Production Of Amino Acids PDF
Document Details
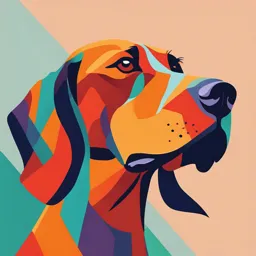
Uploaded by HeartfeltStrength
Dr. Joham Sarfraz Ali
Tags
Summary
This document discusses the production of amino acids, focusing on the role of bacteria like C. glutamicum. It covers topics such as categories of amino acids, strains used, and the importance of amino acid excretion.
Full Transcript
PRODUCTION OFAMINO ACIDS Dr. Joham Sarfraz Ali. Food Biotechnology “ BTE-682” Introduction Amino acids are the basic constituents of cellular proteins and important nutrients for all living cells. Their importance is closely related to their nutritional...
PRODUCTION OFAMINO ACIDS Dr. Joham Sarfraz Ali. Food Biotechnology “ BTE-682” Introduction Amino acids are the basic constituents of cellular proteins and important nutrients for all living cells. Their importance is closely related to their nutritional value, in particular for those amino acids that are essential for man and animals. (lysine, threonine, methionine, tryptophan, phenylalanine, valine, leucine, isoleucine, and, to some extent, arginine and histidine). Introduction Amino acids are used for various purposes, depending on their taste, nutritional value, other physiological activities, and chemical properties. Besides their application in human nutrition (flavor enhancers, sweeteners), they are used as feed supplements (animal nutrition), as well as for cosmetics, in the pharmaceutical industry, and as building blocks for chemical synthesis Introduction In addition to the importance of amino acids as food supplements, there is a huge market for amino acids as additives to animal feed. The basic goal is to improve the value of animal feed, which typically lacks the essential amino acids lysine, methionine, threonine, and tryptophan as well as other essential amino acids to a minor extent The economic driving force for manufacturing and adding amino acids to animal feed is twofold: 1. Improvement of the quality of feed leads to a reduction of the quantity needed, which leads to cost reduction. 2. Reduction of animal waste(manure), because the individual animal will take in as much food as necessary to obtain a sufficient amount of the most limiting nutrients, which, in general, are essential amino acids. Categories of Amino acids Categories of Amino Acid: Amino acids which are used in bulk quantities in food and feed applications can be divided into four categories: 1. Those that are mainly synthesized by chemical procedures (methionine) 2. Those that are synthesized by enzymatic synthesis, frequently using the enzymes of immobilized bacterial cells. (Aspartate). 3. Those that are produced by gene-tailored organisms. Glutamate, which is produced by C. glutamicum, represents the oldest biotechnological product in this field, and at the same time, the amino acid is produced in the largest quantities. 4. The rest of the amino acids, namely lysine, threonine, and phenylalanine,are produced by using fermentative techniques and microorganisms. Strains used A number of bacteria have been isolated that were also characterized by the peculiar capacity of excreting glutamate. Later, they were all reclassified as C. glutamicum. Corynebacterium glutamicum, a soil bacterium, belongs to the group of mycolic acid containing actinomycetes. After the successful introduction of C. glutamicum for commercial production of large quantities of amino acids, other bacteria, namely Escherichia coli, have also successfully been used for this purpose. Interestingly, a bacterium closely related to C. glutamicum, namely Corynebacterium ammoniagenes, is commercially used for the production of large quantities of nucleotides, another important flavor enhancer in food biotechnology Amino acid producing strain Amino acid producing strains can simply be classified into three different categories: 1. Wild-type strains able to excrete particular amino acids under specific culture conditions. 2. Regulatory mutants from which feedback control of amino acid biosynthesis has been removed. 3. Genetically modified strains in which the biosynthetic capacity has been amplified. Understanding how bacteria naturally release amino acids can help us produce amino acids more efficiently. In the past, people thought that bacteria didn't purposefully release amino acids, and it was just a result of certain techniques. Now, we know that many bacteria actually have a built-in ability to release amino acids, and we can use this natural process to our advantage when trying to make amino acids for various purposes. This new understanding allows scientists to create better and more effective methods for producing amino acids. Amino acid excretion There are actually at least two plausible reasons that may cause amino acid excretion under physiological conditions. As a soil bacterium, C. glutamicum uses all available materials from its natural surroundings as carbon and energy sources. Peptides, derived from decomposing organic matter, are certainly an important source for these needs, and, like most bacteria, C. glutamicum harbors efficient uptake systems for peptides. On the other hand, this organism is not very well equipped with efficient degradation systems for various amino acids, e.g., lysine, arginine, branched chain, and aromatic amino acids. If peptides are used as carbon and energy sources, amino acids close to the central metabolism, e.g., glutamate, aspartate, or alanine, are efficiently