Renal System Chapters 19 & 20 Fall 2024 PDF
Document Details
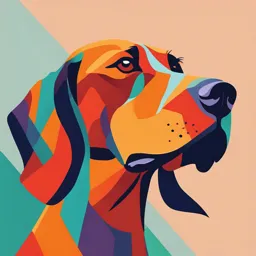
Uploaded by AwedDaisy
Tags
Summary
This document provides an overview of the renal system, including its functions and anatomy. It details the processes within the nephron, specifically covering aspects like filtration and reabsorption. The materials in this document appear to be lecture notes and not an examination paper.
Full Transcript
Renal System Chapters 19 & 20 Functions of the Renal System The renal system has six functions: Regulation of extracellular fluid volume and blood pressure Regulation of body fluid osmolarity. Maintenance of ion balance in the body fluids Plays a role in homeostatic regulation of bo...
Renal System Chapters 19 & 20 Functions of the Renal System The renal system has six functions: Regulation of extracellular fluid volume and blood pressure Regulation of body fluid osmolarity. Maintenance of ion balance in the body fluids Plays a role in homeostatic regulation of body fluid pH Excretion of wastes Production of hormones Anatomy: The Renal System The basic components of the renal system are illustrated in this figure. The components include: The kidneys: filter the blood and produce urine. The Ureter: muscular tube that connects the kidney to the urinary Kidney bladder. Conveys urine from the kidney to the bladder. Ureter The Urinary Bladder: where the urine is stored until it is excreted from the Urinary bladder body. Urethra Urethra: a tube that conveys the urine from the bladder to the external body. Anatomy: The Renal System The main organ of the renal system is the kidney. Structually the kidney is composed of two layers, an outer layer called the cortex, and an inner layer called the medulla. The medulla surrounds the upper part of the ureter, which is called the renal pelvis. The renal pelvis receives urine produced in the cortex and medulla and conducts it to the ureter. The cortex and medulla are composed of small tubules called nephrons. The nephrons are the functional unit of the kidney. Anatomy: The Renal System The nephrons are tubules that are associated with The cortex contains all blood vessels. The Bowman’s capsules, nephrons extend through Proximal and distal tubules. the cortex and medulla and open into the renal The medulla contains pelvis. The filtering of the loops of Henle And collecting blood and processing of ducts. the filtrate into urine is the function of the nephrons. Renal pelvis Renal pelvis Anatomy: The Renal System The blood vessels associated with the nephron are referred to as the vascular element of the nephron. The tubule is referred to as the tubular element of the nephron. Anatomy: The Renal System The vascular element consists of: An afferent arteriole: receives blood from the renal artery that supplies blood to the kidney. The afferent arteriole connects to the glomerulus, a network of capillaries associated with Bowman’s capsule of the tubule. The glomerulus connects to an efferent arteriole, which connects to a network of capillaries called the peritubular capillaries that wrap around the other parts of the tubule. Anatomy: The Renal System The tubular element consists of 4 parts that carry out different functions: Bowman’s capsule, which surrounds the glomerular capillaries. Proximal convoluted tubule (PCT) Loop of Henle, divided into an descending and ascending limb. Distal convoluted tubule (DCT) The DCT connects to a Collecting Duct, which is empties into the renal pelvis. A collecting duct may be attached to up to 7 tubules. Anatomy: The Renal System The distal tubule actually passes between the efferent and afferent arterioles to form the juxtaglomerular apparatus (JA). The JA plays an important role in regulating the rate of filtration of the blood (referred to as the Glomerular Filtration Collecting duct Rate or GFR). Nephron Functions: Filtration, Reabsorption, Secretion, and Excretion Peritubular capillaries Distal tubule S R F R S Bowman’s Proximal Hormonally regulated capsule tubule reabsorption of water and Na+, and secretion R of K+ S R R Renal artery (20% of afferent arteriole plasma Loop KEY of filters into BC). Henle = Filtration: blood to lumen = Reabsorption: lumen to blood Collecting duct = Secretion: blood to lumen E = Excretion: lumen to external Selective reabsorption of (urine) environment water and some ions (Na, K, To bladder and Cl) external environment Nephron Functions: Filtration Filtering of blood plasma into the lumen of the nephron takes place across the walls of the glomerular capillaries and Bowman’s Capsule. About 20% of the blood plasma volume that passes through the glomerular capillaries filters into the lumen of the Bowman’s Capsule. Nephron Functions: Filtration Occurs by bulk flow: Everything in the blood passes freely out of the blood and into the lumen of the Bowman’s Capsule, except for blood cells and blood proteins. Glomerular capillaries are fenestrated but their pores are too small to allow blood cells to pass, and the negative charge on the rim or the pores prevents blood proteins from passing. Filtrate in Bowman’s capsule consists of water and dissolved solutes (ions, glucose, amino acids, etc). Nephron Functions: Filtration Forces That Influence Filtration Hydrostatic Pressure (PH) created by pressure of the blood flowing through the glomerular capillaries. Colloid osmotic pressure inside the glomerular capillaries is higher than that of the