GEOL3051A (2024) Quiz 1 PDF
Document Details
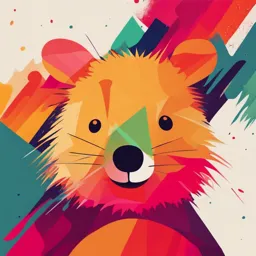
Uploaded by FriendlyTrust
Wits
2024
Linda Iaccheri
Tags
Summary
This document is an introduction to GEOL3051A (2024), a course on mining geology taught at Wits University. It covers topics on the formation of ore deposits, their classification, and the socio-economic drivers for mining activities. The document details the course outline, assessments, and expectations for students.
Full Transcript
GEOL3051A (2024): L1 Introduction Dr Linda Iaccheri Lecturer, School of Geosciences [email protected] Linda Iaccheri Room 7b, Ground Floor School of Geosciences Lecturer in Mining Geology and Wits Isotope Geoscience Laboratory (WIGL) [email protected]...
GEOL3051A (2024): L1 Introduction Dr Linda Iaccheri Lecturer, School of Geosciences [email protected] Linda Iaccheri Room 7b, Ground Floor School of Geosciences Lecturer in Mining Geology and Wits Isotope Geoscience Laboratory (WIGL) [email protected] MSc Geology – Italy PhD Geology/Geochemistry - Australia Industry experience Research interests: Economic Geology, from waste to $ and ore-forming hydrothermal processes GEOL3051A 2024 Block 3: July 15th to August 30th Block 4: September 9th to October 18th Lectures Mondays and Fridays : in GLT Lectures Thursdays : GLT? Practicals in GLT CONSULTATIONS: every Thursday after the class (from 9.45 to 11.00am) I encourage you to ask questions during the lectures and practicals! By the end of this course, students are expected to have: Detailed knowledge of Southern African’s major mineral fields and resources, their genesis and suitability for exploitation. An understanding of the socio-economic drivers for mining and exploration activities. An understanding of the roles of a mining engineer in the mining and exploration industries. Knowledge of the mining value chain The course runs for 2 blocks: Block 3: ➔Understand how ore deposits form and where they are located Block 4: ➔Resources classification and resources orebody modelling GEOL3051A - BLOCK 3 Ore deposits definitions Classification of ore deposits (magmatic, hydrothermal, sedimentary) Ore-forming processes (how do they form, where they are located and why?) Ore deposits in South Africa GEOL3051A - BLOCK 4 Exploration geology and techniques (how do we find ore deposits?) SAMREC Code Modifying factors Resources vs Reserves classification How to build and understand a resource orebody modelling FINAL EXAM: A 2-hour examination will be held in November. The final year mark for GEOL3051A consists of: November examination mark: 50% Class assessments mark: 50% (Block 3 + Block 4 + attendance to practicals) GEOL3051A - BLOCK 3 Assessments There are a total of 4 assessments in Block 3, which will contribute to your class mark: Monday 29th July (12.30-13.15): Quiz 1 on Lectures weeks 1 and 2 (10%) Monday 12th August (12.30-13.15): Quiz 2 on Lectures weeks 3 and 4 (10%) Monday 26th Aug (12.30-13.15): Quiz 3 on Lectures week 5 and 6 (10%) Tues 13 Aug and Tues 20 Aug: Group presentations on a specific ore deposit/commodity (10 minutes) (20%) GEOL3051A (2024) - BLOCK 3 From me: Attendance is mandatory! 10% class mark on attendance! Quiz and exams will be on what we discuss in the classroom Feel free to interrupt me during the lecture Discussions are highly valued and encouraged I expect that you stay on top of your learning, engage in the classroom and do not wait until the end of the term for bulk learning Why economic geology should be important to you? When do you use resources from ore deposits? Since when humankind is using natural resources? Mining - then LION CAVE (ESWATINI) IRON ORE: OLDEST MINE Source: Bert Woodhouse African adventure ~43 000 years ago, cosmetics were being mined to beautify men and woman - who even than recognised that nature needs a little help. Which commodity(s) are mined at the moment in South Africa? Mining – now in South Africa, for example… Sishen iron ore mine, northern Cape: 14 km long Platinum Mines, source Anglo American Rustenburg Mining - now South Deep gold mine, Mamatwan manganese Mpumalanga; mine, Northern Cape 7th deepest Mining supports our daily life Remember – if we don’t fish for it or grow it, we MINE it! Mining supports our daily life Although we don’t think much of the resources that we use daily, our lives are reliant on Earth's natural resources. Example: computer: microprocessor made of silicon, drivers made of various metals and metal alloys, all of which are encased in plastic, which is made from oil, or maybe your computer has an aluminum case, which is also from the earth. Before they powered your computer, the components of these parts once started as raw materials within the earth. Global implications: 4 Global implications: ► What will happen to demand of resources? ► Which resources will become critical in 4 the near future? Global implications: More people means that we need more natural resources. As the population grows, we are facing many important questions. ► How long will our oil reserves last? ► Are there new ore bodies that we haven't discovered yet? ► Can we find new mineral resources deeper in the earth? To help us answer these questions, we turn to the field of economic geology. You as a mining engineer: Why do you need to know about geology and how the ore