Problem Set PDF
Document Details
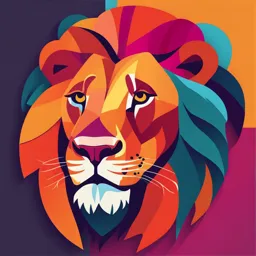
Uploaded by TransparentManticore390
Tags
Summary
This document provides a detailed overview of various biological processes. Topics including cell receptors, hormones, neurotransmitters and signaling mechanisms are explored in depth. The content is suitable for a biology course at the undergraduate level.
Full Transcript
1. Cell receptors are proteins with binding sites for specific signaling chemicals, the signaling chemicals is called a ligand. 2. Hormones, neurotransmitters, cytokines and calcium ions as examples of functional categories of signaling chemicals in animals. Chemical signaling involves var...
1. Cell receptors are proteins with binding sites for specific signaling chemicals, the signaling chemicals is called a ligand. 2. Hormones, neurotransmitters, cytokines and calcium ions as examples of functional categories of signaling chemicals in animals. Chemical signaling involves various molecules that facilitate communication between cells, each with distinct roles and mechanisms. Hormones are secreted by endocrine glands into the bloodstream and act over long distances to regulate systemic processes like metabolism and reproduction. In contrast, neurotransmitters are released by neurons and act locally across synapses to transmit signals to nearby neurons, muscles, or glands, often mediating rapid responses. Cytokines, primarily produced by immune cells, can act in autocrine, paracrine, or endocrine manners to modulate immune responses and inflammation; they differ from hormones in their broader range of producing cells and lower concentrations. Finally, calcium ions (Ca²⁺) function as intracellular second messengers, mediating processes like muscle contraction and neurotransmitter release by amplifying signals within cells. While hormones and cytokines can act over long distances, neurotransmitters and calcium ions typically operate locally or intracellularly, highlighting the diversity in chemical signaling mechanisms. 3. Hormones are classified into three main chemical categories: amine hormones, protein (or peptide) hormones, and steroid hormones, each with distinct structures and mechanisms of action. Amine hormones are derived from amino acids like tyrosine or tryptophan. Examples include epinephrine and norepinephrine, which are secreted by the adrenal medulla to mediate the fight-or-flight response, and melatonin, produced by the pineal gland to regulate circadian rhythms. These hormones can bind to cell surface receptors or intracellular receptors depending on their solubility. Protein hormones, composed of chains of amino acids, include short peptides like antidiuretic hormone (ADH) and larger proteins like insulin and growth hormone. They are water-soluble and bind to cell surface receptors, initiating signaling cascades via second messengers. In contrast, steroid hormones are lipid-derived molecules synthesized from cholesterol. Examples include cortisol, which regulates stress responses, and sex hormones like testosterone and estrogen. Being lipophilic, steroid hormones diffuse across cell membranes and bind to intracellular receptors, directly influencing gene expression. This classification highlights the diversity in hormone structure and function, tailored to specific physiological roles. 4. Neurotransmitters encompass a wide range of chemical substances that facilitate communication between neurons. Amino acids are a major class, including excitatory neurotransmitters like glutamate and aspartate, which activate post-synaptic cells, and inhibitory ones like GABA and glycine, which suppress neural activity. Peptides, such as substance P, oxytocin, and beta-endorphin, are larger molecules often co-released with other neurotransmitters. They act on G-protein-coupled receptors and have diverse effects, including modulating synaptic transmission and influencing long-term processes like gene expression. Amines, derived from amino acids, include monoamines like dopamine, serotonin, and norepinephrine, which regulate mood, arousal, and attention. Acetylcholine is another key amine involved in muscle activation and cortical plasticity. Lastly, nitric oxide (NO) is a unique gaseous neurotransmitter that acts as a retrograde messenger, diffusing across membranes to modulate synaptic activity via cGMP signaling pathways. These diverse neurotransmitter types highlight the complexity of neural communication. 5. Quorum sensing allows Vibrio fischeri to regulate bioluminescence based on population density: as bacteria grow, autoinducer concentrations increase, and once a threshold is reached, the lux operon is activated, leading to light production. This bioluminescence is crucial for the mutualistic relationship with the Hawaiian bobtail squid, providing counter-illumination camouflage to protect the squid from predators. High bacterial density within the squid's light organ is essential to reach the autoinducer threshold, and a daily cycle of bacterial expulsion and regrowth maintains this symbiotic relationship. 6. Localized signaling involves neurotransmitters in the nervous system, which diffuse across the synaptic gap to act on nearby cells over a short distance (e.g., synaptic or paracrine signaling). In contrast, distant signaling in the endocrine system uses hormones transported via the blood system to reach distant target cells, where they bind to specific hormone receptors, producing slower but widespread effects. Other types include autocrine (self-signaling) and juxtacrine (direct cell-to-cell contact). 