fluid in Bowman’s capsule due to the retention of proteins in the plasma and blood cells. Fluid Pressure (Pfluid )= fluid pressure created by the filtrate pushing on the walls of Bowman’s capsule back against the capillaries of the glomerulus. Nephron Functions: Filtration The net filtration pressure pushing fluid across the capillary-Bowman’s capsule interface depends on hydrostatic pressure, colloid osmotic pressure, and fluid pressure according to the formula: Net Filtration Pressure = PH – (p + Pfluid) Normal PH = 55 mmHg Normal colloid osmotic pressure (p) = 30 mmHg Normal Pfluid = 15 mmHg Plugging these numbers into the formula gives a net filtration pressure of 10mmHg. Glomerular Filtration Rate (GFR) The GFR is the expression of the filtration efficiency of the kidneys. GFR is defined as the amount of fluid that filters from the glomeruli into the Bowman’s capsules of all the nephrons of the kidneys per unit time. Normal GFR = 180L/Day (125ml/min) Note: Since the normal total blood volume is 5L, the total blood volume is normally filtered 36 times every day. Three types of physiologic mechanisms regulate the GFR: Autoregulatory mechanisms Autonomic nervous system (sympathetic) Hormones All of the mechanisms work together in regulating the GFR. Mechanisms Regulating the GFR All of these mechanisms work by regulating the blood pressure in the afferent arteriole, which regulates the hydrostatic pressure (PH ) of filtration across the glomerular capillary- Bowman’s Capsule interface. Due to the function of these mechanisms, as long as the mean arterial pressure remains between 80 and 180mmHg the GFR remains about 180L/day. There are two autoregulatory mechanisms that regulate the GFR: The myogenic Response The tubuloglomerular feedback mechanism (TGFM) These are the main regulators of the GFR. Mechanisms Regulating the GFR: The Myogenic Response Myogenic response mechanism: maintains the GFR at around 180 L/Day regardless of changes in the systematic blood pressure. It results from the ability of the smooth muscle of the afferent arteriole to respond to stretch by contracting. Mechanisms Regulating the GFR: The Myogenic Response An increase systematic BP increases stretch in wall of afferent arterioles in the kidneys, which the afferent arterioles respond to by vasoconstricting. Vasoconstriction of afferent arterioles decreases the radius of the arterioles increasing resistance to blood flow through the afferent arterioles decreasing blood flow through the afferent arterioles and into the glomeruli, which offsets the increase in systemic BP. A decrease in systemic BP causes decreases stretch in the wall of the afferent arterioles, which respond by vasodilating. Vasodilation of afferent arterioles increases the radius of the afferent arteriole reducing resistance to blood flow through the afferent arteriole increasing blood flow through the afferent arterioles and into the glomeruli, which offsets the decrease in systemic BP. Therefore, although systemic BP may change, PH in the glomeruli remains the same, and so GFR remains 180 L/day. Mechanisms Regulating the GFR: Tubuloglomerular Feedback Mechanism (TGFM) The TGFM is a result of the functioning of the juxtaglomerular apparatus that is formed where the distal tubule passes between Efferent arteriole Bowman’s capsule Distal tubule the afferent and efferent arterioles. Glomerulus Macula densa 4 1 5 At this point in the nephron, the Afferent distal tubule has a specialized arteriole group of cells in it wall called the Granular 3 2 Proximal macula densa. These cells secrete cells tubule a chemical signal (a paracrine) that is involved in the TGFM. Also at this point in the nephron Collecting duct the afferent arteriole is Loop surrounded by cells called of Henle granular cells, which secrete a protein called renin into the blood. (more on renin later) Mechanisms Regulating the GFR: Tubuloglomerular Feedback Mechanism (TGFM) The TGFM is based on the flow rate of the filtrate through the distal tubule. Efferent arteriole Bowman’s capsule If GFR increases above Distal tubule 1 180L/day. Glomerulus Macula densa Flow of filtrate through tubule 4 2 1 increases. 5 Afferent Flow of filtrate past macula densa 3 increases. arteriole 3 Granular 2 Paracrine signal diffuses from Proximal 4 cells macula densa to afferent tubule arteriole. Paracrine signal causes afferent 5 arteriole to constrict. Resistance to blood flow in afferent Blood flow into arteriole increases Collecting glomerulus duct decreases Hydrostatic pressure in glomerulus decreases. Loop of Henle GFR decreases back toward 180L/day. Mechanisms Regulating the GFR: ANS Influence on the GFR Normally, the ANS has little influence on the GFR compared to the autoregulatory mechanisms. ANS influence on the GFR is mediated by the sympathetic subdivision of the ANS. The smooth muscle of both afferent and efferent arterioles have α receptors which receive sympathetic innervation, and act to regulate the vasoconstriction of these arterioles. If blood pressure drops sharply due to loss of blood or dehydration, the CNS will increase sympathetic output in an effort to maintain a normal blood pressure. This causes vasoconstriction of both afferent and efferent arterioles, thus decreasing blood flow through the glomerulus. This will adjust GFR with the goal of decreasing fluid loss through urine and so maintaining blood volume which will help in maintaining blood pressure. Mechanisms