Rdna Notes 2 PDF
Document Details
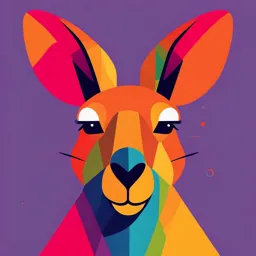
Uploaded by RapidLepidolite2427
Tags
Related
- ANSC20010 Genetics and Biotechnology Section 6 Past Slides PDF
- Module-II-Basic-Biotechnology- PDF
- Biotechnology Techniques - DNA Extraction and DNA Cloning PDF
- PCR Theory and Primer Design - Lecture 12 - DNA Extraction and PCR Theory PDF
- Biotechnology 101 PDF
- DNA Extraction Principles and Techniques PDF
Summary
These lecture notes provide an overview of biotechnology, focusing on key techniques like DNA extraction, PCR, and their applications. The document covers different types of PCR and their uses in various fields, including diagnostics and research.
Full Transcript
LECTURE 12 Biotechnology Overview Biotechnology refers to applied biology using molecular methods and living organisms or their components. It modifies genetic material to create new substances or functions. It is mainly used in medicine, agriculture, and species ident...
LECTURE 12 Biotechnology Overview Biotechnology refers to applied biology using molecular methods and living organisms or their components. It modifies genetic material to create new substances or functions. It is mainly used in medicine, agriculture, and species identification, such as in rats (Araujo et al., 2014) and for antibiotic production. Key Techniques in Biotechnology 1. DNA Extraction o The goal is to purify DNA, separating it from the cell membrane, proteins, and other components. The DNA must be of good quality, quantity, and purity, free of RNA and proteins. o Methods include lysing cells and using chemical/enzymatic treatments to remove unwanted macromolecules. o Three common techniques: ▪ Organic extraction (Phenol-chloroform method) ▪ Non-organic methods (Salting out, proteinase K) ▪ Adsorption method (Silica-gel membrane) o Key chemicals used include lysis buffer (breaks open cells), proteases (break down proteins), RNases (break down RNA), and alcohol (precipitates DNA). 2. Measuring DNA Quality and Quantity o Techniques: ▪ Gel electrophoresis: Separates DNA by size for visualization. ▪ Nanodrop: Measures concentration and purity of DNA using absorbance. ▪ Spectrophotometer: Measures DNA concentration by absorbance at 260 nm. ▪ Qubit: Detects dsDNA quality via fluorometry. ▪ Gel electrophoresis separates DNA by size for purity detection. Nanodrop measures DNA concentration, and spectrophotometry checks absorbance at 260 nm (A260). o The A260/A280 ratio determines DNA quality (DNA absorbs at A260, proteins at A280). 3. Polymerase Chain Reaction (PCR) o PCR is used to amplify specific DNA regions, generating billions of copies in about 2 hours. o Common applications: gene sequencing, paternity tests, disease diagnosis, and forensic identification. o PCR works with small DNA amounts and consists of several components: 1. DNA Template – DNA fragment to be amplified. 2. Dual Primers – Forward and reverse primers that define the start and end of the target region. 3. Taq Polymerase – An enzyme that copies the amplified region. 4. Nucleotides (dNTPs) – DNA building blocks. 5. Buffer – Provides the optimal environment for the polymerase. 4. PCR Process o The PCR process runs on a thermal cycler in stages: ▪ Initial Denaturation – High heat separates DNA strands. ▪ Denaturation – DNA becomes single-stranded at 94–96°C. ▪ Annealing – Primers attach to the DNA at 45–65°C. ▪ Extension – At 72°C, Taq polymerase elongates the new DNA strand. ▪ Final Extension – Ensures complete replication. o The reaction repeats in cycles (e.g., 35 cycles), amplifying DNA exponentially. LECTURE 13-14 1. Multiplex PCR: - Amplifies multiple DNA targets in a single reaction. - Useful for simultaneous analysis of several regions in DNA. 2. Nested PCR: - Reduces non-specific binding. - Involves two PCR reactions: the first amplifies the target DNA, and the product is used in the second reaction. - Used in amplifying small or degraded DNA, such as forensic samples. 3. Inverse PCR: - Identifies unknown sequences using known flanking sequences. - Involves restriction enzyme digestion. - Used for detecting retroviruses and transposons. 4. Reverse Transcriptase PCR (RT-PCR): - Converts mRNA into complementary DNA (cDNA) using reverse transcriptase. - Used to detect or study gene expression. 5. Overlap PCR: - Joins multiple DNA fragments by splicing. - Useful for cloning large DNA fragments or fusing genes. 