7. Transmembrane receptors and intracellular receptors differ significantly in their structure and function. Transmembrane receptors are located in the plasma membrane and consist of hydrophilic amino acids in their extracellular and intracellular domains, with hydrophobic amino acids forming the transmembrane domain. They have an extracellular ligand binding domain that interacts with water-soluble ligands, which remain outside the cell. In contrast, intracellular receptors are found in the cytoplasm or nucleus and are composed entirely of hydrophilic amino acids. These receptors bind water-insoluble ligands that can enter the cell, requiring the signaling chemical to pass through the plasma membrane before interacting with the receptor. While transmembrane receptors transduce signals across the membrane without the signaling chemical entering the cell, intracellular receptors necessitate the entry of the signaling molecule for binding and activation. 8. Signal transduction pathways are initiated by the binding of a ligand to a specific receptor, triggering receptor activation. This activation leads to a cascade of events that amplify the signal within the cell. In many cases, receptor activation stimulates the production of second messengers, such as cyclic AMP or calcium ions, which diffuse rapidly throughout the cell. These second messengers often activate protein kinases, enzymes that phosphorylate other proteins, creating a phosphorylation cascade that further amplifies the signal. This amplification process ultimately results in a cellular response, which can include changes in gene expression, alterations in metabolic pathways, or modifications to cell structure and function. The signal is eventually terminated through various mechanisms, including the degradation of second messengers or the inactivation of signaling proteins, ensuring that the cell can respond to new signals and maintain homeostasis. 9. At the neuromuscular junction, the process begins with acetylcholine released from a motor neuron, diffusing across the synapse to bind to the acetylcholine receptor, an integral protein functioning as a ligand-gated ion channel on the motor end plate of the muscle cell. This binding causes the channel to open, allowing sodium ions to flow into the postsynaptic cell and causing depolarization, making the voltage across the plasma membrane more positive. If sufficient, this depolarization triggers an action potential that travels along the muscle fiber, resulting in muscle contraction. The signal terminates when acetylcholinesterase breaks down the acetylcholine in the synaptic cleft, ensuring controlled muscle activity. 10.Epinephrine, a key hormone in the "fight or flight" response, binds to G protein-coupled receptors (GPCRs) on liver cells, triggering a signaling cascade. This binding activates the G protein, causing its alpha subunit to separate and form an alpha-GTP complex, which then activates adenylate cyclase. Adenylate cyclase converts ATP into cyclic AMP (cAMP), a second messenger that activates protein kinase A (PKA). PKA phosphorylates enzymes that catalyze the hydrolysis of glycogen into glucose, rapidly mobilizing energy to prepare the body for immediate action during stressful situations. 11.The mechanism of action of transmembrane insulin receptors with tyrosine kinase activity begins when the insulin hormone binds to the receptor, causing the receptor molecules to dimerize. This activates the receptor's kinase domain through autophosphorylation, initiating a series of reactions in downstream signaling pathways. One key outcome is the translocation of GLUT-4 from intracellular vesicles to the plasma membrane, where the vesicles fuse with the plasma membrane, and GLUT-4 is incorporated into it. This allows glucose to enter the cell, facilitating glucose uptake and metabolism. 12.Steroid hormones like estradiol, progesterone, and testosterone diffuse across the plasma membrane and bind to intracellular receptors in the cytoplasm or nucleus. This binding activates the receptor, allowing it to interact with a specific DNA binding site on the receptor. The activated receptor binds to DNA sequences, acting as a transcription factor to regulate gene expression. This process alters mRNA synthesis, leading to changes in protein production that control various cellular functions. 13.Positive and negative feedback mechanisms play crucial roles in regulating cell signaling pathways, each with distinct effects on cellular responses. Negative feedback loops typically maintain homeostasis by resisting changes in the system. For example, in calcium signaling, rapid Ca2+ uptake by mitochondria triggers negative feedback to limit and stabilize cytosolic Ca2+ concentration, even when the uptake signal persists. This helps prevent excessive calcium levels that could be harmful to the cell. In contrast, positive feedback loops amplify changes, often leading to rapid and significant responses. An example is the ripening of fruit, where the first ripe apple produces ethylene gas, which triggers nearby apples to ripen and produce more ethylene, creating a cascading effect throughout the tree. This amplification allows for coordinated ripening of fruit. Both types of feedback are essential for fine-tuning cellular responses to various stimuli and maintaining appropriate signaling dynamics. 14.Progesterone, secreted by the corpus luteum post-ovulation, acts on the uterus to prepare it for potential implantation. It promotes the differentiation and proliferation of endometrial cells, increasing the vascularization and thickness of the endometrium. Additionally, progesterone stimulates these cells to secrete nutrients, creating a supportive environment for embryo implantation and early development. 