deposits form? Have you ever seen this diagram? Have you ever seen this diagram? The Mine Value Chain Mine Value Chain Extend the life of a mine! 1. Professional Membership Free for students! SGA and SEG Student Chapters 8 2.Driving licence 3.Passport Free for students! GEOL3051A L2 what is an ore deposit? Dr Linda Iaccheri School of Geosciences [email protected] “why economic geology should be important to you?” What is Economic Geology? What is an ore deposit? What is Economic Geology? Economic geology is the study of the formation and extraction of earth materials that have some economic potential in society. Economic potential means that they are materials that are currently valuable or may potentially be valuable in the future. The economically valuable materials are generally called mineral resources and include minerals and ore deposits. What is an ore deposit? Ore deposits = what is mined or has been mined Useful rocks that are mined for a profit ($) Which commodities are included in the ore deposits? Which commodities are included in the ore deposits? - Ores of metals - Ores of gemstones - Ores of minerals used in industrial products - Rocks used as aggregate, for building stone - Coal and oil-shale Oil and gas are also commonly referred to as mineral resources, despite the fact that they are not actually minerals. Ore deposits are useful rocks that are mined for a profit How do I know that a rock is useful?? Example of ore minerals (see Practical 1) Rocks are composed of minerals; The important ore minerals include: Native metals Sulphides Oxides and hydroxides Silicate Carbonates Many of the ore minerals are rare in the Earth crust Ridley 2013 99% of the minerals that form the Earth’s crust are made of just 8 elements, called MAJOR ELEMENTS: The rest of the elements of the periodic table are called TRACE ELEMENTS. Ore minerals are rare in the Earth’s crust! 99% of Earth’s crust is made of just 8 elements: Geological processes active on the Earth concentrate ore minerals (and trace elements) in certain places to form ore deposits - Geochemical classification of the elements based on their predominant bonding affiliation - Subdivision of the elements (major and trace) of the periodic table into 4 groups - It was originally formulated to explain the chemical differentiation of the Earth into the core, mantle, crust, and atmosphere Ridley 2013 Lithophile Elements MOST OF THE MAJOR ELEMENTS ARE BELONGS TO THIS GROUP It includes the major elments Chalcophile Elements Chalcophile elements combine readily with sulphur, and have low affinity with oxygen, forming Sulphides (examples below and Practical 1) MOST OF THE SCARCE METALS ARE BELONGS TO THIS GROUP Copper chalcopyrite CuFeS2 covellite CuS chalcocite Cu2S Zinc sphalerite ZnS Lead galena PbS Nickel pentlandite (Ni,Fe)9S8 Molybdenum molybdenite MoS2 Mercury cinnabar HgS Siderophile Elements Native Elements Importance of the GOLDSCHMIDT CLASSIFICATION: Elements can be divided into: 1. Lithophile (oxygen affinity, dominantly silicates and oxides, abundant in the crust) 2. Chalcophile (sulphides affinity) 3. Siderophile (iron affinity, rare in the crust) 4. Atmophile (atmosphere affinity) Knowing the affinity of an element helps in predicting in which mineral group the ore metals will dominantly occur The Goldschmidt classification helps us in predicting in which mineral we will find economically important metals that we want to mine Example: Cu is a chalcophile element Cu ore minerals are sulfides Most of the ore metals are in low concentrations in the Earth’s crust The table shows the average percentage concentrations of ore metals in the crust in ppm: To form an ore deposit, the percentage of valuable metal must be greatly enriched above its average percentage in the Earth’s crust Ridley 2013 Concentration Factors The Concentration Factors Concentration Factor is the amount by which the abundance of an element must be increased above its normal/average concentration in the crust to make an ore deposit (to make it commercially extractable). Average crustal abundance of an element in % and ppm: Al and Fe (= Major elements) present in % Trace elements in ppm, because they are scarce in the Earth’s crust Minimum exploitable concentration of an element to make profit: how many times the concentration must be increased to have an exploitable abundance of a metal Concentrator Factor This concentration/enrichment factor increases with decreasing abundance of the element in the crust. How can the concentration of an element in the crust be increased so to reach minimum profitable value? How can the concentration of an element in the crust be increased so to reach minimum profitable value? 