metabolized. Those amino acids that are not efficiently metabolized, however, will accumulate and will lead to problems due to high internal Concentrations In a broader sense, this concept may hold for any imbalanced situation in the cell that can be corrected via export activity ( 1. Metabolic imbalance) Overflow Metabolism If there happens to be an unlimited supply of carbon and nitrogen as well as of energy, and if at the same time some other metabolic limitation occurs which leads to decreased growth or even growth arrest, bacterial cells in general decide not to limit substrate uptake, but to continue the central metabolism at high speed and to waste the surplus of resources instead of switching down their metabolic activity to save nutrients. This situation is called “ 2.overflow metabolism” , It is typical for glutamate overproduction in C. glutamicum. Limitations leading to overflow mechanisms can naturally occur for different reasons or can be artificially introduced in various ways, including lack of essential metal ions or cofactors. This holds for glutamate production in C. glutamicum, where limitation may occur due to lack of the essential cofactor biotin. Export system Amino acids have been shown not to passively cross the permeability barrier of the plasma membrane under production conditions. It is accepted that most, if not all, amino acid production processes necessarily depend on the presence of active export systems In addition to the trivial argument that most amino acids simply cannot cross the phospholipid bilayer without the help of transport proteins, it is also important to keep the internal amino acid concentration sufficiently low by active extrusion in order to avoid undesired feedback inhibition. This can be achieved only by energy dependent, active export systems, which guarantee that in spite of high rates of biosynthesis as well as increasing external product concentrations, the cytoplasmic amino acid concentration will remain at a relatively low level, compatible with normal physiological situations. For bulk amino acid production, only a small number of microorganisms are used, namely C. glutamicum (glutamate, lysine, and aromatic amino acids), E. coli (threonine, aromatic amino acids, and cysteine), and, to some extent, Serratia marcescens (arginine and histidine). In view of this fact and on the basis of the available models explaining the physiological background of amino acid production, the question arises whether these organisms may have special capacities with respect to amino acid excretion. Escherichia. coli is on this list precisely because it is the major source of physiological and biochemical knowledge available There are already genetic tools at hand that directed the choice of this organism for its use in amino acid production. The same argument holds true for C. glutamicum at another level, given that this organism is presumably the best studied bacterium with respect to technical fermentation. In the context of bacteria for metabolite production, it's been suggested that those with less tightly regulated central and peripheral metabolism may be more suitable. This idea was proposed for bacteria like E. coli and S. marcescens. However, the success of E. coli in amino acid production contradicts this notion. Taken together, the reason for choosing a particular production organism does not seem to be directly derived from its physiological or genetic suitability for this purpose. The choice is obviously driven by the amount of genetic and physiological knowledge available, and by the recognition of a particular organism to be productive (C. glutamicum) or easy to handle (E. coli). Metabolic network Schematically, the metabolic network related to metabolite production can be divided into several individual components. Basically, we can discriminate four different major steps being involved: substrate uptake, central metabolic pathways, amino acid biosynthesis, and amino acid excretion. Furthermore, the integration into global regulatory networks such as carbon control and nitrogen control has to be considered, as well as the importance of cellular metabolic balances like energy balance and redox balance. Moreover, specific situations have to be taken into consideration, such as amino acid degradation pathways or product reuptake by amino acid uptake systems. Many studies have been done on the importance of central metabolic pathways in C. glutamicum for the efficient synthesis of products such as lysine or glutamate In these studies the core significance of anaplerotic reactions within this metabolic node has been demonstrated, making the set of anaplerotic reactions a particularly fascinating target. Two precursor metabolites oxaloacetate and pyruvate, which serve as precursors for about 35% of cell biomass and metabolic products, are generated in the anaplerotic node. It may be concluded that the anaplerotic node with its complex cenario of partly counteractive reactions is one of the “secrets” of C. glutamicum that explains its exceptional metabolic flexibility and versatility. PHYSIOLOGICAL, BIOCHEMICAL, AND GENETIC TOOLS USED TO IMPROVE AMINO ACID PRODUCTION In the past decades, classical approaches including many rounds of mutation, selection, and screening, in some cases combined with molecular biology techniques such as threonine production, have led to impressively efficient strains of C. glutamicum and E. coli that are used in amino