Electron Optics (PHYS 312) Ain Shams University PDF
Document Details
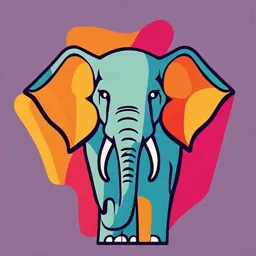
Uploaded by CharitableAffection
Ain Shams University
2024
Ain Shams University
Dr. Rania Badry Amin El Sayed
Tags
Summary
This document is a past paper for the Electron Optics (PHYS 312) course at Ain Shams University. It covers the fundamentals of electron optics and different types of microscopy. This includes light optics and electron microscopy. The document also details sample preparation techniques.
Full Transcript
Physics Department Ain Shams University Faculty of Women for Arts, Science and Education Electron Optics (PHYS 312) Prepared by: Dr. Rania Badry Amin El Sayed Ph...
Physics Department Ain Shams University Faculty of Women for Arts, Science and Education Electron Optics (PHYS 312) Prepared by: Dr. Rania Badry Amin El Sayed Ph.D. Degree in Science (Physics- Spectroscopy) Course title: Electron Optics Code no.: PHYS 312 Number of hours: 2 h lec. /w Course Objectives: - The course introduces the principles and some applications of Electron optics to prepare the student to use her information in different applications. Intended Learning Outcomes of Course (ILOs) The graduates must acquire the following skills: - Knowledge and Understanding: - a1. Identify the principles of Electron optics. a2. Recognize types and design of electron lenses. a3. Describe Motion of electrons in uniform fields. a4. Demonstrate the deferent types of electron microscope and applications. Intellectual Skills: - b1.Choose optimum solutions for physical problems based on analytical thinking. Professional and Practical Skills: - c1. Use effectively the learning resources centers for research tasks. General and Transferable Skills: - d1. Acquire the life-long learning and considering the community-linked problems. Chapter 1 Page 1 of 42 1. Introduction Electron optics is a branch of physics in which the principles of optics are applied to beams of electrons. This branch involves the study and design of instruments used to form, focus, and manipulate electron beams, typically by using electric or magnetic field. Electrons are subject to laws similar to those under light. However, despite this similarity, there are profound differences that lead to greater difficulty in the design of electron optical devices. These differences are as follows: - When it is released from the source of radiation, the beam travels at a speed that is characterized by the speed of light, while the electrons are released from the material by small velocities. In order to obtain electronic packages that are practical for electronic devices, these electrons should be accelerated, and this is achieved by influencing them with suitable electric or magnetic field. - The light beam usually spreads in a straight line and does not deviate from its path except at the surface separating the different media or as a result of changing the density of the medium in which it spreads. The paths of electrons, in general, are curved paths in these fields. 1.1. Classical Optics Classical optics is divided into two main branches: geometrical (or ray) optics and physical (or wave) optics. -Geometrical Optics (ray optics) describes the propagation of light in terms of "rays" which travel in straight lines, and whose paths are governed by the laws of reflection and refraction at interfaces between different media. -Physical Optics, light is considered to propagate as a wave. This model predicts phenomena such as interference , diffraction, and polarization which are not explained by geometric optics. Page 2 of 42 1.2. Types of light lens: Converging (positive) lens: bends rays toward the axis. It has a positive focal length. Forms a real inverted image of an object placed to the left of the first focal point and an erect virtual image of an object placed between the first focal point and the lens (Fig 1). Fig (1) Converging Lens Diverging (negative) lens: bends the light rays away from the axis. It has a negative focal length. An object placed anywhere to the left of a diverging lens results in an erect virtual image (Fig. 2). These two types are used in light microscopes Fig (2) Diverging Lens 1.3. Lens defects: - 1.3.1. Chromatic aberration Chromatic aberration is distortion that occurs when there is a failure of a lens to focus all colors (wavelengths) to the same convergence point. The resulting image would be blurry and delocalized. Page 3 of 42 Fig (3) Chromatic aberration -Correcting the aberration is necessary. In light optics chromatic aberration can be corrected by combining a converging lens with a diverging lens. This is known as a “doublet” lens Fig (4) Correction of chromatic aberration - A few manufacturers have combined an electromagnetic (converging) lens with an electrostatic (diverging) lens to create an achromatic lens -Recent improvements in aberration correction have resulted in significantly-improved image quality and sample information. 