Reach For Stars 2025 PDF
Document Details
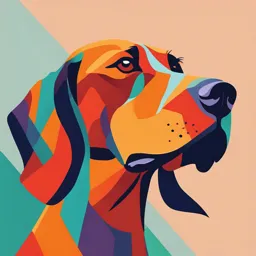
Uploaded by ConsiderateGlockenspiel5007
Webb Bridge Middle School
2025
Tags
Summary
This document provides a comprehensive overview of various stellar objects, including pulsars, red giants, and supernovae. It describes their properties, relationships to each other, and evolution through the different stages of their life cycles.
Full Transcript
**Aquila** **Hulse Taylor pulsar** On a standard H-R diagram, the Hulse-Taylor pulsar (PSR B1913+16) would appear far to the left and slightly above the main sequence, representing its extremely high temperature and relatively low luminosity as a neutron star, Key points about the Hulse-Taylor pul...
**Aquila** **Hulse Taylor pulsar** On a standard H-R diagram, the Hulse-Taylor pulsar (PSR B1913+16) would appear far to the left and slightly above the main sequence, representing its extremely high temperature and relatively low luminosity as a neutron star, Key points about the Hulse-Taylor pulsar on an H-R diagram: **Location:** Extremely far to the left on the diagram due to its high temperature as a neutron star  **Canis Major** **Sirius** Luminosity = 25.4 times spectral class A Constellation Canis Major \| Information \... **Cassiopeia** **Cassiopeia A** Cassiopeia A would appear far to the upper left, positioned as a very luminous and hot object, signifying its status as a supernova remnant with extremely high temperature due to the rapidly expanding debris from the stellar explosion  Cassiopeia A - Wikipedia **Cetus** **Mira** more than 8,000 times more luminous M2- M7 A constellation of stars in the sky Description automatically generated **Cygnus** **Cygnus X-1** **SS Cygni** Ss Cygni is a star and cygnus x-I was a blackhole. Cygnus X-1 be classified as companion star, a blue supergiant classified as an OB star (HDE 226868), would appear on the diagram as a very hot, luminous star located high on the main sequence due to its high mass and temperature. SS Cygni is 40 times brighter than the sun and is an K5 spectral class.  Artist\'s impression of Cygnus X-1 \| ESA \... **Dorada** **SN 1987A** the progenitor star of Supernova 1987A would be located near the blue supergiant region, indicating a massive, hot star that evolved rapidly towards the end of its life before exploding; its path on the H-R diagram would show a \"blue loop\" evolution, moving slightly towards the red giant branch before returning to hotter temperatures due to stellar winds and mass loss, ultimately ending as a supernova when it reached the core collapse stage Constellation Dorado - The \... **Draco** **NGC 6543**  NGC 6543, also known as the \"Cat\'s Eye Nebula,\" would appear as a very hot, highly luminous point located far to the left and slightly above the main sequence, representing the central star of the nebula which is a white dwarf in the final stages of its stellar evolution **Lyra** **RR Lyrae** 40 to 50 times more luminous than the Sun A or F  **Ophiuchus** **SN 1604** On an H-R diagram, SN 1604, also known as Kepler\'s Supernova, would appear as a very bright point located far to the upper left  **Orion** **Betelgeuse** 7,600 to 14,000 times that of the Sun M2 lab  Betelgeuse: A guide to the giant star \... **Southern Hemisphere** **GW 150914**  - - **Structure, Physical Properties and Behavior of Red Giant Branch** - **Structure of Red Giants:** A red giant branch (RGB) star is structured with a central, inert helium core surrounded by a shell where hydrogen is actively fusing into helium, causing the star to expand significantly and become very luminous, appearing red in color; this hydrogen-burning shell is the key feature of a star on the red giant branch, marking the stage after it has left the main sequence in its stellar evolution. **Inert helium core:** The core of a red giant branch star is primarily composed of helium that is not currently undergoing fusion, meaning it is not producing energy through nuclear reactions. **Hydrogen-burning shell:** The energy production in a red giant branch star comes from a thin shell surrounding the helium core where hydrogen is fusing into helium through nuclear reactions. **Large envelope:** The outer layers of the star expand significantly, creating a large, cool envelope that gives the star its red appearance. **Physical Properties of Red Giants:** A red giant branch star is characterized by a very large radius (up to 200 times that of the Sun), a cool surface temperature (around 3,000 - 4,000 Kelvin), a high luminosity (up to thousands of times the Sun\'s luminosity), and a spectral type of K or M, indicating its red color; it has an inert helium core surrounded by a shell of hydrogen actively fusing through the CNO cycle, causing its expansion and low surface temperature. **Behavior of Red Giants:** Stars behave in a number of ways as they move along the red giant branch (RGB), including Increasing luminosity: As the hydrogen shell produces more helium, the star\'s core increases in temperature and mass, causing the star to become more luminous. Increasing size: The star\'s outer convective envelope deepens as it grows, causing the star to become larger. Cooling: The star\'s surface temperature decreases as its luminosity increases. Dredge-up: The star\'s outer convective envelope can reach deep enough to bring fusion products to the surface, changing the surface abundance of certain elements. RGB bump: A noticeable clustering of stars on the RGB caused by a discontinuity in hydrogen abundance. Helium flash: A short thermonuclear runaway event that occurs when the star\'s core reaches temperatures to fuse helium. This event injects enough energy to power a rapid evolution off the RGB. Moving to the horizontal branch: After the helium flash, the star moves along the horizontal branch. The evolutionary path a star takes along the RGB depends on its mass. For example, stars with a mass less than about 2 M ☉ will eventually reach a core temperature hot enough to begin fusing helium to carbon**.** - **Structure, Physical Properties and Behavior of Asymptotic Giant Branch** - An Asymptotic Giant Branch (AGB) star is a late-stage stellar evolution phase where a star has a complex internal structure with a dense, inert core of carbon and oxygen, surrounded by a helium-burning shell, a hydrogen-burning shell further out, and a very large, cool, extended outer envelope, leading to a bright red giant appearance with high luminosity and significant mass loss due to strong stellar winds; this phase is characterized by recurrent thermal pulses that mix elements from the core to the surface, contributing to the production of heavy elements through nucleosynthesis. **Key structural features of an AGB star: Central Core:** A largely inert core composed primarily of carbon and oxygen, which is highly dense and electron-degenerate. **Helium Burning Shell:** A thin shell surrounding the core where helium is fusing into carbon, providing the primary energy source during the AGB phase. **Intershell Region:** A layer between the helium burning shell and the hydrogen burning shell, enriched with helium and carbon, where convective mixing can occur. **Hydrogen Burning Shell:** A further outer shell where hydrogen is fusing into helium. **Extensive Envelope:** A very large, cool, and tenuous outer layer of gas, which is primarily composed of hydrogen and other elements dredged up from the core through convection. **Physical properties and behavior: High Luminosity:** AGB stars are extremely luminous due to the energy produced by the shell burning, appearing as bright red giants on the Hertzsprung-Russell diagram. **Large Radius:** The extended envelope results in a significantly large stellar radius, making the star appear very cool and red. **Pulsational Variability:** AGB stars are known for their pulsations, causing fluctuations in brightness over time, which are observable as periodic variations in their light curves. **Mass Loss:** The strong stellar winds driven by pulsations lead to substantial