Bio 1M Evolutionary Processes (Complete) PDF
Document Details
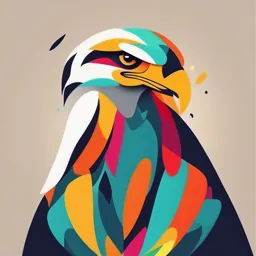
Uploaded by UnboundDemantoid
Tags
Summary
This document provides a summary of evolutionary processes, including concepts like selection, variation in traits, and allele frequencies.
Full Transcript
Bio 1M: Evolutionary processes (complete) Evolution by natural selection POLL What is the missing from the story I told last chapter? – Heritable variation in traits – Selection (i.e., differential reproductive success) based on these traits Answer: Where does heritable variation in traits come...
Bio 1M: Evolutionary processes (complete) Evolution by natural selection POLL What is the missing from the story I told last chapter? – Heritable variation in traits – Selection (i.e., differential reproductive success) based on these traits Answer: Where does heritable variation in traits come from? Some genetics Our basic traits are determined by genes A location where a gene can occur is called a locus (pl. loci) A particular version of a gene is called an allele Complex organisms usually have two alleles at each locus – These can be the same, or different Loci Complex organisms usually have two alleles at each locus – These can be the same, or different An organism with different alleles at a particular locus is referred to as heterozygous (adj., n. form heterozygote) An organism with two copies of the same allele at a particular locus is referred to as homozygous (adj., n. form homozygote) Evolution Heritable changes in species traits over time Driven by changes in allele frequencies POLL What processes cause evolution? – Answer postponed: 1 Analyzing genotype frequencies 1 Genotypes and phenotypes A genotype is the collection of an individual’s genes A phenotype is the collection of an individual’s physiological and physical traits – What we can observe about an individual – Phenotype is largely (but by no means entirely) determined by genotype Example: peppered moths Kettlewell’s experiment https://en.wikipedia.org/wiki/Kettlewell%27s_experiment Two different alleles possible at the wing color gene: A1 and A2 . – Individuals with A1 A1 genotype have light-winged phenotype – Individuals with A2 A2 genotype have dark-winged phenotype . – Individuals with A1 A2 genotype ??? If individuals with genotype XY have the same phenotype (on average) as those with XX, we say that X is a dominant allele and Y is a recessive allele. – We will call this simple dominance – This is rarely exactly true, but often close enough to true. Allele interactions When neither allele dominates, a variety of complicated things can happen. People also tell complicated stories about naming these things. We’re also not going to worry about that: – Co-dominance – Incomplete dominance – We will use complex dominance for anything that’s not very close to simple dominance Table 20.1 Analyzing genotype frequencies We analyze genotype frequencies as follows: – Make simple assumptions about how frequencies work – Calculate expected frequencies under our assumptions – Measure observed frequencies in the population – Look for evidence of systematic (not random) difference between expected and observed frequencies 2 Making simple assumptions Expected frequencies are usually calculated by assuming that alleles assort randomly and independently, like flipping two coins, or rolling two dice Activity: Coin flipping I flip two fair coins (ie., each coin will land heads with probability 1/2). What is the probability of: – Two heads – Two tails? – One of each? Answer: 1/4, 1/4, 1/2. Activity: Professional coin flipping A professional gambler can flip a coin so that it lands heads 70% of the time. She flips two coins. What is the probability of: – Two heads – Two tails? – One of each? Answer: 0.49, 0.09, 0.42 Hardy-Weinberg distribution The Hardy-Weinberg distribution is the distribution expected if alleles work like coins (random and independent). If p is frequency of allele A1 and q is frequency of allele A2 , then: – Frequency of genotype A1 A1 is p2 . – Frequency of genotype A2 A2 is q 2 . – Frequency of genotype A1 A2 is 2pq. Why the 2? – Answer: Because you could get A1 from Mom and A2 from Dad, or A1 from Dad and A2 from Mom . . . two ways to do it 3 Example: calculating allele frequencies I collect 20 peppered moths from a particular place, and find that 4 have genotype A1 A1 , 8 have genotype A1 A2 , and 8 have genotype A2 A2 . What is the observed frequency of each allele? What is the expected frequency of each genotype under the Hardy-Weinberg assumptions? Is this population in Hardy-Weinberg equilibrium? – Answer: We see more homozygotes than expected * Answer: We can always summarize as more or less homozygotes * Answer: if allele frequency is right – Answer: But is this reliable evidence? That’s a question for statistics. What do we mean by expected? If we flip a fair coin 100 times, what is the expected number of heads? – What if we flip it 25 times? We don’t expect to get exactly the expected value. The ‘expected value’ is an average of what is expected under our assumptions – The idea is a conceptual average over what would happen if we did the same experiment many times How do you know a coin is perfectly fair? You can never be sure that a coin is perfectly fair, you can only evaluate your evidence that it’s more or less close to fair. Similarly, we never have evidence that a population is exactly in Hardy-Weinberg equilibrium We can only evaluate our evidence that it is far from (or close to) equilibrium What’s another way to think about the evidence? – Answer: How clear is it that we really have more (or less) homozygotes than expected? 