1.3.2. Spherical aberration - Spherical aberration occurs when parallel light rays that pass through the central region of the lens focus farther away than the light rays that pass through the edges of the lens. -Result is multiple focal points and a blurred image. Page 4 of 42 -Spherical aberrations are worst at the periphery of a lens so again a small opening aperture that cuts off the most offensive part of the lens is the best way to reduce the effects of spherical aberration. Fig (5) Spherical aberration 1.4. Limitations of the Human Eye Our concept of the physical world comes largely from what we see around us. For most of recorded history, this has meant observation using the human eye, which is sensitive to radiation within the visible region of the electromagnetic spectrum: light with wavelength in the range 300–700 nm. The eyeball contains a fluid whose refractive index (n ≈ 1.34) is substantially different from that of air (n ≈ 1). As a result, most of the refraction and focusing of the incoming light occurs at its curved front surface, the cornea. 1.5. Resolution of the Human Eye Given sufficient light, the unaided human eye can distinguish two points 0.2 mm apart. If the points are closer together, they will appear as a single point. This distance is called the resolving power or resolution of the eye. A lens or an assembly of lenses (a microscope) can be used to magnify this distance and enable the eye to see points even closer together than 0.2 mm. For example, try looking at a newspaper picture, or one in a magazine, through a magnifying glass. You will see that the image is actually made up of dots too small and too close together to be separately resolved by your eye alone. The Page 5 of 42 same phenomenon will be observed on an LCD computer display or flat screen TV when magnified to reveal the individual “pixels” that make up the image. 1.6. Optical microscope (Light microscope) The optical microscope, often referred to as light microscope, is a type of microscope which uses visible light and a system of lenses to magnify images of small samples. Optical microscopes are the oldest design of microscope and were possibly invented in their present compound form in the 17th century. Basic optical microscopes can be very simple, although there are many complex designs which aim to improve resolution and sample contrast. The image from an optical microscope can be captured by normal light-sensitive cameras to generate a micrograph. Originally images were captured by photographic film but modern developments in charge-coupled device (CCD) cameras allow the capture of digital images. Purely digital microscopes are now available which use a CCD camera to examine a sample, showing the resulting image directly on a computer screen without the need for eyepieces. Alternatives to optical microscopy which do not use visible light include scanning electron microscopy and transmission electron microscopy. There are two basic configurations of the conventional optical microscope: the simple microscope and the compound microscope. The vast majority of modern research microscopes are compound microscopes while some cheaper commercial digital microscopes are simple single lens microscopes. A magnifying glass is, in essence, a single lens simple microscope. In general, microscope optics are static; to focus at different focal depths the lens to sample distance is adjusted, and to get a wider or narrower field of view a different magnification objective lens must be used. Most modern research microscopes also have a separate set of optics for illuminating the sample. Page 6 of 42 Fig (6) Optical (light) microscope 1.7. Applications of optical microscope Optical microscopy is used extensively in microelectronics, nanophysics, biotechnology, pharmaceutics’ research, mineralogy and microbiology. Also, it is used for medical diagnosis, the field being termed histopathology when dealing with tissues, or in smear tests on free cells or tissue fragments. 