mass loss, enriching the surrounding interstellar medium with heavy elements. **Nucleosynthesis:** The convective mixing during thermal pulses brings processed material from the helium burning shell to the surface, leading to the production of heavier elements through the slow neutron capture process (s-process). **Important points to remember about AGB stars:** They represent the final stages of stellar evolution for low to intermediate mass stars. The composition of the envelope can be significantly altered by the dredge-up process, leading to the formation of carbon stars. The study of AGB stars is crucial for understanding the origin of heavy elements in the universe. - **Structure, Physical Properties and Behavior of Planetary Nebulae** - Planetary nebulae are awe-inspiring celestial objects that mark the end of a star\'s life. They are formed when a star like our Sun exhausts its nuclear fuel, sheds its outer layers, and creates a beautiful, glowing shell of gas. Here\'s a breakdown of their structure, appearance, and behavior: **Structure**: **Central Star**: At the heart of a planetary nebula lies a hot, dense white dwarf star. This remnant star is the core of the original star that created the nebula. **Gaseous Shell**: The ejected outer layers of the star form a vast, expanding shell of gas. This shell is composed of various elements, including hydrogen, helium, carbon, nitrogen, and oxygen. **Halos and Jets**: Some planetary nebulae exhibit intricate structures like halos and jets. These features are thought to be caused by the interaction of the stellar wind from the central star with the surrounding gas. **Physical Appearance**: Planetary nebulae are known for their diverse and often stunning appearances. They can be nearly spherical, ring-shaped, bipolar (with two lobes), or even more complex shapes. The colors of planetary nebulae are determined by the elements present in the gas and the temperature of the central star. Common colors include red (hydrogen), blue (oxygen), and green (nitrogen). **Behavior**: **Expansion**: The gaseous shell of a planetary nebula is constantly expanding outward at high speeds, typically around 20-50 kilometers per second. **Ionization**: The intense ultraviolet radiation from the hot central star ionizes the gas in the nebula, causing it to glow. **Evolution**: Planetary nebulae are relatively short-lived phenomena, lasting only about 10,000 to 20,000 years. As the nebula continues to expand, it eventually disperses into the interstellar medium, enriching it with elements essential for the formation of new stars and planets. **Examples**: **Ring Nebula (M57):** A classic example of a ring-shaped planetary nebula located in the constellation Lyra. **Helix Nebula (NGC 7293**): A large and bright planetary nebula with a complex structure, resembling an eye. **Cat\'s Eye Nebula (NGC 6543):** A stunning planetary nebula with intricate patterns and multiple shells. Planetary nebulae are not only beautiful but also play a crucial role in the recycling of matter in the universe. They provide valuable insights into the late stages of stellar evolution and the chemical enrichment of galaxies. - **Structure, Physical Properties and Behavior of White Dwarfs** - **White Dwarfs**: The Stellar Remnants. White dwarfs are the remnants of stars like our Sun that have exhausted their nuclear fuel. They are incredibly dense objects, packing a mass comparable to our Sun into a volume roughly the size of Earth. This extreme density arises from the unique physical properties and behavior of these celestial bodies. **Structure:** A typical white dwarf consists of a dense core composed primarily of carbon and oxygen. Surrounding this core is a thin layer of helium, and often an even thinner outer layer of hydrogen. This layered structure is a result of the star\'s evolutionary history, as different elements settle into distinct layers due to their densities. **Physical Properties: Extreme Density**: White dwarfs are incredibly dense, with an average density of about 10\^6 grams per cubic centimeter. This is roughly equivalent to a ton per cubic inch! **Degenerate Matter**: The matter within a white dwarf is in a state known as \"degenerate matter.\" In this state, the electrons are so tightly packed that they behave differently than in ordinary matter. This unique state of matter allows white dwarfs to resist gravitational collapse, even without ongoing nuclear fusion. **High Temperature**: Despite their small size, white dwarfs are extremely hot, with surface temperatures ranging from tens of thousands to hundreds of thousands of degrees Celsius. This high temperature is a remnant of their formation process and gradually decreases over time as they radiate away their thermal energy. **Behavior: Cooling and Fading**: White dwarfs are essentially cooling stellar embers. They do not generate energy through nuclear fusion, so they gradually radiate away their heat and luminosity. Over billions of years, they slowly cool and dim, eventually becoming black dwarfs -- cold, dark remnants. **Chandrasekhar Limit**: There is a limit to the mass a white dwarf can attain, known as the Chandrasekhar Limit. If a white dwarf accumulates mass beyond this limit, it can undergo a catastrophic collapse, potentially leading to a supernova explosion or the formation of a neutron star. **Accretion**: In binary star systems, a white dwarf can accrete matter from a companion star. This accretion can lead to various phenomena, including nova eruptions and the formation of exotic objects like Type Ia supernovae. White dwarfs are fascinating objects that offer valuable insights into the evolution of stars and the ultimate fate of our Sun. Their unique properties and behavior continue to be studied by astronomers, providing clues to the mysteries of the cosmos. - **Structure, Physical Properties and Behavior of Neutron Stars** - A neutron star is an extremely dense object formed when a massive star collapses, composed primarily of neutrons packed so tightly together that their density rivals that of an atomic nucleus, resulting in incredibly strong gravitational fields, rapid rotation, and extremely powerful magnetic fields; key structural features include a layered crust with increasing neutron density towards the core, potentially containing exotic matter like quark-gluon plasma in the innermost region, while their observable behavior is often characterized by pulsations of radiation due to their magnetic field alignment with the rotation axis. **Key points about neutron stars: Structure: Outer Crust: **Primarily composed of atomic nuclei embedded in a sea of electrons, with increasing neutron richness as density increases.** Inner Crust: **A complex lattice structure of increasingly neutron-rich nuclei, sometimes described as a \"pasta\" phase with formations like spaghetti and lasagna-like sheets. **Outer Core: **Mostly composed of neutrons with a small proportion of protons, where the matter is essentially a uniform superfluid. **Inner Core: **The composition is highly theoretical, potentially containing exotic matter like quark-gluon plasma, hyperons, or other exotic particles depending on the star\'s mass. **Physical Properties: Extreme Density: **Neutron stars are among the densest objects in the universe, with a teaspoon of material weighing billions of tons. **Strong Magnetic Fields: **Magnetic fields billions of times stronger than Earth\'s, influencing the star\'s emission patterns. **Rapid Rotation: **Neutron stars often spin very quickly due to the conservation of angular momentum during the collapse.** High Temperature: **Newly formed neutron stars are extremely hot, gradually cooling over time.** Behavior: Pulsars: **When a neutron star\'s magnetic field axis is not aligned with its rotational axis, it emits beams of radiation that appear as pulses when observed from Earth.