sea diving can significantly impact respiratory physiology due to changes in environmental pressure, gas composition, and breathing patterns. Here are the key respiratory changes associated with deep-sea diving: 1. Increased Ambient Pressure: As a diver descends, the pressure around them increases. At depths of 10 meters , the pressure is twice that at sea level. This increased pressure affects the lungs, causing them to compress. Lung Compression: As pressure increases, the air in the lungs is compressed. Breathing Volume: The volume of air that a diver can inhale decreases due to the increased pressure at depth, which can lead to more rapid or deeper breaths at greater depths. 2. Gas Exchange Changes: Oxygen (O₂ ): At deeper depths, the partial pressure of oxygen increases. This increases the amount of oxygen dissolved in the blood, which can be beneficial up to a certain point, but at very deep depths, it can become toxic. Oxygen toxicity can lead to seizures and other serious health issues. Carbon Dioxide (CO₂ ): The concentration of carbon dioxide in the blood may rise due to increased metabolic activity or insufficient ventilation during deep dives. This can lead to respiratory acidosis (low pH in the blood) and increase the urge to breathe. 3. Decreased Lung Compliance: Compression of Lung Tissue: At depths greater than 10 meters, the chest wall and lungs become stiffer, and the lungs' compliance decreases. This makes it harder to inhale deeply and may require more effort to maintain adequate ventilation. 4. Breathing Patterns: Increased Breathing Rate: At greater depths, divers may unconsciously breathe more rapidly and shallowly due to the body's response to increased pressure and altered gas exchange. Hypercapnia: In certain cases, especially with deeper dives, the body may experience a buildup of CO₂ (hypercapnia). This is due to increased production of CO₂ from metabolic activity combined with reduced efficiency in gas exchange at higher pressures. 5. Barotrauma: Changes in pressure can cause air spaces in the body (e.g., sinuses, middle ear, and lungs) to become imbalanced, potentially leading to barotrauma. This condition can result in discomfort, pain, and damage to lung tissue or other air-filled cavities, especially if equalization procedures are not followed.