1.8. Types of optical microscope Additionally, there are two types of optical microscope which are simple optical microscope and compound optical microscope Point of comparison (P.O.C) Simple Compound Materials Glass Glass Ray or beam Light Light Structure Simple Complex Number of lenses Single lens Large number of lenses Resolution Low High Page 7 of 42 Chapter 2 Page 8 of 42 2.1. Electron The electron is an elementary particle, with a negative electric charge. The electron has a mass that is approximately 1/1836 that of the proton. Electrons have properties of both particles and waves; they can collide with other particles and can be diffracted like light. The wave properties of electrons are easier to observe with experiments than those of other particles like neutrons and protons because electrons have a lower mass and hence a larger De Broglie wavelength for a given energy. Since an electron has charge, it has a surrounding electric field, and an electron moving relative to an observer generates a magnetic field. External electromagnetic fields affect an electron according to the Lorentz force law. Electrons radiate or absorb energy in the form of photons when accelerated. Laboratory instruments are capable of containing individual electrons as well as electron plasma using electromagnetic fields. Special telescopes can detect electron plasma in outer space. With respect to electron optics, the nature of the electron as a charged particle causes electrons to interact with imposed electric fields, and their spin causes magnetic field interactions as well. These interactions form the fundamentals of electron optical theory. Thus, Electrons play an essential role in numerous physical phenomena, such as electricity, magnetism, and thermal conductivity, and they also participate in gravitational, electromagnetic and weak interactions. 2.2. Motion of electrons in uniform (electrostatic and magnetic) fields 2.2.1. Effect of uniform magnetic field on the motion of the electron The force F acting on an electron in a uniform magnetic field is given by F Bev Where B is the strength of magnetic field, e charge of electron and v the electron velocity. Page 9 of 42 Since the magnetic force F is at right angles to the velocity direction, the electron moves round a circular path. Centripetal force = mv2/r The centripetal acceleration of the electrons is Bev a m v 2 Bev a Hence r m which gives mv r eB e/m = v/Br If the electron enters the field at an angle to the field direction the resulting path of the electron (or indeed any charged particle) will be helical as shown in Figure (7). Fig. (7) Motion of electron under the effect of magnetic field 2.2.2. Effect of uniform electric field on the motion of the electron Consider an electron beam directed between two oppositely charged parallel plates as shown in Fig. (4). With a constant potential difference between the two plates, the trace is curved towards the positive plate. Page 10 of 42 Fig. (8) Motion of electron under the effect of electric field The force acting on each electron in the field is given by eVP F eE d where E = electric field strength, V p= potential difference between plates, d = plate spacing. The vertical displacement y is given by With the acknowledgement of the acceleration of the electron in the y direction we can determine the equation for the path of the electron 1 1 eVp 2 y at 2 ( )t 2 2 md 1 eVp x 2 ( ) 2 md v 2 This is the equation for a parabola. It must be remembered that the electric force acts along the line of the electric field direction while the magnetic force acts at right angles to the field direction. Note that: Charged particles move in parabolas if projected into an electric field in a direction at right angles to the field. Meanwhile, charged particles move in straight lines and accelerate (or decelerate) if projected into an electric field along the direction of the field. Page 11 of 42 Examples: 1- An electron is accelerated from rest through a potential difference of 5000 V and then enters a magnetic field of strength 0.02 T acting at right angles to its path. Calculate the radius of the resulting electron orbit. 2- In an electron microscope, (i) what accelerating voltage is needed to produce electrons with wavelength 0.0600nm? (ii) If protons are used instead of electrons, what accelerating voltage is needed to produce protons with wavelength 0.0600nm? (hint: me=9.1x10−31kg and mp=1.67x10−27kg) 3- An electron after being accelerated through a potential difference 100 V enters a uniform magnetic field of 0.004 T, perpendicular to its direction of motion. Calculate the radius of the path described by the electrons. (hint: 6.63 × 10−34 J·s. and me=9.1x10−31kg ) 2.3. Types and design of electron lenses: The idea of working the electron lenses, if the beam travels from a high-voltage area to a low-voltage area or vice versa, it deviates. That is, the electric field as well as the magnetic field work on the deviation of electrons and thus can be converged or diverged. There are two types of electron lens: the electrostatic lens and magnetic lens sometimes called electromagnetic lens. 2.3.1. Electrostatic lenses: - The most straightforward example of an electric field is the uniform field produced between two parallel conducting plates. An electron entering such a field would experience a constant force, regardless of its trajectory (ray path). This arrangement is suitable for deflecting an electron beam. The simplest electrostatic lens consists of one plate