** Accretion Disks: **In binary systems, a neutron star can pull matter from its companion star, forming a hot accretion disk around it. **Gravitational Lensing: **The extreme gravity of a neutron star can bend light passing near it, allowing for gravitational lensing observations. - **Structure, Physical Properties and Behavior of pulsars** - A pulsar is a rapidly rotating neutron star with an extremely strong magnetic field, emitting beams of electromagnetic radiation from its magnetic poles, which appear as pulses when the beam sweeps across Earth\'s line of sight due to its rotation, making it seem like a cosmic lighthouse; essentially, pulsars are the collapsed core of a massive star left behind after a supernova explosion, composed primarily of neutrons and characterized by incredibly high density and rapid spin rates, producing regular pulses of radiation ranging from milliseconds to seconds depending on the pulsar. **Key structural and physical properties of pulsars: Composition:** Primarily composed of neutrons, with a small percentage of protons and electrons due to the extreme gravitational pressure. **Size:** Radius typically around 10 kilometers, making them incredibly dense. **Mass:** Mass is usually between 1.18 and 1.97 times that of the Sun, with most pulsars around 1.35 solar masses. **Magnetic Field:** Extremely strong magnetic fields, funneling charged particles along the magnetic poles. **Rotation:** Rapid rotation, with periods ranging from milliseconds to seconds, depending on the pulsar. **Behavior of pulsars: Pulse Emission:** As the pulsar rotates, its magnetic field directs beams of radiation (radio waves, X-rays, gamma rays) along the magnetic poles, creating the characteristic pulsed appearance when the beam sweeps across Earth\'s line of sight. **Clock-like Precision:** The regular pulses from a pulsar are remarkably consistent, making them valuable tools for astronomers to study astrophysical phenomena and test theories of gravity. **Slowdown:** Over time, the pulsar\'s rotation gradually slows down due to the energy loss from emitting radiation. **Binary Pulsar Systems:** Some pulsars exist in binary systems with another star, providing valuable insights into gravitational effects and the evolution of stellar systems. **Formation of a Pulsar:** A massive star reaches the end of its life, collapsing under its own gravity during a supernova explosion. The core of the star becomes incredibly dense, forcing protons and electrons to combine and form neutrons, creating a neutron star. If the collapsing core has a strong magnetic field and is spinning rapidly, it can become a pulsar. - **Structure, Physical Properties and Behavior of Black Holes** - A black hole is a region in space where gravity is so intense that nothing, not even light, can escape, and its structure is primarily defined by the \"event horizon\" which marks the point of no return, surrounding a singularity where all the mass is concentrated; the only measurable physical properties of a black hole are its mass, charge, and angular momentum, and its behavior is characterized by the accretion of matter around it, potentially forming jets of high-energy particles as it pulls in material from its surroundings. **Key points about black holes: Structure: Singularity: **The central point of a black hole where all its mass is concentrated, considered to have infinite density and zero volume. Event Horizon: The boundary around the singularity where the escape velocity equals the speed of light, marking the \"point of no return\". **Physical Properties: Mass**: The primary property of a black hole, determining the size of its event horizon.** Charge: **A theoretical property, but generally believed to be negligible as charged particles would be attracted to neutralize the charge. Angular Momentum: A measure of the black hole\'s rotation. **Behavior: Accretion Disk: **As matter falls towards a black hole, it forms a hot, spinning disk around the event horizon. **Gravitational Lensing: **Light from distant objects can be bent around a black hole, allowing for indirect observation. **Jets: **When matter is pulled into a spinning black hole, powerful jets of high-energy particles can be ejected along the rotational axis.** Important Concepts: \"No-Hair Theorem\":** A theoretical concept stating that once formed, a black hole can only be characterized by its mass, charge, and angular momentum. **Schwarzschild Radius:** The radius of the event horizon, proportional to the mass of the black hole. - **Structure, Physical Properties and Behavior of Supernovas** - Structure, Physical Properties, and Behavior of Supernovae. Supernovae are cataclysmic stellar explosions that mark the violent end of a star\'s life. They are classified into various types based on their observed spectra and underlying mechanisms. Let\'s delve into the primary types and their subtypes: **Type I Supernovae: Type Ia Supernovae: Structure:** Typically occur in binary star systems where a white dwarf accretes matter from a companion star. **Physical Properties:** Lack hydrogen in their spectra. Exhibit strong lines of silicon and other heavy elements. Possess a relatively consistent peak luminosity, making them useful as \"standard candles\" for measuring cosmic distances. **Behavior:** Triggered by the white dwarf reaching the Chandrasekhar limit, causing a runaway thermonuclear explosion. Result in the complete destruction of the white dwarf. **Type Ib and Ic Supernovae: Structure:** Originate from massive stars that have lost their outer hydrogen (Ib) or both hydrogen and helium (Ic) layers through stellar winds or mass transfer to a companion star. **Physical Properties:** Lack hydrogen lines (Ib) or both hydrogen and helium lines (Ic) in their spectra. Show strong lines of helium (Ib) or weaker helium lines (Ic). **Behavior:** Result from the core collapse of massive stars. Often leave behind a neutron star or, in some cases, a black hole. **Type II Supernovae: Type II-P Supernovae: Structure:** Occur in massive stars with extended hydrogen envelopes. Physical Properties: Exhibit strong hydrogen lines in their spectra. Show a plateau phase in their light curves, indicating a period of relatively constant luminosity. **Behavior:** Triggered by the core collapse of a massive star. Often leave behind a neutron star. **Type II-L Supernovae: Structure:** Similar to Type II-P supernovae but with less extended hydrogen envelopes. **Physical Properties:** Exhibit strong hydrogen lines in their spectra. Show a linear decline in their light curves, lacking a plateau phase. **Behavior:** Triggered by the core collapse of massive stars. Often leave behind a neutron star. **Other Types: Type IIn Supernovae:** Characterized by narrow emission lines in their spectra, indicating interaction with dense circumstellar material. **Superluminous Supernovae:** Extremely luminous supernovae, often associated with rapidly rotating massive stars. **Key Points:** Supernovae are incredibly energetic events that play a crucial role in the chemical enrichment of the universe. The classification of supernovae is based on their observed spectra and light curves. Understanding the different types of supernovae provides insights into the life cycles of stars and the formation of heavy elements. **Additional Notes:** The study of supernovae is an active field of research, and our understanding of these events continues to evolve. New types of supernovae are being discovered, and the classification system is constantly being refined. Supernovae are important tools for studying cosmology, as they can be used to measure distances and the expansion rate of the universe. - **Orbits, Behavior and Potential evolution of novae** - Orbits, Behavior, and Potential Evolution of Novae. A nova is a cataclysmic stellar explosion that occurs in a binary star system. One star in this system is a white dwarf, a dense remnant of a star that has exhausted its nuclear fuel. The other star is typically a main-sequence star or a red giant. **Orbits and Behavior** Binary Star System: Novae occur in binary star systems where the two stars are in close orbit around each other. **Accretion Disk**: As the