acid production. After introduction of modern genetic tools and sophisticated methods for pathway analysis, the era of rational metabolic design was begun Now additional aspects have come into scientific focus, namely substrate uptake, precursor synthesis by central pathways, further steps within the anabolic pathway, redox and energy balance, and last but not least, active product excretion.At the beginning, straightforward molecular approaches such as direct deletion or overexpression of particular genes were, in general, not as successful as conservative methods of strain breeding. This is most likely due to the fact that optimum productivity with respect to a single anabolic end product, i.e., an amino acid, is based on fine tuning of the whole cellular network of pathways and regulation circuits. Transport Reactions: Transport reactions are important in amino acid production and are thus candidates for optimization in three different ways. First, highly active nutrient uptake is a prerequisite for efficient synthesis of amino acids Second, have to be actively secreted in order to be accumulated in the surrounding medium. Third the latter process may be counteracted by the activity of amino acid uptake systems. Several new families of transporters are known. All these families have a number of members found in the genomes of a variety of bacteria. It has been demonstrated that amino acid uptake systems may be of significance for amino acid production in various ways. Tryptophan production by C. glutamicum was found to be improved when the uptake system for aromatic amino acids was decreased in its activity. The advantage of decreasing the activity of amino acid uptake for production may be twofold: 1. A possible energy consuming futile cycle of energy dependent uptake and export can be avoided. 2. Feedback inhibition as well as repression by high internal amino acid concentrations will not occur. In addition to the list of targets for optimization, based on the dissection of metabolic and regulatory events, there are a number of additional aspects to consider.A highly interesting point which frequently is discussed in connection with strain optimization is the presence of auxotrophies for amino acids or related compounds in production strains. AMINO ACID PRODUCTION: TECHNICAL ASPECTS Among many techniques used namely extraction, chemical synthesis, enzymatic synthesis, and fermentation, only the latter, which, except for the production of methionine, is used for all large scale bulk amino acids, continuous culture. Fermentation techniques used can be divided into batch and fed batch cultures, and continuous culture. Industrial amino acid fermentation is mostly performed using the former two procedures. In general, fed batch processes, in which the substrate is not only provided at the beginning of the process but is added repeatedly during fermentation, lead to higher productivity as compared to batch processes By lowering the initial concentration of nutrients (sugar), the lag time can be shortened and growth as well as yield can be increased. This is mainly due to osmotic stress caused by high nutrient concentrations. Continuous fermentation is technically very demanding, as it requires continuous feeding of sterilized fresh media and sterile air. Furthermore, bacteria often undergo spontaneous mutations which, under the metabolic pressure of amino acid fermentation, may lead to reduced productivity during continuous culture for a long period of time. It is worth noting that continuous culture is in principle only possible if amino acid excretion occurs during growth. There are several processes in which the production phase is clearly separated from the growth phase. Because cells are constantly kept in the growth phase in continuous culture, this procedure would not easily be applicable in these cases. Apart from that, continuous culture is a much better tool for studying microbial physiology in response to environmental conditions than batch culture. Consequently, the information obtained from continuous culture experiments is useful for optimizing conditions and feeding strategies in fed batch process. Selected Examples of Amino Acids – Besides those amino acids which are currently of core interest in the food industry, e.g., glutamate, phenylalanine, aspartate, and cysteine, several other amino acids are used for different purposes, namely as feed additives and for the pharmaceutical and cosmetic industry. – Some of which will be discussed in today's lecture Glutamate – C. glutamicum is used, although E. coli and other organisms are also able – The most important amino acid in food biotechnology, and the first amino acid to be produced by fermentation, is glutamate (monosodium glutamate, MSG). – Currently, more than 1,000,000 tons of glutamate are produced annually. – L-glutamate is closely related to the central metabolism. Treatments leading to glutamate excretion – Several different kinds of treatments lead to glutamate excretion. – Based on the original observations, glutamate was found to be excreted under conditions of biotin limitation – Glutamate overproduction can also be induced by addition of some kind of detergents, e.g., polyoxyethylene sorbitane monopalmitate (Tween 40) or polyoxyethylene sorbitane monostearate (Tween 60). it is assumed that the detergents used exert a regulatory effect rather than a direct increase in permeability. – Addition of β-lactam antibiotics (penicillin), which are known to interact in cell wall biosynthesis, leads to glutamate excretion. – Strains which are auxotrophic for precursors required in phospholipid biosynthesis, such as glycerol or fatty acids, can excrete glutamate into the surrounding medium. – The apparently simple method of applying increased temperature also leads to increased glutamate excretion, in the case of temperature sensitive mutants – Recently, it was shown that direct changes in the architecture of the cell wall, brought about by disrupting enzymes involved in the synthesis of cell wall components in C. glutamicum, such as fatty acids or trehalose, lead to increased glutamate excretion, too. – General membrane acting factors, such as addition of local anaesthetics or application of osmotic stress, may induce glutamate excretion in C. glutamicum. L-phenylalanine – Phenylalanine production is particularly important because of its use in the synthesis of the low- calorie sweetener aspartame (methyl ester of the dipeptide L-aspartyl-L-phenylalanine), which is 150–200 times sweeter than sucrose. – Besides fermentation, phenylalanine is also produced using chemical synthesis. – For microbial production of L-phenylalanine mainly E. coli and C. glutamicum (and related species) are used. – Strains have been obtained which are able to produce up to 50g/l of L-phenylalanine. L-trytophan – L-tryptophan is an amino acid with increasing potential commercial interest. – It is currently mainly used as feed additive, despite its value as an essential amino acid, because of a number of casualties of eosinophilia myalgia syndrome (EMS) in humans which occurred due to the consumption of impure L-tryptophan manufactured by fermentation. – L-tryptophan is produced by strains of E. coli and C. glutamicum. – In order to obtain effective tryptophan producing organisms, similar alterations of precursor pathways and of the biosynthetic route as described above for phenylalanine production have been carried out. Threonine – Threonine is another member of the aspartate family of amino acids. – Like the essential amino acid lysine, threonine is mainly used as a feed additive. – Its ranked as third in the production volume with about 20,000 tons annually. – Development of threonine producers is interesting because of two major points. Development of threonine producing strains resembles a straightforward approach in combining classical strain breeding techniques with recombinant DNA technology. – Among these changes is the introduction of the capacity to use alternate carbon sources as well as the abolishment of amino acid degradation pathways. – Threonine is also an example where knowledge on substrate transport in general and on specific excretion systems in particular is available. Future Developments and Perspectives – Classical procedures of strain breeding by mutation combined with selection and screening procedures have proven in the past to be powerful tools for providing efficient amino acid producing strains. With easy access to modern molecular techniques, strain improvement on the basis of detailed biochemical knowledge becomes more and more important. – In the era of rational metabolic design, we need a combination of data and knowledge from many different fields for understanding bacterial metabolism. – Process optimization needs an increase in systemic knowledge of how a bacterial cell functions. – The availability of a large number of bacterial genomes, fostered the direct application of genomic information for the design of production strains. Genome annotation was helpful for defining the inventory of enzymes and pathways with putative biotechnological significance. Post genome approaches, i.e., transcriptome and proteome analysis, are currently applied with increasing frequency. – Genome breeding: Based on comparative genome analysis of wild-type and production strains, a set of mutations was identified by which these strains differ. Based on physiological and biochemical knowledge, mutations which were supposed to be relevant for amino acid production were selected and introduced step by step into the wild type strain. The aim of this strategy is to combine beneficial genetic properties identified in the genomes of various mutants into newly engineered producing strains. Future Developments and Perspectives – Metabolome data represents the basis for sophisticated metabolic flux analysis. Based on gas chromatography (GC)- or liquid chromatography-mass spectrometry (LC-MS) techniques. – Metabolic flux analysis through NMR, MS techniques provide a complete analysis of the dynamic situation of cell metabolism (the fluxome). – The information obtained by various levels of analyses mentioned here can be integrated into a systems biology approach only with the help of powerful bioinformatics tools. – Reaching the aim of an increase in the systemic understanding of cell metabolism, however, does not only require global approaches, but certainly depends to a large extent on additional information from biochemistry and physiology. E.g. Identification and analysis of specific export proteins to be responsible for amino acid excretion. – Further development in fermentation techniques is still a major option for improvement of amino acid production,based on detailed knowledge of biochemistry and physiology of the bacterial cell. Assignment Biotechnological Strategies for Reducing Food Waste in the Fresh Fruit Supply Chain