containing a circular hole (aperture) centered about the optic axis. The plate D is placed between two parallel electrodes A and C and voltage between them V2 (assuming that the voltage of C is zero); if the voltage of plate D is different, the shape of the voltage level distribution between A and C change. Instead of being flat, parallel and the spread of the electrons falling on it is straight, the voltage level is bent and the electron beam is either diverged or converged. Page 12 of 42 Fig. (9) Electrostatic Lens Electrostatic lenses have been used in cathode-ray tubes. Although some early electron microscopes used electrostatic lenses, modern electron-beam instruments use electromagnetic lenses that do not require high-voltage insulation and have somewhat lower aberrations. Example of electrostatic lenses: The cathode ray tube is the optimal application of electrostatic lenses. The cathode- ray tube (CRT) is a vacuum tube that contains one or more electron guns and a phosphorescent screen, and is used to display images. It modulates, accelerates, and deflects electron beam(s) onto the screen to create the images. A cathode-ray tube is a display device used in television sets and computer monitors. It is not possible to construct a negative magnetic lens although negative electrostatic lenses can be made Fig. (10) Cathode Ray Tube Page 13 of 42 2.3.2. Magnetic Lens: - Instead of an electrostatic field we can also use a magnetic field to focus charged particles. The Lorentz force acting on the electron is perpendicular to both the direction of motion, and to the direction of the magnetic field. A homogeneous field deflects charged particles, but does not focus them. The simplest magnetic lens is a donut-shaped coil through which the beam passes, preferably along the axis of the coil. To generate the magnetic field an electrical current is passed through the coil. The magnetic field is strongest in the plane of the coil, and gets weaker as we move away from it. In the plane of the coil, the field gets stronger as we move away from the axis. Thus, a charged particle further from the axis experiences a stronger Lorentz force than a particle closer to the axis (assuming that they have the same velocity). This gives rise to the focusing action. Unlike the paths in an electrostatic lens, the paths in a magnetic lens contain a spiralling component, i.e. the charged particles spiral around the optical axis. The vast majority of electron microscopes in use today use magnetic lenses due to their superior imaging properties, and the absence of the high voltages that are required for electrostatic lenses. Fig. (11) Magnetic Lens Page 14 of 42 2.4. Physical similarity and difference between light and electron lenses: 2.4.1. Similarity: Two types are diverging or converging 2.4.2. Difference: Point of comparison Light lenses Electron lenses (P.O.C) Materials Glass Metal Ray or beam Light Electron Types Diverging and Electrostatic and converging lenses magnetic lenses Application Used in light Used in electron microscopes microscopes 2.5. Types of Microscopies There are two types of microscopy: 1- Optical (light) microscope. 2- Electron microscope [Transmission Electron Microscopy (TEM) and Scanning electron microscope (SEM)]. 2.5.1. Electron Microscope What is the Electron Microscopy? The electron microscope is a type of microscope that uses a beam of electrons to create an image of the specimen. It is capable of much higher magnifications and has a greater resolving power than a light microscope, allowing it to see much smaller objects in finer detail. They are large, expensive pieces of equipment, generally standing alone in a small, specially designed room and requiring trained personnel to operate them. Page 15 of 42 The faster the electrons travel, the shorter their wavelength. The resolving power of a microscope is directly related to the wavelength of the irradiation used to form an image. Reducing wavelength increases resolution. Therefore, the resolution of the microscope is increased if the accelerating voltage of the electron beam is increased. The accelerating voltage of the beam is quoted in kilovolts (kV). It is now possible to purchase a 1,000kV electron microscope, though this is not commonly found. 