6. Amplified Fragment Length Polymorphism (AFLP): - Selectively amplifies DNA fragments from digested genomic DNA. - Highly sensitive, detects genetic polymorphisms and is used in microsatellite analysis. Uses of PCR: - Identifying individuals from tiny DNA samples. - Studying ancient DNA from fossils or preserved specimens like mummies. - Amplifying DNA from embryos or for prenatal diagnosis. - Diagnosing diseases like HIV or COVID-19. - Basic genetic research. Gel Electrophoresis (for PCR Analysis): -Purpose: Separates DNA fragments by size using an electric current. -How It Works: DNA (negative charge) moves toward the positive electrode. Smaller fragments move faster than larger ones. *Steps: 1. Prepare gel with agarose powder and buffer. 2. Load DNA samples mixed with dye into wells. 3. Apply voltage; DNA moves based on size. Primer Design: -Primers are short DNA sequences that help start DNA replication. -Key Aspects: Length: 18-30 nucleotides. GC content: 40-60%, affects melting temperature (Tm). Tm Formula: For short primers, Tm = 2(A+T) + 4(G+C). Primers should not bind to each other or themselves. Annealing Temperature (Ta): Calculated by Ta = 5°C below the lowest Tm of primer pair. Primer Types: 1. Universal Primers: Bind to various DNA templates. 2. Species-specific Primers: Amplify DNA from a specific species. 3. Oligo dT Primers: Used in RT-PCR for cDNA synthesis from mRNA. 4. Degenerate Primers: Mix of similar primers for amplifying related genes across different species. LECTURE 16 DNA Sequencing Definition: The process of determining the order of bases in a strand of DNA. Significance: Has revolutionized the understanding of genetics. Applications of DNA Sequencing: Human Disease: Helps identify mutations (e.g., cancer). Genome Studies: Reveals Single Nucleotide Polymorphisms (SNPs). Evolutionary Studies: Provides insight into how genes evolve. Sanger Sequencing Method: Known as the "chain termination method" (first generation sequencing). Developed in 1977 by Frederick Sanger. Used to sequence DNA by creating complementary DNA strands from a single-stranded template. Key Components: DNA Primer: Starts DNA synthesis. dNTPs: Regular nucleotides (A, T, C, G). ddNTPs: Special nucleotides that stop DNA synthesis (lack 3' hydroxyl group). Sanger Sequencing Steps: 1. PCR Amplification: DNA is amplified, then denatured to separate strands. 2. DNA Fragment Generation: DNA fragments of various lengths are generated, each ending with a fluorescently labeled ddNTP. 3. Capillary Electrophoresis: Separates DNA fragments by length (shorter fragments move faster). 4. Laser Detection: A laser detects the fluorescent label on each fragment, generating a chromatogram to display the DNA sequence. Final Outcome: A readable DNA sequence, identified by analyzing the order of fluorescent peaks, with shorter DNA fragments appearing first. LECTURE 17-18 Human Genome Project The Human Genome Project was the first large-scale DNA sequencing project, utilizing Sanger Sequencing (Chain Termination method). Took 13 years to complete (1990-2003) and cost $3 billion. Next Generation Sequencing (NGS) NGS represents the next step in DNA sequencing technology, introduced between 2004 and 2006. Offers massively parallel sequencing with ultra-high throughput, speed, and scalability. NGS revolutionized genomics, allowing sequencing of entire genomes, targeted regions, RNA, and epigenetic studies. Advantages of NGS Increased speed and accuracy while reducing costs and labor. Can sequence more genomes at a lower cost per megabase. Applications of NGS Rapid whole genome sequencing, deep sequencing of target regions, RNA sequencing (RNA-seq), gene expression analysis, and cancer research. Useful for identifying novel pathogens, genetic variants, and epigenetic factors like DNA methylation. Categories of NGS 1. Sequencing by Hybridization: Uses probes to detect specific DNA sequences, commonly for identifying disease-related SNPs and chromosomal abnormalities. 2. Sequencing by Synthesis (SBS): Builds on Sanger sequencing, utilizing cyclic processes to incorporate nucleotides into growing DNA chains. NGS Process Involves sample preparation (library formation through DNA fragmentation and adapter ligation). Amplification occurs on solid surfaces (beads or silicon), creating DNA clusters. Data output consists of millions to billions of short DNA reads, which are analyzed for meaningful insights. Illumina Sequencing (Solexa Technology) Library Preparation: DNA is broken into fragments (200-600 bp), adapters are attached, and the DNA is denatured (made single-stranded). Bridge Amplification: DNA binds to primers on the flow cell, forming bridges that are amplified to create clonal clusters of the target DNA. Sequencing Reaction: Uses fluorescently labeled nucleotides and reversible terminators. After