white dwarf\'s strong gravity pulls matter from its companion star, an accretion disk forms around the white dwarf. **Thermonuclear Runaway**: The accreted matter builds up on the white dwarf\'s surface until it reaches a critical mass. At this point, a thermonuclear runaway reaction ignites, causing a sudden, dramatic increase in brightness. **Ejection of Matter**: The explosion ejects a shell of gas and dust into space, often at speeds of thousands of kilometers per second. **Light Curve**: The brightness of a nova typically increases rapidly over a few days, reaches a peak, and then gradually declines over weeks or months. **Potential Evolution:** The long-term evolution of a nova system depends on several factors, including the masses of the two stars, the rate of mass transfer, and the frequency of nova eruptions. **Recurrent Novae**: Some nova systems experience multiple eruptions, with intervals ranging from decades to centuries. These are known as recurrent novae. **White Dwarf Growth**: With each eruption, the white dwarf gains mass. If it accumulates enough mass, it may eventually undergo a more catastrophic event, such as a Type Ia supernova. **Merger**: In some cases, the two stars in the binary system may merge, leading to a variety of outcomes, including the formation of a single, more massive star or a black hole. **Observational Significance:** Novae are important objects of study for astronomers because they provide insights into: **Stellar Evolution**: They offer clues about the late stages of stellar evolution and the processes that lead to white dwarf formation. **Nucleosynthesis:** Novae produces and eject a variety of elements, including carbon, nitrogen, and oxygen, which are essential for life. **Distance Measurements**: The brightness of novae can be used to estimate distances to galaxies. By studying novae, astronomers can gain a better understanding of the universe and its history. - **Orbits, Behavior and Potential evolution of dwarf novae** - **Orbits and Behavior: Binary System**: Dwarf novae are composed of a white dwarf and a low-mass companion star, typically a red dwarf. **Close Orbit**: The two stars in a dwarf nova system orbit each other very closely. This proximity allows material from the companion star to be drawn towards the white dwarf due to its strong gravitational pull. **Accretion Disk**: As the material flows from the companion star, it forms a disk around the white dwarf. This accretion disk is heated by friction as the material spirals inward. **Thermal Instability:** Under certain conditions, the accretion disk can become thermally unstable. This instability causes the disk to heat up rapidly, leading to a sudden increase in brightness. **Outbursts**: These outbursts can last for several days or weeks, during which time the system can brighten by several magnitudes. After the outburst, the system returns to its quiescent state, and the process can repeat. **Potential Evolution:** The long-term evolution of dwarf novae is still an area of active research. However, several possible scenarios have been proposed: **Mass Accretion**: As the white dwarf accretes mass from its companion, it can eventually reach the Chandrasekhar limit, the maximum mass a white dwarf can support. At this point, the white dwarf may undergo a thermonuclear explosion, known as a Type Ia supernova. **Common Envelope Evolution**: In some cases, the two stars in a dwarf nova system may merge, forming a single, more massive star. This process is known as common envelope evolution. **Formation of a Cataclysmic Variable**: Dwarf novae are a type of cataclysmic variable star. As the system evolves, it may transition into other types of cataclysmic variables, such as novae or polars. **Additional Considerations:** **Subtypes**: Dwarf novae can be further classified into subtypes based on their outburst characteristics, such as SU Uma stars and Z Cam stars. **Observational Studies**: Observations of dwarf novae, including their light curves and spectra, provide valuable information about the physical processes at work in these systems. **Theoretical Modeling:** Theoretical models of dwarf novae help us understand the underlying physics of these systems and predict their behavior. By studying dwarf novae, astronomers can gain insights into a wide range of astrophysical phenomena, including accretion disk physics, stellar evolution, and the formation of compact objects. - **Orbits, Behavior, and Potential Evolution of X-ray Binaries** - **Orbits**: **Close Binary Systems**: X-ray binaries typically have close orbits, often measured in days or even hours. **Elliptical Orbits:** Many X-ray binaries have elliptical orbits, which can lead to significant variations in the mass transfer rate and X-ray luminosity as the distance between the two stars changes. **Tidal Interactions**: The strong gravitational forces between the two stars can cause tidal interactions, which can affect the orbital period and eccentricity over time. **Behavior**: **Accretion Disk** Formation: As matter flows from the companion star, it forms an accretion disk around the compact object. **X-ray Emission:** The infalling matter in the accretion disk heats up to millions of degrees, emitting intense X-rays. **Jets and Outflows**: Some X-ray binaries produce powerful jets of particles that are ejected at nearly the speed of light. **Variability**: X-ray binaries can exhibit significant variability in their X-ray luminosity, often due to changes in the accretion rate or the geometry of the accretion disk. **Potential Evolution:** **Mass Transfer and Orbital Evolution:** The mass transfer from the companion star to the compact object can cause the binary system to evolve over time. The orbital period can decrease, and the eccentricity can change. **Formation of Millisecond Pulsars:** In some cases, the accretion of matter onto a neutron star can spin it up to extremely high rotation rates, forming a millisecond pulsar. **Type Ia Supernovae**: If the companion star is a white dwarf, the accretion of matter can eventually trigger a thermonuclear explosion, resulting in a Type Ia supernova. **Black Hole Growth**: In systems with black holes, the accretion of matter can lead to the growth of the black hole\'s mass. **Specific Types of X-ray Binaries:** **High-Mass X-ray Binaries (HMXBs):** These systems typically involve a massive companion star, often a supergiant or a Be star. **Low-Mass X-ray Binaries (LMXBs):** These systems involve a low-mass companion star, often a red dwarf or a white dwarf. **Ultra-Luminous X-ray Sources (ULXs):** These are extremely luminous X-ray sources, often associated with intermediate-mass black holes or super-Eddington accretion onto stellar-mass black holes.The study of X-ray binaries provides valuable insights into the physics of accretion disks, the behavior of compact objects, and the evolution of binary star systems. These systems are also important sources of gravitational waves and may play a role in the enrichment of the interstellar medium with heavy elements. - **Orbits, Behavior, and Potential Evolution of Neutron Star Binaries** - **Orbits and Behavior:** **Tight Orbits:** Due to their immense gravitational pull, neutron star binaries have incredibly tight orbits. They can orbit each other multiple times per second! **Gravitational Waves:** As they orbit, they emit gravitational waves, causing their orbits to gradually decay. This energy loss brings the stars closer together over time. **Accretion Disks:** In some cases, one neutron star can accrete matter from its companion, forming a swirling accretion disk. This process can power intense X-ray emission. **Pulsars**: If one or both stars are pulsars, they emit beams of radiation that sweep across the sky like cosmic lighthouses. These pulsars can be used to study the properties of the binary system and test theories of gravity. **Potential Evolution:** The fate of a neutron star binary is a cataclysmic merger. As the stars spiral closer and closer, they eventually collide in