2.5.2. Principle of electron microscopy Electrons are such small particles that, like photons in light, they act as waves. A beam of electrons passes through the specimen, then through a series of lenses that magnify the image. The image results from a scattering of electrons by atoms in the specimen. Differences between Light Microscope and Electron Microscope Light Microscope Electron Microscope Illuminating sourceis the Light. Illuminating source is the beam of electrons. Specimen preparation takes usually few minutesto hours. Specimen preparation takes usually takes few days. Live or Dead specimen may beseen. Only Dead or Dried specimens are seen. Condenser, Objective and eyepiece lenses are made up of glasses. All lenses are electromagnetic. It has low resolvingpower (0.25µm to It has high resolving power (0.001µm), about 250 0.3µm). times higher than lightmicroscope. It has a magnification of500X to 1500X. It has a magnification of 100,000X to 300,000X. Page 16 of 42 The object is 5µmor thicker. The object is 0.1µm or thinner. Image is Colored. Image is Black and White. Vacuum is notrequired. Vacuum is essential for its operation. There is no need ofhigh voltage electricity. High voltage electric current is required (50,000 Volts and above). There is no coolingsystem. It has a cooling system to take out heat generated by high electric current. Filament is notused. Tungsten filament is used to produce electrons. Radiation risk isabsent. There is risk of radiation leakage. Specimen is stainedby colored dyes. Specimen is coated with heavy metals in order to reflect electrons. Image is seen by eyes through ocular Image is received in Zinc Sulphate Fluorescent lens. Screen or PhotographicPlate. It is used for thestudy of detailedgross internal structure. It is used in the study of external surface, ultra structure of cell and verysmall organisms. Page 17 of 42 Chapter 3 Page 18 of 42 3.1. Types of Electron Microscopes: - Transmission Electron Microscope (TEM) Scanning Electron Microscope (SEM) 3.1.1. Transmission Electron Microscope (TEM): In the 1920s, it was discovered that accelerated electrons behave in vacuum much like light. They travel in straight lines and have wavelike properties, with a wavelength that is about 100,000 times shorter than that of visible light. Furthermore, it was found that electric and magnetic fields could be used to shape the paths followed by electrons similar to the way glass lenses are used to bend and focus visible light. Ernst Ruska at the University of Berlin combined these characteristics and built the first transmission electron microscope (TEM) in 1931. For this and subsequent work on the subject, he was awarded the Nobel Prize for Physics in 1986. The first electron microscope used two magnetic lenses, and three years later he added a third lens and demonstrated a resolution of 100 nm, twice as good as that of the light microscope. Today, electron microscopes have reached resolutions of better than 0.05 nm, more than 4000 times better than a typical light microscope and 4,000,000 times better than the unaided eye. There are four main components to a transmission electron microscope: An electron optical column, a vacuum system, the necessary electronics (lens supplies for focusing and deflecting the beam and the high voltage generator for the electron source), and control software. A modern TEM typically comprises an operating console surmounted by a vertical column and containing the vacuum system, and control panels conveniently placed for the operator. The microscope may be fully enclosed to reduce interference from environmental sources. It may even be operated remotely, removing the operator from the instrument environment to the benefit of both the operator and the instrument. The electron column includes elements analogous to those of a light microscope. The light source of the light microscope is replaced by an electron gun, which is built into the column. The glass lenses are replaced by electromagnetic lenses. Unlike glass lenses, the power (focal Page 19 of 42 length) of magnetic lenses can be changed by changing the current through the lens coil. (In the light microscope, variation in magnification is obtained by changing the lens or by mechanically moving the lens). The eyepiece or ocular is replaced by a fluorescent screen and/or a digital camera. The electron beam emerges from the electron gun (usually at the top of the column), and is condensed into a nearly parallel beam at the specimen by the condenser lenses. The specimen must be thin enough to transmit the electrons, typically 0.5 μm or less. Higher energy electrons (i.e., higher accelerating voltages) can penetrate thicker samples. After passing through the specimen, transmitted electrons are collected and focused by the objective lens and a magnified real image of the specimen is projected by the projection lens onto the viewing device at the bottom of the column. The entire electron path from gun to camera must be under vacuum (otherwise the electrons would collide with air molecules and be scattered or absorbed). Fig. (8) Illustration of a TEM 3.1.2. Electron Source Three main types of electron sources are used in electron microscopes: tungsten, lanthanum hexaboride (LaB6 ) , and field emission gun (FEG). Each represents a different combination of costs and benefits. The choice of source type is an