Regulating the GFR: Hormones A variety of hormones influence the GFR. The most important ones are Angiotensin II (AGII) and prostaglandins. The levels of these hormones influence the GFR by altering the surface area for filtration of the glomerular-Bowman’s capsule interface. Exactly how this is achieved is an active area of research. AGII is also a potent vasoconstrictor. AGII is also involved in regulation of fluid and electrolyte balance in the body (more on this later). Nephron Functions: Filtration, Reabsorption, Secretion, Excretion Once the filtrate is formed in Bowman’s Capsule it must be processed or else important nutrients (ex. amino acids, glucose) and water would be lost in the urine. Since the bulk filtration produces 180L/day of filtrate, we would have to be constantly drinking to replace the lost fluid or our body would quickly dehydrate. So, as it passes from Bowman’s capsule through the rest of the nephron, reabsorption and secretion of solutes occurs before the filtrate is excreted into the renal pelvis as urine. Nephron Functions: Reabsorption Reabsorption is the movement of water and solutes from the filtrate back into the blood. Reabsorption occurs in the PT, Loop of Henle, DT, and Collecting Duct. Efferent Peritubular capillaries Distal arteriole tubule S R Glomerulus F cortex Afferent R S arteriole Bowman’s Proximal medulla capsule tubule Most reabsorption of water and R Hormonally S solutes occurs as the filtrate R R regulated passes through the Proximal reabs of water tubule. Loop and Na+, and KEY of secretion of K+ Henle F = Filtration: blood to lumen To R = Reabsorption: lumen to blood Collecting renal duct vein S = Secretion: blood to lumen E E = Excretion: lumen to external Selective reabsorption of water and (urine) environment some ions (Na+, K+, Cl-) To bladder and external environment Nephron Functions: Reabsorption Two types of reabsorption occur in the kidney tubules: 1. Bulk reabsorption: Takes place in the proximal tubule and loop of Henle. Goal: recovery of water, amino acids, glucose, and Na+ ions from the filtrate. Nephron Functions: Reabsorption 2. Regulated reabsorption: Takes place in the distal tubule and collecting duct. Regulated by hormones. Goal: selective reabsorption of water and Na+ ions from the filtrate. Nephron Functions: Reabsorption As the filtrate moves through the proximal tubule, about 75-80% of the volume is reabsorbed back into the interstitial fluid of the kidney. Because the concentration of solutes in the filtrate is the same as in the interstitial fluid, there is no concentration gradient to move solutes out of the filtrate into the interstitial fluid. So, active transport mechanisms are required (i.e. mechanisms that require the expenditure of energy.) Nephron Functions: Reabsorption Four types of active transport mechanisms are used by the epithelial cells that make up the walls of the proximal tubule: 1. Primary active transport Transport of the solute requires the direct expenditure of ATP to move the solute from the filtrate to the interstitial fluid. Na+ ions are reabsorbed from the filtrate by this mechanism. Nephron Functions: Reabsorption- Primary Active Transport Because Na+ is in high concentration in the filtrate relative to inside the epithelial cells, it can move down its concentration Tubule Proximal tubule cell Interstitial lumen gradient into the cells (1). Na+ utilizes fluid transporters in the apical membrane of Na+ the cells, or it reabsorbed Na+ enters cell can also move through leak channels. through various [Na+] membrane proteins, [Na+] [Na+] low moving down its high high electrochemical Once it is inside the cell, Na+ is gradient. actively transported across the basal membrane and into the interstitial fluid by ENaC the Na+-K+ pump (2). Because Na+ is in Na+ is pumped out the relatively high concentration in the basolateral side of ATP cell by the interstitial fluid, it requires energy to Na+-K+-ATPase pump. Na+ move it across the basal membrane of the Na+ cell. This energy comes from the ions K+ Apical hydrolysis of ATP. ions Basal K+ Leak channel Nephron Functions: Reabsorption- Secondary Active Transport 2. Secondary Active Transport The energy required for transport of the solute comes from hydrolysis of ATP used in moving another solute (usually Na+). Glucose is a solute reabsorbed by this mechanism. All glucose is reabsorbed as the filtrate passes through the proximal tubule. Reabsorption of glucose is dependent on the co- transport of Na+ Others solutes reabsorbed by secondary active transport are amino acids, some ions, and various organic molecules Nephron Functions: Reabsorption- Secondary Active Transport Glucose is in low concentration in the filtrate relative to inside the cells. Therefore, to move glucose across the apical membrane against its concentration gradient requires energy. Na+ moving down its Glucose electro- This energy comes from the movement of Na+ down[Na+] high [Na+] low chemical gradient and Na+ [glu] high reabsorbed uses the [glu] low its concentration gradient. The SGLT transport protein SGLT protein to pull (1) in apical membrane is glucose into the cell against able to capture some of the energy from the SGLT its [glu] low movement of Na+ down its concentration concentration glu GLUT gradient. gradient and use that energy to move glucose into the cell. Glucose diffuses Na+ Once inside the cell, glucose is moved down [Na+] out the high basolateral