a violent event that releases enormous amounts of energy in the form of gravitational waves, electromagnetic radiation, and possibly even a short-lived black hole. **Key Events in a Merger:** **Inspiral:** The stars spiral inward due to gravitational wave emission. **Merger:** The two stars collide, forming a highly distorted object. **Black Hole Formation:** The merged object may collapse into a black hole, accompanied by a powerful burst of gamma rays. **Kilonova:** A brief, intense burst of light is emitted as heavy elements are synthesized and ejected into space. **The Importance of Studying Neutron Star Binaries:** Testing General Relativity: Neutron star binaries provide a unique laboratory to test Einstein\'s theory of general relativity in extreme gravitational regimes. **Understanding the Origin of Heavy Elements:** The merger of neutron star binaries is thought to be a major source of heavy elements like gold and platinum. **Unraveling the Mysteries of the Universe**: Studying these systems can provide insights into the formation and evolution of stars, galaxies, and the universe as a whole. **Observational Techniques: Gravitational Wave Astronomy:** Instruments like LIGO and Virgo detect gravitational waves emitted by merging neutron star binaries. **Electromagnetic Observations:** Telescopes across the electromagnetic spectrum, from radio to gamma rays, observe the light emitted during mergers. By combining these observational techniques, scientists are gaining a deeper understanding of these fascinating cosmic phenomena. - **Orbits, Behavior, and Potential Evolution of Black Hole Binaries** - **Orbits and Behavior: Gravitational Waves:** As the black holes orbit each other, they emit gravitational waves, which are ripples in the fabric of spacetime. These waves carry energy away from the system, causing the orbit to decay over time. **Inspiral:** As the orbit decays, the black holes spiral closer and closer together. This process is known as inspiral. **Merger:** When the black holes are close enough, they merge to form a single, larger black hole. This merger event releases a tremendous amount of energy in the form of gravitational waves. **Ringdown:** After the merger, the newly formed black hole undergoes a period of ringdown, during which any distortions in its shape are dissipated as gravitational waves. **Potential Evolution:** The evolution of a black hole binary depends on several factors, including the masses and spins of the black holes, their initial separation, and the presence of other stars or gas in the system. Here are some possible evolutionary paths: **Isolated Binary:** If the binary is isolated from other stars and gas, it will continue to spiral inward and eventually merge. The time it takes for the merger to occur depends on the initial separation of the black holes. **Dynamical Capture:** In some cases, two black holes may be captured into a binary system through dynamical interactions with other stars in a dense stellar environment, such as the core of a globular cluster. **Stellar Evolution:** Black hole binaries can also form through the evolution of massive binary star systems. If both stars in a binary system are massive enough to collapse into black holes at the end of their lives, they can form a black hole binary. **Observations and Future Research:** The detection of gravitational waves from black hole mergers by LIGO and Virgo has opened a new window on the universe and provided valuable insights into the behavior and evolution of these systems. Future observations with advanced gravitational wave detectors, such as LIGO-India and Einstein Telescope, will allow us to study black hole binaries in even greater detail and to explore a wider range of masses and spins. **Key Points:** Black hole binaries are systems consisting of two black holes in close orbit around each other. They emit gravitational waves as they orbit, causing their orbits to decay. They eventually merge to form a single, larger black hole. Their evolution depends on several factors, including their masses, spins, and initial separation. Gravitational wave observations have provided valuable insights into the behavior and evolution of black hole binaries. - **Observation and classification of post main-sequence stars and stellar remanent by spectra, light curves, and Physical parameters.** - Post-main sequence stars and stellar remnants are classified based on their spectra, primarily by identifying specific absorption lines that reveal the chemical composition and temperature of the star\'s outer layers, allowing astronomers to distinguish between red giants, planetary nebulae, white dwarfs, neutron stars, and black holes, with each type exhibiting unique spectral characteristics depending on their evolutionary stage and mass. **Key points about spectral classification of post-main sequence stars: Spectral class system:** Stars are classified using the Morgan-Keenan (MK) system, with letters O, B, A, F, G, K, and M representing decreasing temperature, where O is the hottest and M the coolest. **Luminosity classes:** In addition to the spectral class, a Roman numeral is added to indicate the star\'s luminosity class (e.g., giant, supergiant, main sequence) based on the width of certain absorption lines in the spectrum. (Temperature, color and luminosity) - Observing and classifying post-main sequence stars and stellar remnants using light curves primarily involves analyzing the characteristic patterns of brightness variations over time, which can reveal key information about the star\'s evolutionary stage, including whether it is a red giant, white dwarf, neutron star, or black hole, based on the presence of pulsations, outbursts, or eclipses in the light curve. Important factors to consider when analyzing light curves: **Periodicity:** The presence of regular or irregular pulsations can indicate specific stellar types. **Amplitude:** The magnitude of brightness variations can reveal the nature of the stellar process causing the variability. **Spectral analysis:** Combining light curve data with spectroscopic observations provides crucial information about the star\'s temperature, composition, and radial velocity. Examples of specific post-main sequence stellar types identified through light curve analysis: **Cepheid variables:** Giant stars with well-defined pulsation periods that are used as standard candles for distance measurements. **RR Lyrae stars:** Similar to Cepheids but with shorter periods, often found in globular clusters. **Cataclysmic variables (CVs):** Binary systems with a white dwarf accreting material from a companion star, causing sudden brightness outbursts. **Symbiotic binaries:** Systems where a hot white dwarf interacts with a cooler, extended envelope, producing unique emission lines in the spectrum. (luminosity, mass) - When observing and classifying post-main sequence stars and stellar remnants based on luminosity, the key factor is their position on the Hertzsprung-Russell (H-R) diagram, where stars with higher luminosity appear towards the top, with different evolutionary stages like red giants, asymptotic giant branch (AGB) stars, and white dwarfs being distinguished by their location relative to the main sequence and their luminosity levels, which are significantly higher than main sequence stars of similar temperature due to their expanded outer envelopes; with the most luminous post-main sequence stars being massive red giants and the least luminous being white dwarfs nearing the end of their evolution **Photometry:** Measuring the star\'s apparent brightness to determine its luminosity. **Spectroscopy:** Analyzing the star\'s spectrum to identify chemical composition and temperature, which helps determine its evolutionary stage. **Parallax measurements:** Determining a star\'s distance to calculate its absolute luminosity - When observing and classifying post-main sequence stars and stellar remnants, the primary factor determining their type is their initial mass, with low-mass stars ending as