important part of the instrument selection process. Perhaps the single most important characteristic of the Page 20 of 42 source is brightness, which characterizes the electron current density of the beam and the angle into which the current is emitted (current density per steradian solid angle); and ultimately determines the resolution, contrast and signal-to-noise capabilities of the imaging system. FEG sources offer brightness up to 1000 times greater than tungsten emitters, but they are also much more expensive. In some high current applications, LaB6 or tungsten may actually work better than FEG. A tungsten gun comprises a filament, a Wehnelt cylinder, and an anode. These three together form a triode gun, which is a very stable source of electrons. The tungsten filament is hairpin-shaped and heated to about 2700°C. By applying a high positive potential difference between the filament and the anode, thermally excited electrons are extracted from the electron cloud near the filament and accelerated towards the anode. The anode has a hole in it so that an electron beam, in which the electrons may travel faster than two thousand kilometers per second, emerges and is directed down the column. The Wehnelt cylinder, which is held at a variable potential slightly negative to the filament, directs the electrons through a narrow cross-over to improve the current density and brightness of the beam. Tungsten sources are least expensive, but offer lower brightness and have limited lifetimes. The brightness of a tungsten source can be increased, but only at the cost of shorter lifetime. Because the emission area is large, a tungsten source can provide very high total beam current. Like tungsten, LaB6 guns depend on thermionic emission of electrons from a heated source, a lanthanum hexaboride crystal. LaB6 sources can provide up to 10x more brightness than tungsten and have significantly longer lifetimes, but require higher vacuum levels, which increases the microscope’s cost. The emitting area of LaB6 is smaller than tungsten, increasing brightness but reducing total beam current capability. Field emission guns, in which the electrons are extracted from a very sharply pointed tungsten tip by an extremely high electric field, are the most expensive type of source, but generally provide the highest imaging and analytical performance. High resolution TEM, based on phase contrast, requires the high spatial coherence of a field emission source. The higher brightness and greater Page 21 of 42 current density provided by these sources produce smaller beams with higher currents for better spatial resolution and faster, more precise X-ray analysis. Field emission sources come in two types, cold field emission and Schottky (thermally assisted) field emission. Cold field emission offers very high brightness but varying beam currents. It also requires frequent flashing to clean contaminants from the tip. Schottky field emission offers high brightness and high, stable current with no flashing. The latest generation of Schottky field emitters (FEI XFEG) retains its current stability while attaining brightness levels close to cold field emission. As a rule of thumb, if the application demands imaging at magnifications up to 40- 50 kX in TEM mode, a tungsten source is typically not only adequate, but the best source for the application. When the TEM imaging magnification is between 50-100 kX, then the brightest image on the screen will be generated using a LaB6 source. If magnifications higher than 100 kX are required, a field emission source gives the better signal. In the case of small probe experiments such as analytical or scanning techniques, then a field emission gun is always preferred. Fig. (11) Thermionic Electron Gun Page 22 of 42 P.O.C. Tungsten LAB6 Field emission Construction 1- A filament Organic compounds and 1- Heating power 2- A wehnelt lanthanum and boride supply cylinder 2- Emitter 3- An anode 3- Extraction, 4- Accelerating voltage 5- Anode Shape Hairpin-shape Lanthanum hexaboride Different shapes crystal Brightness Lower brightness More brightness than - Schottky field tungsten emission: high brightness and high, stable current with no flashing - Cold field emission: very high brightness but varying beam current and require frequent flashing to clean contaminants from the tip. Image magnification 40-50kX 50-100kX Higher than 100kX Cost Low cost Require higher vacuum The most expensive type levels, which increases of source the microscope’s cost Lifetime Shorter lifetime Longer lifetime Longest lifetime Page 23 of 42 Is a microscopic technique in which a beam of electrons is transmitted through a specimen to form an image. An image is formed from the interaction of the electrons with the sample as the beam is transmitted through the specimen. The image is then magnified and focused onto an imaging device, such as a fluorescent screen or a layer of photographic film. Electron source Evacuated chamber Sample port Viewing screen Fig. (9) TEM device Page 24 of 42 3.1.4. How does TEM Work 1. Tungsten filament: generate a beam of electrons that is then focused on the specimen by the condenser. 