side of its concentration gradient across the basal membrane ATP the cell by a transport protein called using the GLUT K+ protein. GLUT (2). No energy is required for this. Na+ is moved across the basal membrane K+ Leak channel by the Na+-K+ pump. This requires energy Na+ is pumped out by from the hydrolysis of ATP (3). Apical Na+-K+-ATPase membrane Basolateral pump. membrane Nephron Functions: Reabsorption- Secondary Active Transport Note: The energy for moving glucose across the apical membrane comes from the movement of Na+ down its concentration gradient. Without this energy, glucose could not be reabsorbed. The movement of Na+ across the basal membrane is critical for this mechanism to work. If the Na+ is not moved out of the cell into the interstitial fluid, this mechanism would shut down, because the Na+ concentration gradient between the filtrate and inside of the cell would not be maintained and there would be no energy to move glucose across the apical membrane. So, the energy for moving glucose comes “secondarily” from the expenditure of energy by the Na+-K+ ATPase pump. Nephron Functions: Reabsorption- “Passive” Reabsorption 3. “Passive” Reabsorption: Used to reabsorb some of the urea in the filtrate. Cell membranes are highly permeable to urea. There are no active transport proteins that move urea across membranes. Urea is in the same concentration in the filtrate as it is in the interstitial fluid. So, no concentration gradient exists to move urea out of the filtrate. So, to reabsorb urea from the filtrate, a concentration gradient for urea has to be created. Nephron Functions: Reabsorption- “Passive” Reabsorption Creation of a Urea Concentration Gradient: As other solutes (Na+ , etc) are reabsorbed, the filtrate becomes less concentrated than the Interstitial Fluid (IF) This creates an osmotic gradient that draws water out of the filtrate and into the IF. As the volume of the filtrate decreases, the concentration of urea in the filtrate increases relative to the concentration of urea in the IF. Because cell membranes are permeable to urea, the urea in the filtrate diffuses across the epithelial cells into the IF down the concentration gradient. Note: Energy is required to move the other solutes out of the filtrate, so the process is not really as passive as the name “passive” reabsorption implies. Nephron Functions: Reabsorption- Transcytosis 4. Transcytosis: Involves the transport of solutes by vesicles. Used to reabsorb any small protein that may have leaked into the filtrate across the wall of the Bowman’s Endocytosis capsule during filtration. Transcytosis mostly occurs in the proximal tubule. transport Protein receptors on the apical membrane bind small proteins that Exocytosis are in the filtrate. A vesicle forms by endocytosis. Vesicle is transported across the cell. Contents are released into the IF as digested (amino acids) or intact proteins by exocytosis. Nephron Functions: Reabsorption The solutes that are reabsorbed from the filtrate in the proximal tubule are taken up into the blood in the peritubular capillaries and carried out of the kidneys by the venous circulation. These reabsorption transport mechanisms can become saturated (i.e. overwhelmed) if there is too much of a given solute in the filtrate. So, in this case, the solute will pass into the urine. Ex: Diabetes Mellitus (“sweet urine”) Diabetes Mellitus Normally all the glucose that passes into the filtrate in Bowman’s capsule is reabsorbed as the filtrate passes through the proximal tubule. So, there is no glucose in the urine. In D.M., the glucose levels rise so high that the Na+ - glucose co-transporter can’t reabsorb all of the glucose from the filtrate. So, some of the glucose passes into the urine and is excreted. The presence of this sugar in this sugar in the urine makes the urine “sweet”. Nephron Functions: Filtration, reabsorption, secretion, and excretion Peritubular capillaries Distal tubule Efferent arteriole S Glomerulus R F cortex R S Afferent arteriole Bowman’s Proximal Renal artery (20% of capsule tubule medulla R afferent arteriole plasma filters into S Hormonally BC). R R regulated reabs of water and Na, Loop and secretion KEY of of K Henle F = Filtration: blood to lumen To R = Reabsorption: lumen to blood Collecting renal duct vein S = Secretion: blood to lumen E E = Excretion: lumen to external Selective reabsorption of (urine) environment water and some ions (Na, K, To bladder and Cl) external environment Nephron Functions: Secretion Secretion is the active movement of solutes from the interstitial fluid into the filtrate in the nephron lumen. The secretion of K+ and H+ is important in homeostatic regulation of these ions in the body fluids. Secretion allows the concentration of solutes in the filtrate beyond what occurs as a result of filtration. Enables the nephron to enhance excretion of a substance. Relies on active transport of solutes across the membrane (K+, H+, drugs, and organic anions) using similar transport mechanisms to those in reabsorption. Nephron Functions: Secretion Organic anions secreted into the filtrate by the proximal tubule include bile salts, salicylate (from aspirin), and the artificial sweetner saccharine. Proximal tubule secretion of organic anions by the organic anion transporter (OAT) is an example of tertiary active transport (use of energy from the hydrolysis of ATP is two steps removed from the OAT. KG- alpha ketogluterate