white dwarfs, intermediate-mass stars potentially forming planetary nebulae around a white dwarf, and high-mass stars exploding as supernovae, leaving behind either a neutron star or a black hole depending on the mass remaining after the explosion. Key points about post-main sequence stars and stellar remnants based on mass: **Low-Mass Stars (\< 8 Solar Masses):** **Evolution:** Become red giants, then shed their outer layers to form a planetary nebula, leaving behind a white dwarf as the stellar remnant. **Intermediate-Mass Stars (8 - 20 Solar Masses):** **Key feature:** May produce more complex and enriched planetary nebulae due to heavier element creation in their cores. **High-Mass Stars (\> 20 Solar Masses):** **Supernova characteristics:** Type II supernovae, often associated with the formation of heavy elements. +-----------------+-----------------+-----------------+-----------------+ | J W S T: | Hubble: | Spitzer: | Chandra | | | | | | | **Orbit** The | **High | **Warm launch** | **Scientific | | JWST orbits the | Precision | The telescope | Principles**: | | Sun at the L2 | Optics:** | was launched at | **X-ray | | point, where | | room | Astronomy**: | | the Sun and | The primary | temperature and | Chandra\'s | | Earth are | mirror, with a | cooled down to | primary purpose | | always in the | large diameter, | its operating | is to observe | | same direction | is meticulously | temperature of | celestial | | relative to the | crafted to | less than 6K | objects in the | | spacecraft. Thi | minimize | after | X-ray spectrum, | | s | optical | launch. **Earth | a region of the | | allows the | aberrations and | -trailing | electromagnetic | | telescope to | maximize light | orbit** The | spectrum | | have a \"cold | collection, | telescope\'s | invisible to | | side\" for | enabling sharp | orbit placed it | the human eye. | | observing and a | images. **Wide | far from Earth, | X-rays are | | \"hot side\" | Wavelength | which allowed | emitted by | | for solar | Range:** Hubble | for passive | high-energy | | panels and | is designed to | cooling and | processes such | | communication | capture light | reduced the | as black holes, | | with | across a broad | amount of | supernova | | Earth. **Optica | spectrum, from | liquid helium | remnants, and | | l | ultraviolet to | needed. **Compa | galaxy | | system** The | near-infrared, | rtmentalization | clusters. By | | JWST\'s optical | allowing | ** | studying these | | system can | observation of | The telescope | emissions, | | correct | various | was divided | astronomers | | wavefront | celestial | into two parts: | gain insights | | errors while in | phenomena. **Ca | the Cryogenic | into the | | orbit. This | ssegrain | Telescope | universe\'s | | means that a | Telescope | Assembly and | most violent | | perfect mirror | Design:** This | the | and energetic | | I \'t required | optical system | Spacecraft. The | phenomena. | | at launch, and | uses a | Cryogenic | **High Angular | | any changes can | combination of | Telescope | Resolution**: | | be | primary and | Assembly | Chandra\'s | | corrected. **Ev | secondary | contained the | design | | ent-driven | mirrors to | cold | prioritizes | | commanding** | focus light | components, | high angular | | The JWST uses | onto the | while the | resolution, | | on-board | instruments, | Spacecraft | allowing it to | | computers and | optimizing | contained the | capture | | software to | image | warm | detailed images | | plan and | quality. **Modu | components. **B | of celestial | | schedule | lar | olt | objects. This | | activities. Thi | Design:** | and go** | is achieved | | s | Hubble is built | Instruments | through its | | allows the | with | were designed | advanced X-ray | | telescope to | replaceable | so that they | optics, which | | skip parts of | instruments, | could be used | consist of | | its observation | allowing for | without | nested pairs of | | plan in | upgrades and | in-orbit | mirrors that | | response to | repairs in | adjustments. | focus X-rays | | real-time | space through | **Eliminate | onto sensitive | | events. **Sunsh | servicing | moving parts** | detectors. | | ield** | missions, | As many moving | **High | | The JWST has a | extending its | parts as | Sensitivity**: | | five-layer | operational | possible were | The | | sunshield that | lifespan. | eliminated. **L | observatory\'s | | blocks heat | **Fine Guidance | ightweight** | detectors are | | sources and | System:** | The telescope | highly | | reduces the | | was designed to | sensitive to | | amount of heat | Precise | be lightweight, | X-rays, | | that reaches | pointing | weighing less | enabling it to | | the telescope | capability | than 110 | detect faint | | by a factor of | ensures the | pounds. **Ritch | sources and | | about 106. This | telescope can | ey-Chrétien | study | | allows the | accurately | design** The | low-luminosity | | telescope to | target specific | telescope was a | objects. This | | cool passively | astronomical | lightweight | sensitivity is | | to around | objects for | reflector with | crucial for | | -390°F. **Infra | observation. ** | an | exploring | | red | Space | 85-centimeter | distant | | focus** The | Environment | diameter | galaxies and | | JWST focuses on | Considerations: | mirror. | understanding | | the near to | ** | | the early | | mid-infrared | The design | b\) Spitzer | universe. | | because it\'s | considers the | Space | **Wide Field of | | easier to | harsh | Telescope | View**: | | observe certain | conditions of | \... | Chandra\'s | | types of | space, | | design | | objects in this | including | | incorporates a | | part of the | temperature | | wide field of | | spectrum. | fluctuations | | view, allowing | | **Testing** The | and radiation, | | it to survey | | JWST underwent | to ensure | | large areas of | | extensive | reliable | | the sky and | | testing over a | operation. | | identify | | six-year | | | potential | | period, |  | | further study. | | cryo-vacuum | | | This capability | | tests and | | | has led to | | separate tests | | | numerous | | for each of its | | | discoveries of | | four scientific | | | new celestial | | instruments. | | | objects and | | | | | phenomena. | |  | | | and Durable | | | | | Construction:** | | | | | Chandra\'s | | | | | components are | | | | | designed to be | | | | | lightweight yet | | | | | extremely | | | | | durable to | | | | | withstand the | | | | | harsh | | | | | environment of | | | | | space. This | | | | | includes | | | | | exposure to | | | | | extreme | | | | | temperatures, | | | | | radiation, and | | | | | micrometeoroids | | | | |. | | | | | **Precision | | | | | Optics**: The | | | | | X-ray mirrors | | | | | are | | | | | manufactured | | | | | with | | | | | extraordinary | | | | | precision, with | | | | | surface | | | | | imperfections | | | | | measured in | | | | | nanometers. | | | | | This level of | | | | | precision is | | | | | essential for | | | | | achieving the | | | | | high angular | | | | | resolution | | | | | required for | | | | | scientific | | | | | observations. | | | | | **Thermal | | | | | Control:** | | | | | Effective | | | | | thermal control | | | | | systems are | | | | | critical for | | | | | maintaining the | | | | | precise | | | | | operating | | | | | temperature of | | | | | Chandra\'s | | | | | instruments. | | | | | This involves a | | | | | combination of | | | | | passive and | | | | | active cooling | | | | | techniques to | | | | | regulate heat | | | | | dissipation. | | | | | **Power | | | | | Generation and | | | | | Management:** | | | | | Chandra\'s | | | | | power system, | | | | | consisting of | | | | | solar panels | | | | | and batteries, | | | | | is designed to | | | | | provide | | | | | reliable and | | | | | efficient power | | | | | supply for the | | | | | observatory\'s | | | | | operations. | | | | | **Data | | | | | Transmission | | | | | and Storage**: | | | | | The | | | | | observatory\'s | | | | | data | | | | | transmission | | | | | and