1. Chemical processing… (sometimes) 2. GEOLOGICAL PROCESSES! We need natural (GEOLOGICAL) processes that concentrates the elements above their average abundance in the crust to form an ore deposits In order to find the location of the ore deposits, we must understand the relationship between minerals – rocks – geological processes: The rock cycle The rock cycle Different geological processes will contribute to the formation of different types of ore deposits. There are main 3 types of ore deposits, shaped by 3 main geological processes: 1. Igneous - ores formed by igneous processes in and around magma chambers; 2. Surface/Sedimentary processes – ores are deposited at the earth’s surface or subsurface 3. Metamorphic or Hydrothermal- ores deposited from hot fluid circulation in the crust Classifications of ore deposits There are many thousands of known ore deposits simplifies the process of developing an understanding the ore deposit; allows estimation of statistical measures of ore deposit spatial distribution, ore grade and ore tonnages The classification of ore deposits into types or classes is based on combinations of their characteristics. Classifications of ore deposits Different classification schemes of ore deposits are based on different features as the primary attribute for categorization: element or mineral extracted, e.g. Cu, Au, Fe etc; host-rock type, e.g. large ultramafic–mafic intrusive bodies, carbonate-bearing sedimentary rocks; tectonic setting and/or geological age, e.g. back-arc basin, intracratonic sedimentary basin, Proterozoic intracratonic basins; major genetic process of enrichment, e.g. magmatic processes, sedimentary processes. Three main types of ore deposits related to the 3 main geological processes: (a) Classification of the principal rock types (b) and an analogous, but much simplified, classification of ore deposit types 6 GEOL3051A L3 Ore terminology Dr Linda Iaccheri School of Geosciences [email protected] Simple ores: Complex ores (polymetallic): By-products: Gangue mineral effects Gangue Waste (overburden) Waste (tailings) Grades and concentration factors Grade Cut-off grade Concentration factor Ores and by-products Simple ores: yield a single metal from a mineral e.g. galena yields Pb Complex ores: one or more metals dominate production with a number of minor metals, known as by-products e.g. the Platreef By-products may determine whether a particular metal or deposit is worth mining Ore minerals have to be separated from uneconomic gangue minerals Gangue is the commercially worthless material that surrounds or is closely mixed with, a wanted mineral in an ore deposit. It must be separated/processed from the ore minerals so to extract the commodity. It can be: A mineral (example, calcite, quartz, pyrite, olivine) harmful elements may occur in both ore and gangue - tennantite (Cu12As4S13) in Cu ore can introduce unwanted arsenic and sometimes Hg - P or U in Fe concentrates - As in Ni concentrates or orogenic gold deposits Waste or Overburden is rock or materials overlying or surrounding the orebody that are displaced during mining without being processed. Tailings = rock/material already stripped of valuable minerals. Grade – the concentration of an element in an orebody Cut-off grade – is the lowest grade at which a rock can be mined for a certain element – (this depends on ease of extraction, location, metal price etc. It is an economic definition). Concentration factor – the concentration needed from the abundance in the average crust to reach the cut-off grade How do you calculate the grade of a deposit? 11 12 Hypogene and Supergene ores Syngenetic and Epigenetic ores Hypogene and Supergene ores Hypogene – mineralisation caused by ascending hydrothermal fluids Supergene – mineralisation caused by descending fluids, generally associated with weathering Syngenetic and Epigenetic ores Syngenetic ores were formed at the same time, and by a similar processes to the host rocks e.g. an iron-rich sedimentary horizon Epigenetic ores were formed at a later time e.g. a mineralised vein Massive ore Dispersed (disseminated) ore Confined ore Concordant ore Discordant ore Dispersed Confined Example: Coal deposits Example: porphyry copper deposits GEOL3051A L4: Let’s talk about igneous rocks! Dr Linda Iaccheri School of Geosciences [email protected] The formation of ore deposits is related to the main geological processes and the formation of rocks Three main types of ore deposits related to the main geological processes: 1. Igneous or magmatic ores form by igneous processes in and around magma chambers; 2. Metamorphic or Hydrothermal ores are related to hot fluid circulation in the crust; 3. Sedimentary ores form at the earth’s surface or subsurface by sedimentary processes. 6 Igneous or magmatic ore are associated and related to igneous processes… We must revise - What are Igneous/magmatic rocks? - What are magmatic processes? INTRUSIVE Igneous (=magmatic) rocks: Extrusive = volcanics Intrusive How do you classify them? How do you distinguish one from another? Felsic minerals: Mafic minerals: Felsic minerals: Mafic minerals: Olivine Quartz Pyroxene Feldspars: Amphibole Orthoclase, Albite, Biotite (mica) Anorthite Muscovite (mica) Felsic rocks Mafic rocks Pyroxene Amphibole Pyroxene Amphibole Bowen’s Reaction Series? Pyroxene Amphibole GEOL3051A L5 Orthomagmatic ore deposits Dr Linda Iaccheri School of Geosciences [email protected] Magmatic ore deposits also known as orthomagmatic ore deposits, ore minerals crystallised from a melt or were transported by a melt. Mineralisations as a result of chemical and mineralogical processes in the magmas Where do we have magmatism on Earth’s crust? Where do magmas form? Where do we have magmatism on Plate tectonics on Earth’s surface Earth’s crust? Where do magmas form? Magmas originate in: Subduction of the crust; Rifts/spreading centers; Hot Spots; Kimberlite eruptions within a