4 Hardy-Weinberg equilibrium When do we expect genotype frequencies to behave like coins? Alleles selected at random from the previous generation: – Answer: Random mating within a closed population – Answer: No differences in fitness between genotypes – Answer: No mutation, no drift (see below) If these assumptions hold exactly, we expect Hardy-Weinberg equilibrium – Hardy-Weinberg distribution, with no change in allele frequencies from generation to generation. This never happens Differences from equilibrium If we observe large differences from the Hardy-Weinberg equilibrium, this is usually a sign that mating is not random, or that natural selection is operating The analysis tells us that something is going on, but not what Hardy-Weinberg is a null model: it tells us what to expect if complicating effects are absent Without a null model, we couldn’t ask how do observations differ from expectations Example: Human blood groups MN blood groups in different human populations are very close to Hardy-Weinberg equilibrium – https://tinyurl.com/MN-morning-2023 – https://tinyurl.com/MN-afternoon-2023 – No evidence for non-random mating, or for fitness differences. – This does not mean it’s not happening, but probably means that it’s small – Especially when we observe the same thing many times 5 Activity: Human blood groups at the global level POLL At the global level, how will MN blood groups compare to HW expectations? – Answer postponed: POLL What did we find? – Answer: More homozygotes POLL Why? – Answer: Mostly because mating is not random – Answer: These data are telling us different (reasonable) stories at different scales Example: Human HLA genes HLA genes are used by the immune system to recognize disease-causing organisms Researchers hypothesized that heterozygous individuals may recognize more bacteria and viruses Data shows that more people are heterozygous for HLA genes than would be expected under the Hardy-Weinberg assumption Heterozygous HLA genes POLL Why might more people be heterozygous for HLA genes than predicted by Hardy-Weinberg? – Answer: Heterozygous people might be more likely to survive – Answer: Heterozygous people may have more offspring * Answer: Effects of this one are more complicated * Answer: Heterozygotes don’t necessarily have heterozygous offspring – Answer: People might be more attracted to people with different HLA types * Answer: Maybe evolved this way because of reasons above 2 Types of natural selection 2.1 Trait level Directional selection Directional selection tends to move a population in a particular direction – Giraffe necks – Human brains 6 Multi-directional selection Directional selection can change through time with the environment – Swallows may get larger during extreme cold spells, smaller again during normal weather * But we need to know whether the changes we saw were heritable – Finch beaks get thicker when food is scarce, and smaller when food is abundant Why might small-beaked finches have advantages? – Answer: May be faster at processing small seeds – Answer: Can use the resources for something else * Answer: Faster growth, more fat storage Stabilizing selection Stabilizing selection tends to keep the population where it is – Answer: Usually because population is already adapted Connections between selection types What happens if the target of directional selection stays the same for a long time? – Answer: The population arrives at the target, and directional selection becomes stabilizing selection Examples? – Answer: Giraffe necks – Answer: Human brains – Answer: Almost everything we see * Answer: Things often develop by directional selection, but at any given time, most of what we see is under stabilizing selection * Answer: Because most organisms are highly adapted Disruptive selection Disruptive selection favors phenotypes different from the average value – Black-bellied seedcrackers have different types with different bill sizes. * Big bills may be good for big seeds and small for small * There may not be a lot of medium seeds in many forests – Animals that get eaten a lot (prey) may want to look different from their peers so that the predators that eat them don’t find them as easily Disruptive