storage | | | | | systems are | | | | | designed to | | | | | efficiently | | | | | capture, | | | | | process, and | | | | | transmit | | | | | scientific data | | | | | back to Earth. | | | | | This involves | | | | | advanced data | | | | | compression and | | | | | error | | | | | correction | | | | | techniques. | | | | | **Autonomous | | | | | Operations:** | | | | | Chandra is | | | | | designed to | | | | | operate | | | | | autonomously | | | | | for extended | | | | | periods, | | | | | requiring | | | | | minimal ground | | | | | intervention. | | | | | This autonomy | | | | | is essential | | | | | for maximizing | | | | | scientific | | | | | productivity | | | | | and minimizing | | | | | operational | | | | | costs. | | | | | | | | | |  | +=================+=================+=================+=================+ | Swift | Fermi | LIGO | Basic Algebraic | | | | | Understanding: | | **SOLID | **Scientific | **Scientific | | | Principles:** | Principles**: | Principles: |  | | Interface | where | based on | | | Segregation, | high-energy | Einstein\'s | L = F x Area = | | and Dependency | gamma-ray | theory of | 4 π | | Inversion) are | photons convert | general | R^2^ σ~SB~ T^4^ | | widely applied | into | relativity, | | | in Swift | electron-positr | which predicts | \"magnitude and | | development to | on | the existence | distance | | create | pairs upon | of | scales\" refer | | well-structured | interaction | gravitational | to a system | | , | with matter. | waves, ripples | used to measure | | flexible, and | **Electromagnet | in the fabric | the distances | | reusable code | ic | of spacetime | to celestial | | components. **T | Radiation**: | caused by | objects based | | ype | Leveraging the | massive cosmic | on their | | Safety:** | properties of | events like | apparent | | Swift\'s strong | electromagnetic | black hole | brightness, | | type system | radiation, | mergers. | often utilizing | | helps prevent | particularly | **Wave-Particle | \"standard | | runtime errors | gamma rays, to | Duality:** LIGO | candles\" like | | by ensuring | study celestial | utilizes | Cepheid | | data types are | objects and | lasers, which | variable stars | | correctly | phenomena. | exhibit both | which have a | | defined and | **Relativistic | wave-like and | predictable | | used throughout | Physics**: | particle-like | relationship | | the code. | Applying | properties, to | between their | | **Immutability: | principles of | detect these | pulsation | | ** | relativity to | tiny | period and | | Favoring | understand the | disturbances in | absolute | | constants (let) | behavior of | spacetime. | luminosity, | | over variables | high-energy | **Interferometr | known as the | | (var) whenever | particles and | y: | \"period-lumino | | possible | their | Interference | sity | | promotes data | interactions. | Patterns**: | relation\" - | | integrity and | **Engineering | LIGO employs an | allowing | | makes code | Principles: | interferometer, | astronomers to | | easier to | Detector | a device that | calculate their | | reason | Technology:** | splits a laser | distance by | | about. **Option | **Scintillation | beam into two | comparing their | | als:** | Detectors:** | and recombines | apparent | | The | Utilizing | them to create | brightness to | | \"Optional\" | scintillation | interference | their known | | type in Swift | detectors to | patterns. Any | intrinsic | | allows | measure the | change in the | brightness. | | developers to | energy and | length of the | | | explicitly | direction of | arms of the | **Magnitude:** | | handle | incident gamma | interferometer, | A | | potential nil | rays. **Silicon | caused by a | measure of how | | values, | Strip | passing | bright an | | preventing | Detectors**: | gravitational | object appears | | unexpected | Employing | wave, alters | from Earth, | | crashes due to | silicon strip | this pattern. | with lower | | missing | detectors for | **Phase | magnitudes | | data. **Protoco | precise | Shifts**: By | representing | | l-Oriented | tracking of | precisely | brighter | | Programming:** | charged | measuring phase | objects. | | Swift heavily | particles | shifts in the | | | utilizes | produced in the | interference | **Apparent | | protocols to | pair production | pattern, LIGO | magnitude:** Th | | define | process. | can detect | e | | contracts and | **Calorimetry** | incredibly | measured | | enable | : | small changes | brightness of a | | polymorphism, | Implementing | in the length | star as seen | | allowing for | calorimetry | of its arms. | from Earth. | | flexible code | techniques to | **Engineering | | | design and | measure the | Principles:** | **Absolute | | greater | energy of | **Precision | magnitude:** Th | | reusability. ** | particles. | Engineering**: | e | | Error | **Spacecraft | **Ultra-High | intrinsic | | Handling:** | Design**: | Vacuum:** LIGO | brightness of a | | Swift\'s | **Robustness**: | operates in an | star, which | | \"do-catch\" | Designing a | ultra-high | would be | | mechanism | spacecraft | vacuum to | observed if it | | provides a | capable of | minimize the | were placed at | | structured way | withstanding | effects of air | a standard | | to handle | the harsh | molecules on | distance of 10 | | potential | conditions of | the laser beams | parsecs. | | errors | space, | and mirrors. | | | gracefully. **V | including | **Vibration | Standard | | alue | extreme | Isolation**: To | candles and the | | Semantics:** By | temperatures, | isolate the | period-luminosi | | default, Swift | radiation, and | interferometer | ty | | structures are | micrometeoroid | from seismic | relation: | | passed by | impacts. | noise, LIGO | | | value, ensuring | **Power | uses | **Standard | | data integrity | Systems**: | sophisticated | candle:** An | | and preventing | Developing | vibration | astronomical | | unintended side | efficient power | isolation | object with a | | effects. | systems to | systems, | known absolute | | **Readability:* | provide the | including | luminosity, | | * | necessary | pendulums and | allowing | | Swift\'s syntax | energy for the | active feedback | astronomers to | | is designed to | spacecraft\'s | systems. | calculate its | | be concise and | operations. | **Laser | distance by | | readable, | **Thermal | Technology**: | measuring its | | emphasizing | Control**: | High-power, | apparent | | clear naming | Implementing | stable lasers | brightness. | | conventions and | thermal control | are crucial for | | | proper | systems to | LIGO\'s | **Cepheid | | indentation. ** | maintain | sensitivity. | variable | | Performance | optimal | Advanced laser | stars:** A type | | Optimization:** | operating | technology | of pulsating | | Swift\'s | temperatures | ensures precise | star where the | | compiler | for the | control of the | period of its | | optimizes code | instruments. | laser beam\'s | pulsation is | | for | **Attitude | properties. | directly | | performance, | Control**: | **Data | related to its | | especially when | Utilizing | Analysis:** | absolute | | working with | precise | **Signal | luminosity. | | large data | attitude | Processing**: | | | sets. | control systems | LIGO generates | **Period-lumino | | | to point the | vast amounts of | sity | | Neil Gehrels | spacecraft | data, which | relation:** The | | Swift | accurately | requires | observed | | Observatory - | towards | sophisticated | correlation | | Wikipedia | celestial | signal | between the | | | targets. **Data | processing | pulsation | | | Handling and | techniques to | period of a | | | Telecommunicati | extract weak | Cepheid | | | ons**: | gravitational | variable and | | | Designing | wave signals | its absolute | | | systems for | from noise. | magnitude, | | | efficient data | **Machine | allowing | | | acquisition, | Learning:** | astronomers to | | | processing, and | Machine | determine the | | | transmission to | learning | distance to a | | | Earth. **Data | algorithms are | Cepheid by | | | Analysis