continent. Different types of magmatism on Earth’s crust: What is meant by partial melting? What is causing the partial melting? The partial melting in the Earth’s crust is due to an increase of T. The required T for partial melting depend on: 1. Pressure (decompression) 2. Volatile presence 1. Partial melting due to variation in pressure Most substances are more dense in crystalline solid form than in liquid state increase in Pressure favors a more compact, solid arrangement of atoms If this pressure drops (e.g. because of fracturing) at high temperatures the solid may begin to melt Pressure drop = decompression On the Earth’s crust, where do we may have pressure drop = decompression and partial melting? Example: Decompression melting of the mantle at divergent plate margins (during rifting) 2. Partial melting due to presence of Volatiles Natural magmas contain dissolved Volatile such as water & gases (O2, CO2, H2S, HCL, CH4, CO); General effect of volatiles is to lower melting temperatures of silicate minerals MORE VOLATILES = LOWER PARTIAL MELTING TEMPERATURES On the Earth’s crust, where do we have presence of volatiles that can facilitate partial melting? Example in subduction zones, where the partial melting of the mantle and crust is due to introduction at depth of fluids/volatiles due to a down going subduction slab. GEOL3051A L5 Introduction to magmatic ore deposits Dr Linda Iaccheri School of Geosciences [email protected] Magmatic ore deposits also known as orthomagmatic ore deposits, ore minerals crystallised from a melt or were transported by a melt. Mineralisations as a result of chemical and mineralogical processes in the magmas Where do we have magmatism on Earth’s crust? Where do magmas form? Where do we have magmatism on Plate tectonics on Earth’s surface Earth’s crust? Where do magmas form? Magmas originate in: Subduction of the crust; Rifts/spreading centers; Hot Spots; Kimberlite eruptions within a continent. Different types of magmatism on Earth’s crust: What is meant by partial melting? What is causing the partial melting? The partial melting in the Earth’s crust is due to an increase of T. The required T for partial melting depend on: 1. Pressure (decompression) 2. Volatile presence 1. Partial melting due to variation in pressure Most substances are more dense in crystalline solid form than in liquid state increase in Pressure favors a more compact, solid arrangement of atoms If this pressure drops (e.g. because of fracturing) at high temperatures the solid may begin to melt Pressure drop = decompression On the Earth’s crust, where do we may have pressure drop = decompression and partial melting? Example: Decompression melting of the mantle at divergent plate margins (during rifting) 2. Partial melting due to presence of Volatiles Natural magmas contain dissolved Volatile such as water & gases (O2, CO2, H2S, HCL, CH4, CO); General effect of volatiles is to lower melting temperatures of silicate minerals MORE VOLATILES = LOWER PARTIAL MELTING TEMPERATURES On the Earth’s crust, where do we have presence of volatiles that can facilitate partial melting? Example in subduction zones, where the partial melting of the mantle and crust is due to introduction at depth of fluids/volatiles due to a down going subduction slab. Crystallization of Magmas Once a melt (magma) is produced, the magma tends to move/flow away from where it was produced flows upwards into rocks of lower temperature and pressure → cools = CRYSTALLIZATION; Rate of cooling determines in part the type of rock formed, as different minerals begin to crystallize at different temperatures or times BOWEN’S REACTION SERIES Modifying Melt Composition Magma compositions may be modified after the melt has formed as a result of: 1. Fractional crystallization 2. Assimilation 3. Magma mixing (1) Fractional Crystallization Primary way that melts composition change Early formed crystals physically removed from remaining melt by crystal settling; prevented from reacting with melt (1) Fractional Crystallization Early-formed crystals settle out and become isolated from melt by later crystals settling above them Remaining melt may even move away in zones of weakness in surrounding rocks, leaving early crystals behind. (2) Assimilation A magma composition can also be changed if a magma assimilates the country rock around it, incorporating fragments (xenoliths), melting & mixing these fragments e.g. ice-cubes into hot coffee: ice melts & coffee cools as it becomes diluted The temperature of the magma must be high enough to melt the country rock. (3) Magma Mixing Occurs when 2 magmas combine to produce a hybrid melt intermediate in composition between them Another scenario: separation of a magma into two or more immiscible melts due to oversaturation of one element The chemical composition of a melt depends on the composition of the magma sources partial melting of the mantle mafic magmatism basalts (mafic rocks) partial melting of the crust felsic magmatism granites (felsic rocks) Magmas due to melting of the mantle Magmas due to melting of the crust partial melting of the mantle mafic magmatism basalts (mafic rocks) partial melting of the crust felsic magmatism granites (felsic rocks) Mafic magmatism will form different ore deposits compared to felsic magmatism Which ore deposits are associated