OA- organic anion Tubule lumen Proximal tubule cell Interstitial fluid Direct active transport. The Na+-K+-ATPase keeps intracellular [Na+] low. [Na+] low K+ K+ ATP Secondary indirect active transport. Na+ Na+ Na+ The Na+-dicarboxylate cotransporter Filtrate NaDC (NaDC) concentrates a dicarboxylate KG– inside the cell using energy stored in NaDC the [Na+] gradient. Metabolism KG– KG– concentrates Tertiary indirect active transport. A– KG– The basolateral organic anion OAT transporter (OAT) concentrates Organic anions OA– OA– OA– organic anions (OA–) inside the enter the lumen cell, using the energy stored in the by facilitated dicarboxylate gradient. diffusion. Apical Basolateral membrane membrane Nephron Functions: Excretion Excretion is the elimination of solutes from the body in the urine. Composition of the urine being excreted = filtration – reabsorption + secretion It’s what’s left in the filtrate after it has passed through the nephron. All the glucose, amino acids, and useful metabolites are gone, and organic wastes and other solutes are more concentrated. Excretion tells us what the nephrons are eliminating, but not the details of how the nephrons are handling a specific solute, i.e. whether the solute is being reabsorbed or secreted. Excretion rate of a solute depends on: Its Filtration rate into Bowman’s capsule and whether the substance is reabsorbed, secreted, or both as it passes through the tubule. Nephron Functions: Excretion Renal Clearance of a solute can tell you how the tubules are handling a particular solute, i.e. whether it is being completely reabsorbed, partially reabsorbed, partially secreted, or completely secreted. Renal Clearance (C) = the volume of plasma cleared of a solute per minute. Calculated using the formula: = urine excretion rate of solute X (UERX) / Plasma concentration of solute X = ml of plasma cleared of solute X per minute Inulin is a polysaccharide that we know is: Non-toxic when injected into the blood Freely filtered across the wall of Bowman’s capsule. Not absorbed or secreted (100% excreted), so Cinulin = GFR, the clearance of inulin can be used to determine a person’s GFR. Nephron Functions: Excretion Example of calculating Clearance of a solute: Inject a subject with inulin to get a known plasma concentration of 100mg inulin/ 100ml of plasma. Collect the subject’s urine over a period of time, and measure the amount of inulin in the urine collected per minute (UERinulin) = 125mg/min. Since, C = UERx / Plasma concentration of solute X, then C inulin= (125mg/min) / (100mg/100ml) So, Cinulin =125ml of plasma is cleared of inulin/minute. Nephron Functions: Excretion Since we know inulin is freely filtered and neither reabsorbed nor secreted, the clearance of inulin equals the GFR. So, our subject’s GFR = 125ml/min (which is normal). Knowing the GFR allows us to calculate the filtration rate (FR) of a solute. The FR of a solute (X) is the amount of the solute filtered out of the plasma by the kidneys per unit time (expressed as mg/min). FRX = (plasma concentration of solute X) * (GFR) So, to determine the FR of a solute: We inject our subject with solute X so that the plasma concentration of X = 100mg of X / 100ml of plasma And our subject’s GFR is = 125ml plasma/min (which we determined previously using inulin) Nephron Functions: Excretion Then taking our formula for calculating FR of a solute FRX = (plasma concentration of solute X) * (GFR) = (100mg of X / 100ml plasma) * (125ml plasma/min) = 125mg of solute X /min So, 125mg of solute X is filtered from our subject’s plasma by the kidneys per minute. Nephron Functions: Excretion Suppose we have a solute Y that we previously determined is freely filtered from the plasma into Bowman’s capsule. To determine how the kidney tubules are handling solute Y, we inject our subject with solute Y so that its plasma concentration is 100mg of Y/100ml plasma. Collect our subject’s urine over a period of time and determine that UERY = 0 mg per minute, i.e. no Y appears in the urine. This tells you that Solute Y is completely reabsorbed from the filtrate by the kidney tubules, and there is no secretion of Y into the filtrate. Nephron Functions: Excretion On the other hand, Suppose we inject our subject with solute Y so that its plasma concentration is 100mg of Y/100ml plasma. Collect our subject’s urine over a period of time and determine UERY = 125 mg of Y/ min. Since Y is freely filtered we can calculate the FRY using the formula: (plasma conc of Y) * (GFR) (100mg/100ml) * (125ml/min) = 125mg of Y/min. So, UERY = FRY This tells you that solute Y is neither secreted nor reabsorbed from the filtrate by the kidney tubules. Nephron Functions: Excretion On the other hand Suppose we inject our subject with solute Y so that its plasma concentration is 100mg of Y/100ml plasma. Collect our subject’s urine over a period of time and determine that the UERY = 130 mg of Y/minute. Since Y is freely filtered we can calculate the FRY using the formula: (plasma conc of Y) * (GFR) (100mg/100ml) * (125ml/min) = 125mg of Y/min. So, UERY > FRY This tells you that solute Y is being actively secreted into the filtrate. Nephron Functions: Excretion On the other hand Suppose we inject our subject with solute Y so that its plasma concentration is 100mg of Y/100ml plasma. Collect our subject’s urine over a period of time and determine that the UERY = 90 mg of Y/min. Since Y is freely filtered we can calculate the