2. Condensed lenses: are used to focus the beam. 3- Vacuum system: The column containing the lenses and specimen must be under high vacuum to obtain a clear image because electrons are deflected by collision by air molecules. 4. Objective lenses: form enlarged, visible image of the specimen on a fluorescent screen. 5. Photographic film: The screen can also be moved aside and the image captured on photographic film as permanent record. Fig. (10) TEM device Page 25 of 42 Conducting Non-conducting 1. Samples must be 2- Must be coated with a 1. Samples must be conductive material to prevent thinned thinned charging 3.1.6. Electron Sample Interaction The following types of species are created once the electron beam hits the sample: Backscattered electrons Secondary electrons Auger electrons X‐Rays Un-scattered electrons (Transmitted) Elastically scattered electrons Inelastically scattered electrons Page 26 of 42 Incident electron beam Backscattered electrons-Elastic Inelastic- Secondary electrons Characteristic X rays Auger Electrons 2θ Inelastic scattering Elastic scattering-Diffraction Transmitted beam Fig. (11) Electron interaction Page 27 of 42 3.1.7. Advantages of TEM 1. TEM has a resolution of better than 1 nm 2. It gives structural information as crystal structure, symmetry and orientation of materials. 3. It can give morphological information of shape and size of particles in a microstructure. 4. It can also reveal the nature of crystallographic defects. 5. It is capable of obtaining composition analysis at nanoscale. 6. It gives us two dimensional image. 3.1.8. Disadvantages of TEM 1. Low sampling volume and rather slow process of obtaining information 2. High capital and running cost of materials 3. Special training required for the operation of the equipment. 4. Difficult sample preparation. 5. Magnetic samples require special care. 6. Non-conducting samples require gold or carbon coating. 3.2. Scanning Electron Microscopy (SEM): The SEM is a microscope that uses electrons instead of light to form an image. The scanning electron microscope has many advantages over traditional microscopes. The SEM has a large depth of field, which allows more of a specimen to be in focus at one time. The SEM also has much higher resolution, so closely spaced specimens can be magnified at much higher levels. Because the SEM uses electromagnets rather than lenses, the researcher has much more control in the degree of magnification. All of these advantages make the scanning electron microscope one of the most useful instruments in research today. In the SEM, we use much lower accelerating voltages to prevent beam penetration into the sample since what we require is generation of the secondary electrons from the true surface Page 28 of 42 structure of a sample. Therefore, it is common to use low KV, in the range 1-5kV for biological samples, even though our SEMs are capable of up to 30 kV. 3.2.1. How does a SEM work? A beam of electrons is produced at the top of the microscope by an electron gun. Electron gun fitted with a tungsten filament cathode. Tungsten is normally used in thermionic electron guns because it has the highest melting point and lowest vapor pressure of all metals, thereby allowing it to be heated for electron emission, and because of its low cost. The electron beam is focused by one or two condenser lenses to a spot about 0.4 nm to 5 nm in diameter. The beam passes through pairs of scanning coils or pairs of deflector plates in the electron column, typically in the final lens, which deflect the beam in the x and y axes so that it scans in a raster fashion over a rectangular area of the sample surface. When the primary electron beam interacts with the sample, the electrons lose energy by repeated random scattering and absorption within a teardrop-shaped volume of the specimen known as the interaction volume, which extends from less than 100 nm to around 5 µm into the surface. The size of the interaction volume depends on the electron's landing energy, the atomic number of the specimen and the specimen's density. Once the beam hits the sample, electrons and X-rays are ejected from the sample. Detectors collect these X-rays, backscattered electrons, and secondary electrons and convert them into a signal that is sent to a screen similar to a television screen. This produces the final image. Page 29 of 42 Fig. (12) SEM device Page 30 of 42 3.2.2. Advantages of SEM 1. Work very fast 2. Provides high-resolution images of a sample’s surfaces. 