with mafic magmatism and which ones are associated with felsic magmatism? Which ore deposits are associated with mafic magmatism and which ones are associated with felsic magmatism? It depends on the Goldschmidt elements classification Magma types and metal contents Relative abundances of selected metals in basalt, andesite, and rhyolite… Example: Chalcophile elements Relative abundances of selected metals in basalt, andesite, and rhyolite… Example: Lithophile elements Felsic and mafic magmatic rocks have different (typical) metal associations: Mafic magmatic rocks/ Ni, Cr, Cu, Co, Felsic magmatic Oceanic/mafic Pt, Pd and Au Li, Sn, Mo, U, C, rocks/ crust REE and W continental crust Implications for understanding ore genesis processes Mineral exploration! Goldsmith elements classification Main types of ore deposits associated with mafic oceanic crust : Robb 2005 Main types of ore deposits associated with the continental crust: Robb 2005 Most of the world’s known ore deposits are hosted in rocks of the continental crust Lithophile elements ores are related to granites and alkaline intrusions Compatible and Incompatible elements Compatible and Incompatible elements A compatible element is one that is readily incorporated into a rock forming mineral; And it prefers to be in the solid/mineral than in the liquid/melt Examples: Ca, V, Cr, Ni.. An incompatible element, in contrast, is one which does not readily form a rock mineral and ‘prefers’ residing in the melt; It is usually too large and/or too highly charged to easily form common rock-forming minerals; Examples; Rb, Sr, Ba, K, Zr, REE, Li, U, Mo The concentration of the compatible elements is greater in the mineral than in the melt, while the concentration of incompatible elements is lower in the mineral. Partial melting and fractional crystallisation Partial melting and fractional crystallisation Partial melting: melting of different minerals at different temperatures Fractional crystallization: crystallization of different minerals at different temperatures and selective separation of elements in different mineral phases from the original melt 5 Processes of ore formation in magmatic systems: 1. Concentration of ore elements as a result of very low degrees of partial melting; 2. Accumulation and concentration of ore minerals in magma chambers during progressive crystallization and fractionation of magmas; 3. Separation of two immiscible melts in a magma chamber; 4. Extreme fractionation during progressive crystallisation of a magma; 5. Incorporation of a mineral that occurs at a specific depth in the Earth. 5 Processes of ore formation in magmatic systems: (1) Concentration of ore elements as a result of very low degrees of partial melting Formation of a melt rich in incompatible elements; Example: carbonatites 5 Processes of ore formation in magmatic systems: (2) Accumulation and concentration of ore minerals in magma chambers during progressive crystallization and fractionation of magmas; Crystallisation of mineral phases with compatible elements Accumulation of these crystals at the bottom of the magma chamber Example: stratiform chromitites reefs 5 Processes of ore formation in magmatic systems: (3) Separation of two immiscible melts in a magma chamber Formation and segregation of a sulfide-rich melt from a silicate melt; Example: Ni-Cu-PGE deposits 5 Processes of ore formation in magmatic systems: (4) Extreme fractionation during progressive crystallisation of a magma The leftover melt after advanced fractional crystallization is rich in incompatible elements and volatiles; Example: REE deposits and pegmatites 5 Processes of ore formation in magmatic systems: (5) incorporation of a mineral that occurs at a specific depth in the Earth. The melt transport to the surface a mineral collected at depth Example: diamonds in kimberlites Deposits formed during differentiation of a magmas: chromite deposits Bushveld – chromitites Podiform chromitites Deposits formed from separation of immiscible sulfide melt phases: base- metal Ni–Cu-PGE sulfide deposits in mafic and ultramafic rocks Nickel sulfide ores in komatiites Sudbury Norilsk Bushveld - Merensky Reef Deposits formed through incorporation of a mineral from depth in the Earth into magma: diamond deposits in kimberlites Tomorrow Practical 2 Please bring to the practical: Pencil & eraser Ruler Calculator Protractor Coloured pencils GEOL3051A L6 Orthomagmatic ore deposits: chromitites Dr Linda Iaccheri School of Geosciences [email protected] 5 Processes of ore formation in magmatic systems: 1. Concentration of ore elements as a result of very low degrees of partial melting; 2. Accumulation and concentration of ore minerals in magma chambers during progressive crystallization and fractionation of magmas; 3. Separation of two immiscible melts in a magma chamber; 4. Extreme fractionation during progressive crystallisation of a magma; 5. Incorporation of a mineral that occurs at a specific depth in the Earth. Today we will explore the following processes in mafic/ultramafic systems: Accumulation and concentration of ore minerals in magma chambers during progressive crystallization and fractionation (differentiation) of mafic magmas: chromite deposits Separation of two immiscible melts in a magma chamber: base-metal Ni–Cu-PGE sulfide deposits