FRY using the formula: (plasma conc of Y) * (GFR) (100mg/100ml) * (125ml/min) = 125mg of Y/min. So, UERY < FRY This tells you that some, but not all, of solute Y is reabsorbed by the kidney tubules from the filtrate, i.e. solute Y is being partially reabsorbed. Nephron Functions: Excretion So, if you know a subject’s: GFR Plasma concentration of a solute in the subject’s plasma Urine Excretion Rate of the solute You should be able to calculate Clearance and Filtration Rate of the solute, and be able to determine how the kidney tubules are handling the solute, i.e. whether it the solute is being reabsorbed or secreted by the kidney tubules. Chapter 20 Fluid and Electrolyte Balance Water Balance in the Body Fluids The maintenance of water balance in the body is critical for homeostasis. Too much water in the body will cause swelling of cells and disrupt normal cell functions, too little water will cause cells to shrivel and also disrupt normal cell functions. Most water is taken into the body via the digestive system, and is lost mostly in the urine. The regulation of water balance in the body is achieved by regulation of urine concentration (osmolarity). When the body needs to get rid of excess water, the kidneys excrete large amounts of dilute urine with low osmolarity around 50mOsM. When the body needs to retain water (ex. If you are dehydrated), the kidneys will excrete a lower volume of more concentrated urine (around 1200mOsM). Note: Normal body fluid osmolarity =300mOsM Water Balance in the Body Fluids The kidneys regulate the concentration of the urine by varying the amount of water and Na+ reabsorbed from the filtrate in the nephron. To produce dilute urine, the nephron must reabsorb solute without allowing water to follow by osmosis. So the cell membranes across which the solute is transported must be impermeable to water. To produce a concentrated urine, the nephron must reabsorb water from the filtrate while leaving solute in the filtrate. Water Balance in the Body Fluids Water in the kidneys is reabsorbed only by osmosis. Osmosis will not take place unless a concentration gradient exists. Water will flow from fluids of LOW osmolarity to fluids of HIGH osmolarity. Therefore, the renal medulla maintains a high osmotic concentration in its interstitial fluid that can be used to osmotically pull water out of the filtrate. Water Balance in the Body Fluids and Urine Concentration The osmolarity of the filtrate changes as filtrate flows through the nephron. The osmolarity of the filtrate in proximal tubule is the same as the osmolarity of the interstitial fluid of the kidney cortex (300mOsM). The loop of Henle first concentrates the filtrate by removing water and then dilutes it by removing Na+, K+, and Cl- ions. This figure shows how the loop of Henle works. Walls of descending limb are permeable to water & impermeable to Na+, Cl-, K+. Water is pulled out by increasing osmotic gradient in the medulla and filtrate osmolarity increases to Descending limb 1200mOsM. Walls of ascending limb are impermeable to water & actively transport Na+, Cl-, K+ Ascending out of the filtrate. Filtrate limb osmolarity decreases to 100mOsM. Water Balance in the Body Fluids and Urine Concentration To maintain the concentration gradient in the kidney medulla the water that is reabsorbed must be removed from the interstitial fluid. This is achieved by a countercurrent exchange system Descending involving the peritubular capillaries limb associated with the loop of Henle. This part of the peritubular capillaries is called the vasa recta. Blood flow through the vasa recta is opposite to the flow of filtrate through the loop of Henle (the respective flows are counter to one another). The blood flowing down into the medulla picks up the Na+, K+, and Cl- Ascending reabsorbed from the ascending limb. As it limb flows back up the blood picks up the water reabsorbed from the descending limb. Water Balance in the Body Fluids and Urine Concentration If the vasa recta didn’t remove the water from the interstitial fluid of the kidney medulla, the concentration gradient in the medulla would be diluted and the loop of Henle wouldn’t be able to function. Just as important, water wouldn’t be able to be reabsorbed from the filtrate as it moved down the collecting duct and resulting in dehydration of the body fluids. (more on this in a minute) Water Balance in the Body Fluids and Urine Concentration There are 2 physiological reasons for producing a filtrate that is more dilute than the interstitial fluid of the body: 1. If urine was excreted with a concentration of 1200mOsM, you’d lose a lot of Na+, K+, Cl-. These ions are needed for a variety of physiological functions (remember membrane potential?). 