selection can lead to speciation – the formation of new species. Comment: See also F20.9 7 Frequency dependence Disruptive selection is closely related to frequency dependence Frequency dependence is the idea that some trait types do relatively better if they are rare. 2.2 Allele level Positive/negative selection An allele that has greater fitness than others in a particular context is called advantageous – It will tend to increase due to positive (natural) selection An allele that has less fitness than others in a particular context is called deleterious – It will tend to decrease due to negative (natural) selection The context can change when the environment changes, or . . . – Answer: When other alleles change Balancing selection Balancing selection tends to maintain allele diversity – When there is no single best allele Disruptive selection at the trait level will always cause some balancing selection – Answer: If natural selection is pushing in different directions, there must be some different alleles involved – Comment: Not 100% true, see seedcrackers Example below Balancing selection can also be caused by heterozygote advantage: when heterozygotes have higher fitness – Answer: The only way to get a heterozygote is by having different alleles combine Example: The sickle cell phenotype Blood cells that can lose their shape and squash malaria parasites! – People heterozygous for this trait get less sick with malaria – People homozygous for this trait have too much instability and severe anemia This is an example of: – Answer: heterozygote advantage 8 Example: seedcrackers What would happen if almost all of the seedcrackers had large bills? – Answer: More small seeds available, small bills become an advantage, an example of . . . * Answer: frequency dependence * Answer: disruptive selection What happens when large-billed and small-billed individuals breed? – Answer: They could have low-fitness offspring – Answer: Can lead to selection for less heritability – Answer: or selection on mate choice Alleles and traits Most traits that we measure depend on many alleles from many different loci We learn a lot from simple traits that depend on a single locus – Simple to study – Simple to explain Changes at one locus can affect the selection environment at another locus 3 Other evolutionary mechanisms 3.1 Genetic drift Genetic drift is change in allele frequencies due to random sampling: – Some individuals have more offspring than others due to chance events – Offspring receive certain parental alleles, and not others These factors will lead to an accumulation of random changes in allele frequencies Drift is a likely reason why different human populations have different MN allele frequencies Thought experiment Imagine flipping a fair coin 100 times – Repeat Now imagine choosing 100 alleles at random (with replacement) from a population of 50 A and 50 B alleles – Repeat, using new population as a starting point 9 Small populations Drift is much stronger in small populations than in large ones (law of averages). Even if a population is big now, it may have been small in the past – Founder effects occur when a new population is started by a small number of individuals – Bottlenecks occur when a population becomes small, then large again * ... or, when a beneficial genetic mutation takes over a population * Answer: variation will be lost at that locus because the new gene is better * Answer: but it can also be lost at other loci at random, because the whole future population is descended from individuals with the new mutation Fixation and loss An allele may drift to a frequency of 0 (it’s lost) or of 1 (it’s fixed) Advantageous alleles are often (not always) fixed – Answer: Positive selection Disadvantageous alleles are usually (not always) lost – Answer: Negative selection Alleles with neutral differences (no selective difference) will be fixed or lost at random – This is also true for alleles with small effects! Drift tends to reduce genetic variation 3.2 Gene flow Gene flow is the movement of alleles from one population to another – This happens when individuals move from one population to another and breed How we think about gene flow depends on how we choose to define a ‘population’ Gene flow can be an obstacle to speciation; it helps keep populations similar 10 3.3 Mutation Mutations are heritable errors in copying DNA Mutations are rare; by themselves they don’t cause much evolution Mutations are extremely important to evolution, however: – Answer: Mutations provide the variation on which natural selection acts – Answer: Mutation is the only source of new alleles Types of mutations Mutations can occur at many scales: – a single DNA base might change – chunks of DNA can be added or subtracted – whole genes (or whole chromosomes) can be duplicated New genetic sequence can come from: – copying errors – other organisms! lateral gene transfer Mutations are random Most mutations are deleterious – bad for fitness Very rarely mutations are beneficial – good for fitness – Such mutations are favored by natural selection Complex organisms Can complex organisms arise through random mutations? – A central question of biology – Large-scale evolution takes a long time – Beneficial changes can accumulate gradually – Much evidence of intermediate forms Evolution of the eye video https://www.pbslearningmedia.org/resource/tdc02. sci.life.evo.nilssoneye/evolution-of-the-eye/ 11 What about sex? Sex does not directly change allele frequencies It does act to bring alleles together (and to split them apart), this is called recombination Sex is not a source of new alleles – Comment: Depending on how we define alleles – But it is a source of new combinations There is still active debate on the advantages and disadvantages of sex in evolution 4 Mating patterns 4.1 Inbreeding Inbreeding refers to mating between close relatives Since relatives will tend to share similar alleles, inbred populations will tend to differ from Hardy-Weinberg equilibrium in what way? – Answer: More homozygous loci Inbreeding depression In many populations, it is observed that inbred individuals have lower fitness: – They are more likely to be homozygous for rare genetic defects – They are less likely to be heterozygous for immune-system genes Inbreeding depression is a serious concern for conservation – As populations get smaller, inbreeding becomes more common Wildlife studies show that panthers with both parents from Florida (small population) do not survive well Human demographic studies show strikingly lower survival for children of first cousins 4.2 Sexual selection Sexual selection is a form of natural selection Occurs when there is heritable variation in traits related to success in obtaining mates 12 Example: Pukeko Studied by Quinn lab here at Mac Hypothesis: Bright crests make males more attractive to females Activity: Pukeko experiments POLL How would you test this hypothesis? – Answer: Give some males bigger or brighter crests * Answer: Paint, cardboard, dietary supplement – Answer: Test whether they are preferred by females Pukeko analysis Why not simply find and use birds with naturally better crests? – Answer: The crests may be better because they differ in other ways (bigger, healthier, etc.) – Answer: We always want to make groups as similar as we can What is a concern with the methods proposed? What could you do about it? – Answer: Whatever we do to improve the crests may have other effects – Answer: Try to compensate and make treatment and control as similar as possible Why the males? Males often have striking traits that females lack, used in courtship, or in battles for mates – Sexual dimorphism refers to trait differences between males and females Why do males more often have these traits than females? – Investment in reproduction – Variation in reproductive success Investment in reproduction In many species, females invest much more in each offspring than males do – Eggs are expensive, sperm are cheap – Females are often more involved in caring for offspring If females invest a lot in each offspring, they can maximize fitness by being choosy about mates If males invest little in each offspring, they can maximize fitness by mating as much as possible 13 Testing the theory POLL How might we test the theory that males compete more sexually because females invest more in offspring? – Answer: Are there any species where these roles seem to be reversed? * Answer: Yes, in some species of pipefish (related to seahorses) the males spend more time and energy caring for young them females – Answer: In these species, do females compete for males? * Answer: Yes, females are larger than males, and develop bright colors at courtship time Variation in reproductive success Males often have greater variation in reproductive success than females do This is a side-effect of the fact that females usually invest a lot in each offspring – Reduces potential total number of offspring – Makes females desirable to males Greater variation in reproductive success means that winning contests is more important to male than female fitness Example: elephant seals Male elephant seals compete for control of breeding beaches Huge variation in reproductive success Huge size difference between males and females (strong sexual dimorphism) Conclusions Mutation (mistakes!) is the source of new variation Natural selection drives adaptation: selects variation that allows organisms to thrive in diverse settings Sex facilitates new combinations, but sexual selection can work against adaptation to the environment Genetic drift and gene flow are also non-adaptive drivers of evolution The organisms we see are the result of all of these processes: – adaptive, non-adaptive, previously adaptive © 2017–2023, Jonathan Dushoff and the 1M teaching team. May be reproduced and distributed, with this notice, for non-commercial purposes only. 14