and | used to | measuring its | | | Interpretation: | identify | pulsation | | | ** | patterns in the | period. | | | Statistical | data and | | | | Analysis: | improve the | How to use | | | Applying | accuracy of | standard | | | statistical | signal | candles for | | | methods to | detection. | distance | | | analyze the | | measurements: | | | collected data | What is LIGO? | | | | and draw | \| LIGO Lab \| | **Observe the | | | meaningful | Caltech | apparent | | | conclusions. | | magnitude of | | | **Computational | | the standard | | | Techniques:** | | candle:** Measu | | | Utilizing | | re | | | advanced | | how bright the | | | computational | | star appears | | | techniques to | | from Earth. | | | model and | | | | | simulate | | **Use the | | | astrophysical | | period-luminosi | | | processes. | | ty | | | **Machine | | relation:** Det | | | Learning**: | | ermine | | | Employing | | the absolute | | | machine | | magnitude of | | | learning | | the star based | | | algorithms to | | on its | | | identify | | pulsation | | | patterns and | | period. | | | anomalies in | | | | | the data. | | **Calculate the | | |  | | apparent | | | | | magnitude to | | | | | the known | | | | | absolute | | | | | magnitude, | | | | | calculate the | | | | | distance to the | | | | | star using the | | | | | inverse square | | | | | law of light. | | | | | | | | | | **The absolute | | | | | magnitude of a | | | | | star is simply | | | | | a simple way of | | | | | describing its | | | | | luminosity. | | | | | Luminosity, L, | | | | | is a measure of | | | | | the total | | | | | amount of | | | | | energy radiated | | | | | by a star or | | | | | other celestial | | | | | object per | | | | | second. This is | | | | | therefore the | | | | | power output of | | | | | a star.** | | | | | | | | | | **Comparing | | | | | apparent | | | | | magnitude and | | | | | absolute | | | | | magnitude = m - | | | | | M = 5 log(d/10) | | | | | - 5 **where | | | | | \"m\" is the | | | | | apparent | | | | | magnitude, | | | | | \"M\" is the | | | | | absolute | | | | | magnitude, and | | | | | \"d\" is the | | | | | distance to the | | | | | star in | | | | | parsecs; a | | | | | larger | | | | | difference | | | | | between the | | | | | apparent and | | | | | absolute | | | | | magnitude | | | | | indicates the | | | | | star is further | | | | | away from | | | | | Earth. | | | | | | | | | | ** Inverse | | | | | square law = | | | | | Intensity ∝ 1/ | | | | | (distance)\^2** | | | | | | | | | | **∝ is | | | | | proportional | | | | | symbol** | +-----------------+-----------------+-----------------+-----------------+ - Orbital mechanics, the study of objects moving in orbits around a larger body, is primarily governed by Newton\'s Law of Universal Gravitation, which states that every particle in the universe attracts every other particle with a force directly proportional to the product of their masses and inversely proportional to the square of the distance between them; essentially, this means that the gravitational pull between two objects increases as their masses increase and decreases as the distance between them increases, allowing objects to orbit around one another due to this attractive force. Key points about orbital mechanics and Newton\'s Law of Universal Gravitation: **Force equation:** The gravitational force between two objects, \"m1\" and \"m2\", separated by a distance \"r\" is calculated using the formula: F = G \* (m1 \* m2) / r\^2 where \"G\" is the gravitational constant. **Centripetal force:** For an object to orbit another, the gravitational force acting on it must provide the necessary centripetal force, which is directed towards the center of the orbit, keeping the object moving in a circular path. **Orbital velocity:** The speed required for an object to maintain a stable orbit at a specific distance from the central body is called its orbital velocity. **Kepler\'s Laws:** While derived from Newton\'s Law of Universal Gravitation, Kepler\'s three laws of planetary motion provide a concise description of orbital behavior, including the elliptical nature of orbits, the constant areal velocity, and the relationship between orbital period and semi-major axis. How it works: **Gravitational pull:** When a satellite orbits a planet, the planet\'s gravitational pull constantly pulls the satellite towards its center, preventing it from flying off in a straight line. **Tangential velocity:** To maintain an orbit, the satellite must also have a tangential velocity, a sideways speed that balances the inward gravitational pull, allowing it to \"fall around\" the planet. **Orbital stability:** If the tangential velocity is too low, the satellite will fall closer to the planet; if it\'s too high, it will escape the planet\'s gravitational pull. Applications of orbital mechanics: **Satellite design and deployment:** Understanding orbital mechanics is crucial for designing and launching satellites to achieve desired orbits around Earth or other celestial bodies. **Spacecraft trajectory planning:** Orbital mechanics calculations are used to plan interplanetary missions, including trajectory adjustments and gravitational slingshots. **Astronomy:** Studying the orbits of planets, moons, and stars helps us understand the formation and dynamics of celestial systems. - The Vis-viva equation in orbital mechanics is a fundamental formula that describes the relationship between an orbiting body\'s velocity and its distance from the central body, directly stemming from the principle of conservation of mechanical energy within a gravitational field; essentially, it shows how an object\'s speed changes as it moves closer or further away from the gravitational source, reflecting the interplay between kinetic and potential energy in an orbit. Key points about the Vis-viva equation: **Equation:** v\^2 = GM(2/r - 1/A) **Where:** v is the orbital velocity at a given point G is the gravitational constant M is the mass of the central body r is the distance from the orbiting body to the center of the central body A is the semi-major axis of the orbit **Interpretation:** As r decreases (moving closer to the central body), the velocity v increases due to the stronger gravitational pull, converting potential energy into kinetic energy. Conversely, as r increases (moving further away), the velocity v decreases as kinetic energy is converted back into potential energy. - When considering orbital mechanics and gravitational interaction using the Schwarzschild radius, it essentially means analyzing how objects move around a massive body, like a black hole, where the gravitational pull is so intense that the escape velocity at a certain distance (the Schwarzschild radius) reaches the speed of light, preventing anything, including light, from escaping; this analysis requires using the mathematical framework of general relativity to accurately describe the orbital behavior near such extreme gravitational conditions. Key points about Schwarzschild radius and orbital mechanics: **Definition:** The Schwarzschild radius (Rs) is calculated as R = 2GM/c² where G is the gravitational constant, M is the mass of the object, and c is the speed of light. **Event Horizon:**The Schwarzschild radius marks the boundary of a black hole, known as the event horizon, where the gravitational pull is so strong that nothing can escape. How orbital mechanics changes near a black hole: **Highly Curved Spacetime:** General relativity explains that massive objects like black holes significantly warp spacetime around them, causing objects in orbit to follow curved paths. **Unstable Orbits:** Unlike in Newtonian gravity, orbits close to the Schwarzschild radius become highly unstable, with even small perturbations causing objects to spiral inwards. **Perihelion Precession:** Even for orbits further away from the event horizon, the strong gravity can cause noticeable precession of the perihelion (the point of closest approach) of the orbiting object, an effect observed with Mercury in our solar system.