each cycle, the fluorescent signal is read, and the terminator is removed for the next nucleotide incorporation. When to Use NGS vs. Sanger Sequencing Sanger Sequencing: Best for investigating small regions of DNA in a limited number of samples. NGS: More efficient for sequencing multiple samples, detecting variants, and when Sanger sequencing would be too time-consuming or expensive. LECTURE 20 Bioinformatics is an interdisciplinary field that combines computer science, biology, physics, chemistry, and statistics to solve biological problems. Its primary goal is to interpret and analyze large amounts of biological data, helping researchers understand biological processes through computational tools and techniques. Key Aspects of Bioinformatics: Big Data Analysis: Bioinformatics is used to analyze huge biological datasets, such as the human genome, which contains about 20,000 protein-coding genes. Despite this, less than 2% of the human genome encodes proteins, with the remaining 98% containing regulatory elements and noncoding RNA products. Transcriptomes: Bioinformatics identifies the complete set of RNA molecules produced by a genome. Proteomes: Bioinformatics examines the structure and properties of every protein produced by a genome. Centers of Excellence: These centers collect, catalog, and provide access to biological data. They began their work in the early 1980s when DNA sequence data started to accumulate. Examples include: EMBL-European Bioinformatics Institute (EMBL-EBI) US National Center for Biotechnology Information (NCBI) National Institute of Genetics in Japan (NIG) Barcode of Life Data System (BOLD) Biological Databases: Biological databases store and organize data in a structured, searchable, and regularly updated format. These databases cross-reference different types of data for easy retrieval. NCBI and GenBank: NCBI (National Center for Biotechnology Information): Provides access to biomedical and genomic information to advance science and health. GenBank: A genetic sequence database at the NCBI, part of the International Nucleotide Sequence Database Collaboration (with DDBJ and ENA). It contains an annotated collection of publicly available DNA sequences. Data Retrieval: GenBank allows the retrieval of nucleotide sequences, which are foundational to biomedical research and discovery. Data sources include GenBank, RefSeq, TPA, and PDB databases. LECTURE 21-22 BLAST Overview BLAST (Basic Local Alignment Search Tool): Compares biological sequences (DNA, proteins) and retrieves similar sequences from databases. Types of BLAST: 1. Blastp: Protein-protein comparisons. 2. tBlastn: Protein compared with a translated nucleotide sequence. 3. Blastn: Nucleotide-nucleotide comparisons. 4. Blastx: Translated nucleotide compared with a protein sequence. 5. tBlastx: Translates nucleotide sequences and compares them at the amino acid level. BLAST Algorithm Heuristic program: Uses shortcuts for fast searching. Parameters: 1. E-value: Measures the statistical significance of sequence alignment (lower E- value means more significant match). 2. Word size: Length of the seed initiating the alignment. 3. Scoring: Rewards/penalties for matching and mismatching bases, and costs for gaps. 4. Filter and Masking: Masks low-complexity regions to prevent misleading results. BLAST Results The top hit represents the best match to the query sequence. Why BLAST is Popular Flexible search algorithms, reliable statistical reports, rapid search speed, and continual development. Sequence Alignment Essential for comparing sequences by identifying regions of similarity. Algorithms: 1. Global alignment: Aligns entire sequences (best when sequences are the same length). 2. Local alignment: Aligns regions of highest similarity (useful for comparing shorter conserved regions). Alignment types: 1. Pairwise alignment: Compares two sequences, used for genome analysis, protein/functional relationship identification. 2. Multiple sequence alignment (MSA): Aligns 3+ sequences, used to find conserved regions and understand evolutionary relationships. Sequence Homology and Evolution Homology: Shared ancestry between sequences. Orthologs: Genes in different species with a common ancestor, encoding similar proteins. Paralogs: Genes within the same species that arose through duplication and may evolve different functions over time. Importance of Alignments Alignments help in comparing sequences and identifying mutations. Tools like CLUSTAL are used for alignment. Good alignment is essential for accurate analysis, such as studying genetic variations linked to diseases (e.g., cystic fibrosis).