Orthomagmatic ultramafic and mafic Intrusions mafic/ultramafic melts are related to the partial melting of the mantle and magmatic processes in the mantle They represent basaltic magmas that crystallised at depth in large magma chambers (intrusions) These intrusions show the effects of slow cooling and strong fractionation They are natural laboratories to study magmatic processes and preserve the crystalline products of fractionation Repositories of some of the largest ore bodies on earth Cu, Ni, PGE, Cr, V. Orthomagmatic ultramafic and mafic Intrusions: What is the difference between an ultramafic and a mafic rock? Which mineral(s) do you expect to have in a ultramafic rock? Which mineral(s) do you expect to have in a ultramafic rock? Example of ultramafic rock? Example of a mafic rock? Pyroxene Amphibole Distribution of layered intrusions worldwide Examples of Orthomagmatic ultramafic and mafic Intrusions Name/Complex Age Location Area km2 Bushveld Palaeoproterozoic South Africa 90,000 Dufek Jurassic Antarctica 50,000 Duluth Precambrian Minnesota, USA 4,700 Stillwater Precambrian Montana, USA 4,400 Muskox Precambrian NW Territory, Canada 3,500 Great Dyke Palaeoproterozoic Zimbabwe 3,300 Kiglapait Precambrian Labrador, Canada 560 Skaergåard Eocene East Greenland 100 Largest ore bodies on Earth for Cu, Ni, PGE, Cr, V Mafic and ultramafic rocks are hosting the biggest resources of Cu, Ni, PGE, Cr, V We saw that metals have different behaviour in geological processes (example: Goldschmidt classification; compatible/incompatible) These metals are not all concentrated in the same reef or rock type Firstly, we will explore the formation of Cr deposits: What is a chromitite? The Chromitite is Massive orebody of magmatic chromite minerals Chromium and Chromite Cr is compatible in Spinel Group minerals and clinopyroxene Chromite is a spinel-group mineral, shows quite a lot of chemical variation as it typically contains Mg and Al as well as other trace elements eg Ti FeCr2O4 – MgCr2O4 (solid solution) Up to 55% Cr Density (ρ) of 4.4-5.1 g/cm3 Mohs hardness (5.5) Chromitites ores bodies > 30% Cr2O3 Magmatic chromite mineralisations occur almost exclusively in association with mafic and ultramafic igneous rocks (anorthosites, dunites, peridotites and pyroxenites). Chromitites layers and pods within Chromitites layers dunite and within anorthosites pyroxenites There are two different geological settings where we can find chromite ore deposits: 1. Podiform chromitites associated with ophiolites 2. Stratiform chromitites associated with layered mafic/ultramafic intrusions 1. Podiform chromites associated with ophiolites What is an ophiolite? 1. Podiform chromites associated with ophiolites ophiolite A fragment of oceanic crust and upper mantle that have been tectonically emplaced onto continental crust in continental collisional tectonic settings. 16 Preservation of Ophiolites Ophiolites are the product of collision of a continental margin or island arc and closure of an ocean Location of Podiform chromite deposits Ophiolites represent the preserved sections of oceanic lithosphere thrusted onto a continental margin during continental collision: Example: Troodos Ophiolitic Complex, Cyprus Idealised complete ophiolitic succession (section of oceanic lithosphere) Sheeted dykes Moho sediments Pillow basalt (lava) dykes Massive diorite and gabbro Layered gabbro Dunite and chromite Peridotite/piroxenite Tectonised ultramafic rocks (part of the upper mantle) In the idealised complete ophiolitic succession Podiform Chromites are : associated with ultramafic rocks, within peridotites, dunites and tectonised ultramafic rocks Pipe-like to pod-like shapes Mostly small Often in clusters Podiform chromites associated with ophiolites Olivine chromitites, Troodos Ophiolite in the shape of pods, ranging from pea-size nodules to large bodies hundreds of meters in extent that may take the form of tabular, cylindrical, or highly irregular bodies Massive pods of chromite ore may be accompanied. by a lateral zone of disseminated chromite that can be traced for hundreds of meters 2. Stratiform chromitites associated with layered mafic/ultramafic intrusions Two Rivers UG2 Mine Chromite Anorthosite UG1 2. Stratiform chromitites associated with layered mafic/ultramafic intrusions → Most are in the Bushveld Complex! Great Dyke (Zimbabwe) Stillwater Complex (Montana, USA) Kemi intrusion (Finland) Dwars River, Eastern Bushveld 2. Stratiform chromitites associated with layered mafic/ultramafic intrusions What is a layered mafic/ultramafic intrusion? A layered intrusion is a fossilized mafic magma chamber They preserve a record of crystal separation and accumulation from mafic/ultramafic magmas Magmatic layering O’Driscoll & VanTongeren (2017) - Elements Magmatic layering Ol Ol Opx Ol Pl Pl Chromitite seam in the Rum intrusion Merensky Reef in the Bushveld Complex Modal layering in the Main Zone Magnetitites in the Upper Zone Magmatic layering in a mafic/ultramafic layered intrusion is interpreted to reflect new magma inputs into the magma chamber Oikocrystic layering in the Critical Zone What do we know about the Bushveld Complex, also known as the Rustenburg Layered Suite? The Bushveld Complex is a Large Igneous Province largest layered igneous complex area of 95,000 km2 intruded between 