2. By producing a dilute filtrate, you can reabsorb water from the filtrate if you need to conserve water, or you can get rid of excess water by not reabsorbing it from the filtrate and allowing it to excreted in the urine. Water Balance in the Body Fluids and Urine Concentration The ability of the nephron to regulate the concentration of the urine that is excreted depends on two factors: The variable water permeability of the walls of the distal tubule and collecting duct Osmotic gradient in the interstitial fluid of the kidney medulla. This osmotic gradient can be used for reabsorbing water from the filtrate as it passes down the collecting duct. Water Balance in the Body Fluids and Urine Concentration: Hormonal Regulation The hormone vasopressin (AKA: Antidiuretic Hormone, ADH) levels in the blood regulates the water permeability of the walls of the distal tubule and collecting duct. ADH is a protein hormone synthesized by neurons in the hypothalamus and secreted from the posterior pituitary gland. ADH acts on the epithelial cells to stimulate the synthesis and insertion of water pores into to the apical membrane of these cells allowing the movement of water out of the filtrate by osmosis. Water Balance in the Body Fluids and Urine Concentration: Hormonal Regulation The higher the blood plasma levels of ADH the greater the number of water pores in the apical membrane of the epithelial cells and Increasing osmotic gradient the more permeable the walls of the distal tubule and collecting duct are to water the more water that is reabsorbed from the filtrate the more concentrated the urine excreted. Water Balance in the Body Fluids and Urine Concentration: Hormonal Regulation The lower the blood plasma levels of ADH the fewer cortex the water pores in the apical membrane of the epithelial medulla cells and the more Increasing osmotic gradient impermeable the walls of the distal tubule and Collecting collecting duct are to water duct the more water that remains in the filtrate and passes into the urine the more dilute the urine excreted. Water Balance in the Body Fluids and Urine Concentration: Hormonal Regulation This figure summarizes the action of ADH on epithelial cells of the distal tubule and collecting duct. Cross-section of kidney tubule Collecting Medullary Vasa Collecting duct cell duct interstitial recta lumen fluid blood Filtrate 600 mOsM 300 mOsm 600 mOsM 700 mOsM Note: water pore already present in basal membrane Interstitial fluid 1 Vasopressin Lumen : Filtrate (ADH) Vasopressin (ADH) receptor 1 Vasopressin binds to mem- brane receptor. Water Balance in the Body Fluids and Urine Concentration: Hormonal Regulation Cross-section of kidney tubule Collecting Medullary Vasa Collecting duct cell duct interstitial recta lumen fluid Filtrate 600 mOsM 300 mOsm 600 mOsM 700 mOsM blood Second 2 messenger Interstitial signal fluid 1 cAMP Vasopressin Lumen : Filtrate Vasopressin receptor 1 Vasopressin 2 Receptor activates binds to mem- cAMP second brane receptor. messenger system. Water Balance in the Body Fluids and Urine Concentration: Hormonal Regulation Cross-section of kidney tubule Collecting Medullary Vasa Collecting duct cell duct interstitial recta lumen fluid Filtrate 600 mOsM 300 mOsm 600 mOsM 700 mOsM Storage vesicles blood Second 2 messenger Interstitial Exocytosis system fluid of vesicles 1 Aquaporin-2 cAMP Vasopressin Lumen : Filtrate 3 water channels Vasopressin receptor 1 Vasopressin 2 Receptor activates 3 Cell inserts AQP2 binds to mem- cAMP second water pores into brane receptor. messenger system. apical membrane. Water Balance in the Body Fluids and Urine Concentration: Hormonal Regulation Cross-section of kidney tubule Collecting Medullary Vasa Collecting duct cell duct interstitial recta lumen fluid Filtrate 600 mOsM H2O 300 mOsm 600 mOsM H2O H2O H2O 4 700 mOsM Storage vesicles blood Second 2 messenger Interstitial Exocytosis signal fluid of vesicles 1 Aquaporin-2 cAMP Vasopressin Lumen : Filtrate 3 water pores Vasopressin receptor 1 Vasopressin 2 Receptor activates 3 Cell inserts AQP2 4 Water is absorbed binds to mem- cAMP second water pores into by osmosis into brane receptor. messenger system. apical membrane. the blood. Water Balance in the Body Fluids and Urine Concentration: Hormonal Regulation Three factors regulate the release of ADH from the posterior pituitary. Of these three, plasma osmolarity is the most potent stimulus *. Normal blood osmolarity = 280 - 1 * 2 3 300mOsM Electrolyte Balance in the Body Fluids As well as regulation of water balance, regulation of Na+ and K+ secretion and reabsorption by the kidney tubules is also very important in regulating blood and body fluid concentrations. Na+ is most abundant ion in Interstitial Fluid (IF), and is the primary factor determining concentration of IF and blood. K+ is most abundant ion in the intracellular fluids. As we have seen, these ions are crucial in the functioning of neurons, and muscle tissue including the heart. So maintaining proper levels of these ions in the body fluids is important. Electrolyte Balance in the Body Fluids Na+ reabsorption from filtrate is primarily regulated by the steroid hormone Aldosterone. Aldosterone is synthesized and secreted by cells in the cortex of the adrenal glands. Aldosterone regulates Na+ reabsorption from filtrate in the distal tubules and collecting ducts. The target cells of Aldosterone are the Principal Cells (P- cells) in the walls of the distal tubules and collecting ducts. Electrolyte Balance in the Body Fluids: Aldosterone This figure summarizes the actions of aldosterone on P-cells. P cell of distal nephron Interstitial Blood 1 Aldosterone fluid combines with a cytoplasmic receptor. Aldosterone combines Lumen with cytoplasmic Aldostrone is a receptor of distal steroid hormone so tubule 1 Aldosterone it can cross cell Aldosterone cytoplasmic membranes and receptor bind to cytoplasmic receptors. Electrolyte Balance in the Body Fluids: Aldosterone P cell of distal nephron Interstitial Blood 1 Aldosterone fluid combines with a cytoplasmic receptor. Lumen 2 Hormone-receptor of distal complex initiates Transcription tubule Aldosterone transcription