3. SEM can produce 3D images of the sample by tilting it at different angles and capturing multiple images. 4. Provide great depth of focus. 5. When SEM is used in conjunction with energy dispersive spectroscopy (EDS), the analysis also provides qualitative and quantitative chemical analysis data about the sample. 6. SEMs are also easy to operate with the proper training and advances in computer technology and associated software make operation user-friendly. 7. SEM can provide valuable information about surface roughness, texture, and topography, which is important in many applications, such as quality control and surface engineering. 3.2.2. Disadvantages of SEM 1. SEMs are expensive, large and must be housed in an area free of any possible electric, magnetic or vibration interference. 2. Special training is required to operate an SEM as well as prepare samples 3. SEMs are limited to solid and inorganic samples 4. SEMs carry a small risk of radiation exposure associated with the electrons that scatter from beneath the sample surface. 5. Only surface features can be seen Page 31 of 42 3.3. SEM and TEM images of some Nanoparticles SEM TEM Nanorods Nanofibers Page 32 of 42 Core Shell NPs CoV-19 virus particles Transmission electron micrograph of SARS-CoV-2 virus particles Page 33 of 42 Colorized scanning electron micrograph of an apoptotic Colorized scanning electron micrograph of an cell (red) heavily infected with SARS-COV-2 virus apoptotic cell (blue) infected with SARS-COV-2 particles (yellow), isolated from a patient sample. Image virus particles (yellow) captured at the NIAID Integrated Research Facility (IRF) in Fort Detrick, Maryland. Credit: NIAID. Colorized scanning electron micrograph of an apoptotic cell (green) heavily infected with SARS-COV-2 virus particles (purple), Page 34 of 42 SEM - ZnO TEM ZnO TEM - Ag Page 35 of 42 TEM Agglomerated CuO NPs Page 36 of 42 TEM Core/shell NPs Page 37 of 42 3.4. Comparison between TEM and SEM: P.O.C TEM SEM Electron Beam Beam focused to fine point; sample Broad, static beams is scanned line by line Voltages Needed Accelerating voltage much lower; TEM voltage ranges from not necessary to penetrate the 60-300,000 volts specimen Interaction of the beam electrons Wide range of specimens allowed; Specimen must be very thin simplifies sample preparation Imaging Electrons must pass through Information needed is collected near and be transmitted by the the surface of the specimen specimen Image Rendering Transmitted electrons are collectively focused by the Beam is scanned along the surface of objective lens and the sample to build up the image magnified to create a real image 3.5. Sample preparation in electron microscopes. Step 1: primary fixation with aldehydes (proteins) As for the TEM sample prep, proteins are fixed and the ultrastructure stabilised as a result of crosslinking by formaldehyde and/or glutaraldehyde. Step 2: secondary fixation with osmium tetroxide (lipids) In addition to fixing bilipid membranes osmium tetroxide increases sample conductivity and minimizes image distortions resulting from charging. Step 3: dehydration series with solvent (ethanol or acetone) Page 38 of 42 A fixed specimen is dehydrated by incubation in a series of ethanol or acetone solutions. Solvent concentration is increased gradually so that water is removed gently, without causing artefacts, mainly shrinkage. Step 4: drying Allowing acetone or ethanol to simply evaporate from sample surface would create artefacts as these solvents have relatively high surface tension and would create micro- ripping of the surface upon leaving. Step 5: mounting on a stub The specimen is mounted on a metal stub using a sticky carbon disc which increases conductivity. Silver-containing glue can additionally be applied for even more conductivity. Step 6: sputter coating with conductive material To prevent charge build up on specimen surface, it is coated with a conductive material, most commonly gold. The metal is applied in a controlled manner in a sputter coater. It is critical that the coating is thick enough to prevent charging (typically around 10 nm) but not thick enough to obscure specimen surface details. 3.6. Applications of Electron Microscopy: - 1. Biology and life sciences Diagnostic electron microscopy Cryobiology Protein localization Electron tomography Cryo-electron microscopy Toxicology Page 39 of 42 Biological production and viral load monitoring Particle analysis Pharmaceutical QC Structural biology 3D tissue imaging 2. Materials research Materials qualification Medical research Nano prototyping Nanometrology Device testing and characterization 3. Industry High-resolution imaging 2D & 3D micro-characterization Macro sample to nanometer metrology Particle detection and characterization Sample preparation Chemical/Petrochemical Page 40 of 42