2060 and 2054 Ma into an Archean basement world’s largest reserves of Cr and PGE’s The Bushveld Complex Several lobes or limbs: – Western – Eastern – Northern – far western – Bethal limb lobes are connected saucer-shaped and sill-like The ultramafic/mafic rocks succession form the 7 km Rustenburg layered Suite, which is overlaid by about 3 km granite suite (Rashoop/Lebowa suites) The Rustenburg layered Suite… Platreef 3 km granitic Granites rocks RUSTENBERG LAYERED SUITE 2000 m 7 km 4000 m layered mafic rocks 400 - 1000 m 800 m 0 – 1600 m The Rustenburg layered Suite: rock types and location of mineralisations: RUSTENBERG LAYERED SUITE V-rich magnetite layers ferrogabbros Platreef norites and gabbro-norites no current metal interest, but presence of dimension stone quarries Anorthosite, norite, chromitite layers and Merensky Reef Orthopyroxenites, chromitite layers Earliest cumulates with olivine and opx-rich packages (dunite and pyroxenites) MERENSKY REEF MERENSKY REEF chromitite anorthosite Chromitites in the Bushveld Complex occur in 3 UG2 REEF stratigraphic groups within the Critical Zone: Upper Group (UG1, UG2, UG3) Middle Group (MG1, MG2, MG3, MG4) Lower Group (LG1, LG2, LG3, LG4, LG5, LG6, LG7) Lower group (LG1-7) chromitites Chromitites layers alternate pyroxenites layers Middle group chromitites Upper group chromitites (UG1-3) UG1 at Dwars River, Eastern Bushveld The UG2 can be traced for at least 350 km around the Complex UG2 Reef chromitite layer mined for PGE UG2 with 5-8 g/t PGE UG2 – typically ~1 m thick Peg Px= pegmatoidal pyroxenite Px= pyroxenite Example of simplified geological succession UG1 and UG 2 (Western Limb – Bushveld Complex) How do chromitite layers form? Simplified ore genetic model: Partial melting of the mantle to create mafic/ultramafic melts Chromium is derived from the partial melting of the mantle Chromium is part of the mafic/ultramafic melt which then ascends through the crust into a mafic/ultramafic magma chamber The mafic/ultramafic magma then crystallizes the mineral chromite (FeCr2O4). Accumulation of chromite into layers either in situ or by gravity-settling The chromitite puzzle - A mass balance problem: Chromite is usually an accessory mineral (not a major component) in a mafic/ultramafic silicate rock how to form mono-mineralic chromitite layers made-up of ~80% chromite crystals? The chromitite puzzle - A mass balance problem Cr is usually 0.1 wt% of a basaltic magma To form a chromitite layer with 40-50% Cr2O3 a large amount of basaltic magma is required At Union Mine, the total thickness of all of the chromitites is 25 m This equates to 20-30 km of magma. There is TOO MUCH chromite in the Bushveld Complex relative to the amount of mafic rocks that are preserved The amount of mafic melt required to form chromitites layers would be preserved a 13 km thick Critical Zone, instead it is only 1.5 km thick So where is the magma that was required to precipitate all the chromitites layers that we find in the Bushveld? Discussed Models for the formation of chromitite layers in the Bushveld Complex (very debated!) Mechanisms suggested for the crystallization of chromite to form mono- mineralic chromitite layers: 1. Changes in pressure in the magma chamber 2. Country rock assimilation from the mafic/ultramafic melt 3. Magma mixing 1. Changes in pressure in the mafic/ultramafic magma chamber Increasing P can remove olivine from the crystallization sequence therefore chromite will crystallize until the pressure drops and olivine will crystallize again. How do we change P across the entire magma chamber? This may be tectonically-driven Alternative is to increase the amount of dissolved CO2 in the magma so there is overpressure 2. Country rock assimilation Chromitite layers could be precipitated when the basaltic parental magma of the intrusion is “suddenly extensively contaminated” with granitic liquid due to the partial melting of the siliceous roof rocks. This is represented by quartz in the triangular phase diagram to the top right: A basaltic magma at “A” would become contaminated by SiO2 and the resulting mix of magma and contaminant would fall somewhere along the dashed line “E-H” and crystallize only chromite. Mixtures of a melt of composition (A) with SiO2 lead to oversaturation in chromite (E-H, see plot to the right) Irvine (1975) – Geochimica acta Cosmochimica 3. Magma mixing Chromite saturation can be caused by mixing between a residual basaltic magma (composition D) and a more primitive basaltic magma (composition E, in the plot to the right) Mixing of the two magmas produces a composition which is within the chromite phase (F) and therefore chromite crystallises D and forms a chromitite layer. F E In the Bushveld Complex… Chromitites correspond to “replenishment horizons” in the mafic-ultramafic layered sequence Successive sill-like injections of new ultramafic melts into a resident viscoplastic mafic/ultramafic magma chamber Chromitite petrogenesis is a confusing and controversial matter Multiple different models have been proposed Most viable are changes in pressure, contamination, and magma mixing in the magma chamber More work to be done before chromitite petrogenesis is solved Why is chromite important? 