in 2 1 the nucleus. mRNA Aldosterone receptor Electrolyte Balance in the Body Fluids: Aldosterone P cell of distal nephron Interstitial Blood 1 Aldosterone fluid combines with a cytoplasmic receptor. Lumen 2 Hormone-receptor of distal complex initiates Transcription tubule Aldosterone transcription in 2 1 the nucleus. mRNA 3 Translation and Aldosterone protein synthesis receptor New protein 3 channels New and pumps Na+ and K+ New Na+/K+ are made and inserted into channels are pumps are made ATP the cell membrane made and and inserted into inserted into the basal apical membrane membrane Electrolyte Balance in the Body Fluids: Aldosterone P cell of distal nephron Interstitial Blood 1 Aldosterone fluid combines with a cytoplasmic receptor. Lumen 2 Hormone-receptor of distal complex initiates Transcription tubule Aldosterone transcription in 2 1 the nucleus. mRNA 3 Translation and Aldosterone protein synthesis receptor New protein 3 channels New and pumps New pumps are made and inserted channels ATP 4 into the cell membrane Proteins are made that modulate Aldosterone- activity of channels and pumps 4 induced proteins are made that modulate activity of ATP channels and pumps Electrolyte Balance in the Body Fluids: Aldosterone Net result = increased Na+ reabsorption from filtrate and increased K+ secretion into filtrate. P cell of distal nephron Interstitial Blood 1 Aldosterone fluid combines with a cytoplasmic receptor. Lumen 2 Hormone-receptor of distal complex initiates Transcription transcription in tubule Aldosterone 2 1 the nucleus. mRNA 3 Translation and Aldosterone protein synthesis receptor New protein 3 channels New and pumps channels New pumps are made and inserted ATP into the cell membrane 4 Proteins modulate Aldosterone- Channels and pumps. 4 induced K+ K+ proteins are made that secreted K+ K+ 5 modulate activity of ATP channels and pumps Na+ Na+ reabsorbed Na+ 5 Result is increased Na+ reabsorption and K+ secretion. Na+ Electrolyte Balance in the Body Fluids: Factors that Regulate Aldosterone Synthesis Two primary factors are responsible for stimulating the synthesis of aldosterone: K+ concentrations in the Interstitial Fluid above normal levels. A decrease in blood pressure below mean arterial pressure. Electrolyte Balance in the Body Fluids: Factors that Regulate Aldosterone Synthesis K+ concentration in the IF: Increased K+ concentrations above normal levels in the IF directly stimulates the endocrine cells of the adrenal gland to synthesize aldosterone. The increased aldosterone levels in the blood increases K+ secretion and Na+ reabsorption in the distal tubules and collecting ducts. This serves to bring K+ levels down to normal and increases Na+ levels. Electrolyte Balance in the Body Fluids: Factors that Regulate Aldosterone Synthesis Blood pressure regulates aldosterone synthesis through a complex pathway called the Renin-Angiotensin-Aldosterone System (RAAS). Juxtaglomerular apparatus RAAS is initiated converts by a drop in BP below MAP converts Has 5 affects Proximal tubule Negative feedback Electrolyte Balance in the Body Fluids: Atrial Natriuretic Peptide (ANP) Another hormone involved in both water balance and Na+ regulation is Atrial Natriuretic Peptide (ANP). ANP is a protein hormone synthesized and secreted by myocardial cells of the atria. Synthesis and secretion of ANP is stimulated by an increase in stretch in the walls of the atria resulting from an increase in blood volume above normal. ANP acts to increase both water and Na+ excretion in the urine. Electrolyte Balance in the Body Fluids: Atrial Natriuretic Peptide (ANP) Actions of ANP: ANP acts on the adrenal glands to decrease synthesis of aldosterone. ANP acts on the hypothalamus to decrease the synthesis and release of ADH. ANP acts on the cardiovascular control center in the medulla oblongata to alter the sympathetic/parasympathetic balance to reduce blood pressure. ANP has 2 effects on kidneys: Increase GFR by vasodilating the afferent arteriole increasing blood flow into the glomerulus. Inhibits the release of renin. The net effect of the actions of ANP is to promote water and Na+ excretion in urine, which serve to decrease blood volume back towards normal. Electrolyte Balance in the Body Fluids: Acid-Base Balance in Body Fluids The kidneys are also involved in regulating acid-base balance in the body fluids. Normal Body fluid pH = 7.4 3 mechanisms regulate body fluid pH: 1. Lung ventilation (main mechanism regulating acid-base balance) CO2 + H2O H2CO3 H+ + HCO3- Decreased lung ventilation = increases CO2 in blood = decreased pH of blood Increased lung ventilation = decreased CO2 in blood = increased pH in blood Electrolyte Balance in the Body Fluids: Acid-Base Balance in Body Fluids 2. Buffers in body fluids HCO3- is main buffer in body fluids. Mostly comes from CO2 transport system in blood. 3. Secretion and reabsorption of H+ and HCO3- by the kidneys. If pH of body fluids falls below 7.4 the kidney tubules will secrete H+ and reabsorb HCO3- to bring the pH of the body fluids back up. If pH of body fluids rises above 7.4 the kidney tubules will reabsorb H+ and secrete HCO3-, bringing pH of body fluids back down. Electrolyte Balance in the Body Fluids: Acid-Base Balance in Body Fluids Book details the transport mechanisms involved in reabsorption/secretion of H+ and HCO3-. You will not be held responsible for these mechanisms. This completes the material on the renal system.