2022 → What is chromium used for? It is a silver-white, hard metal, resistant to tarnish Its main use is in stainless steels and to form alloys Refractory chromite bricks are used to line furnaces Chemical compounds for the manufacture of Paints, inks, preservatives Tanning industry A catalyst Essential in the Green Economy GEOL3051A L7 Orthomagmatic base-metal Ni–Cu sulfide deposits Dr Linda Iaccheri School of Geosciences [email protected] Processes of ore formation in magmatic systems 1. Accumulation and concentration of ore minerals in magma chambers during progressive crystallization and fractionation (differentiation) of mafic magmas: chromitite deposits 2. Ore deposits formed by the separation of two immiscible melts in a mafic/ultramafic magma chamber: base-metal Ni–Cu-PGE sulfide deposits majority of global supply Ni, Cu PGEs as by-products Ni–Cu-PGE sulphides deposits formed from immiscible sulfide melt phases from a mafic and ultramafic silicatic melt Subdivided based on the major economic commodities and geological setting: 1. Base-metal Ni-Cu sulfide deposits in gabbroic rocks 2. Base metal Ni-dominant sulfide deposits in ultramafic lava flows (komatiites) 3. Precious metal, PGE magmatic sulfide deposits in large layered ultramafic-mafic intrusions. Two main types of Ni-Cu-PGE deposits: 1. Deposits shown in red are most important for their Ni content (subordinate PGE) 2. Deposits shown in blue are most important for their PGE content (subordinate Ni and Cu) Location of Ni-Cu-PGE deposits PGE global production and reserves 2019 Ni–Cu-PGE deposits formed from immiscible sulfide melt phases in mafic and ultramafic silicatic magma chamber Great Dyke, Zimbabwe Sudbury Igneous Complex, Ontario, Canada Noril’sk-Talnakh, Russian Federation Merensky Reef, Bushveld Complex What are immiscible sulfide melts? The generation of an immiscible sulfide liquid (melt) seems fundamental to forming these deposits. The attainment of sulfide saturation is necessary and the source of sulfur is not always obvious. Formation of immiscible sulfide melts Solubility of S in mafic melts varies with pressure and temperature and chemical composition S is a trace element in most magmas and pyrite is a common trace mineral The solubility of S in mafic magma is limited and decreases with decreasing T Formation of immiscible sulfide melts When a mafic silicatic mafic magma becomes sulfide-saturated, droplets of sulfide melts (liquid) will form and segregate from original silicate melt The sulfide droplets (immiscible melt) are heavier than original silicate melt, and tend to settle and accumulate at the bottom of the mafic/ultramafic magma chamber There are main 7 steps that have been identified as important in the formation of magmatic sulphide deposit 7 STAGES IN THE LIFE OF A MAGMATIC SULFIDE DEPOSIT Naldrett et al., (2010) South African Journal of Geology, 113 STAGE 1 : Mafic/ultramafic magma generation in the source partial melting of the mantle Naldrett et al., (2010) South African Journal of Geology, 113 STAGE 2 : Development of the magma generated mafic/ultramafic melt moves from the mantle into the crust Naldrett et al., (2010) South African Journal of Geology, 113 STAGE 3 : Fertilisation of the mafic magma Contamination of the rising mafic/ultramafic melt by partial melting of crustal material and/or interaction with the crust Cooling and saturation in S starts Saturation in S and formation of Sulfide immiscible melt droplets Naldrett et al., (2010) South African Journal of Geology, 113 STAGE 4 : Delivery Mafic silicate magma + immiscible sulphide liquid will emplace into the crust Naldrett et al., (2010) South African Journal of Geology, 113 STAGE 5 : Growth accumulation and concentration of sulphides in the magma chamber Naldrett et al., (2010) South African Journal of Geology, 113 STAGE 6: Nourishment Enrichment of sulfide and increase in high magma/sulphide ratio by new input of melt Naldrett et al., (2010) South African Journal of Geology, 113 STAGE 6: Maturity solidification of silicates & sulphides Naldrett et al., (2010) South African Journal of Geology, 113 Separation of immiscible sulfides melt from silicate mafic melts Mafic/ultramafic melts starts cooling in the crust If there is enough S, with cooling the mafic silicate magma may reach saturation in S at the moment of S saturation there is the formation of S-rich droplets S-rich melt from the original mafic silicate melt S-rich melt is called immiscible, because one is separated from the silicate melt, it cannot return back Immiscible sulfides melt and mineralisation: 1. The immiscible S-melt is dense and tends to sink at the bottom of the magma chamber and forming a Fe-Ni-rich MSS (monosulphide solid solution) All chalcophile elements present in the mafic melt will concentrate in the sulfide immiscible melt Os, Ir, Ru, Rh are compatible in MSS enter into the Ni-rich MSS Pt, Pd, Cu and Au are incompatible and stay in the Cu-rich melt Pt, Pd, Au Sulphide Cu-rich liquid liquid MSS Fe,Ni Os, Ir, Ru, Rh ca. 1000°C Immiscible sulfides melt and mineralisation: 2. Further cooling results in the formation of ISS (intermediate solid solution) from the Cu-rich liquid Pt, Pd, Au are incompatible in ISS and concentrate with other trace elements (e.g., Sb, Bi, Te, As), initially as a separate melt phase Pt, Pd, Au semi- metal-rich melt PGM ISS Cpy Cu-rich liquid Ni-MSS Pn MSS Fe-MSS Po PGM ca. 1000°C