15.During days 12–14 of the menstrual cycle, estradiol, secreted by the developing follicle, reaches high levels and triggers a positive feedback mechanism. This increases the secretion of GnRH by the hypothalamus, which stimulates the pituitary to release more FSH. The elevated FSH further enhances estradiol production by the follicle, creating a reinforcing loop. Additionally, the positive feedback causes a surge of LH from the pituitary, leading to ovulation. This process ensures the release of a mature egg for potential fertilization. 16.During the luteal phase of the menstrual cycle, low levels of estradiol exert negative feedback effects on target cells. This negative feedback mechanism inhibits the secretion of GnRH by the hypothalamus, which in turn leads to reduced secretion of FSH and LH from the pituitary gland. As a result, follicle development and ovulation are suppressed. This process helps maintain hormonal balance and prevents the development of new follicles while the corpus luteum is active. The negative feedback effects of estradiol play a crucial role in regulating the menstrual cycle and ensuring proper timing of ovulation 17.The resting potential is the electrical difference across a neuron's membrane when it is not firing, with the inside being more negative than the outside (approximately -70 mV). It is established and maintained by the sodium-potassium pump, which actively transports three sodium ions out of the neuron and two potassium ions into the neuron. This creates an imbalance of ions, with more sodium outside and more potassium inside. The membrane's selective permeability allows some potassium to leak out, further contributing to the negative charge inside. This process requires energy and ensures the neuron is ready to transmit signals. 18.Ion channels play a critical role in depolarization and repolarization during an action potential. At resting potential (-70 mV), the membrane is stable until an initial stimulus causes the membrane potential to reach the threshold (-55 mV). This triggers voltage-gated sodium channels to open, allowing sodium ions to enter the cell by diffusion, causing depolarization as the inside of the membrane becomes more positive. Once depolarization peaks, the sodium channels become inactivated, and voltage-gated potassium channels open, allowing potassium ions to exit the cell. This outflow of potassium restores the negative charge inside the membrane, leading to repolarization and completing the action potential cycle. 19.The propagation of an action potential along a nerve fiber is driven by local currents. An initial stimulus causes a slight depolarization of the membrane, which opens voltage-gated sodium ion channels. As sodium enters the cell, it creates a local electrical current that spreads to the neighboring region of the membrane, causing it to depolarize. If this depolarization reaches the threshold, it triggers an action potential in that region. This process follows the all-or-nothing principle, meaning the action potential either occurs fully or not at all. The signal spreads down the axon in one direction due to the refractory period of the previously excited regions, preventing backward propagation. This sequence of events continues along the length of the axon, allowing the action potential to travel efficiently along the nerve fiber. 20.Saltatory conduction allows faster conduction of impulses along myelinated axons through a specialized structure and process. Schwann cells wrap around the axon, forming a myelin sheath that insulates the axon and prevents ion leakage. This insulation is interrupted at regular intervals by nodes of Ranvier, where ion pumps and channels are clustered. As an action potential reaches a node, it triggers the opening of these channels, causing a rapid influx of ions that regenerates the signal. The action potential then effectively "jumps" to the next node, skipping the myelinated segments in between. This jumping action, known as saltatory conduction, significantly increases the speed of impulse transmission compared to unmyelinated axons, as the signal doesn't need to be regenerated continuously along the entire length of the axon. 21.Signals travel across a synapse when an action potential reaches the axon terminal of the presynaptic neuron, causing calcium channels to open. The influx of calcium triggers vesicles containing neurotransmitter to fuse with the cell membrane, releasing the neurotransmitter into the synaptic cleft. The neurotransmitter molecules then diffuse across the synaptic cleft and bind to specific receptors on the postsynaptic cell, converting the original electrical signal into a chemical signal and initiating a response in the postsynaptic cell. 22.Neonicotinoids are a class of insecticides that target acetylcholine receptors in the nervous system. These compounds bind irreversibly to the acetylcholine receptors, effectively blocking synaptic transmission at cholinergic synapses. Unlike acetylcholine, which is normally broken down by acetylcholinesterase, neonicotinoids cannot be degraded by this enzyme, leading to a persistent disruption of neural signaling. This prolonged interference with synaptic transmission results in paralysis and ultimately death in insects. The potency of neonicotinoids stems from their ability to selectively bind to insect acetylcholine receptors with higher affinity than to mammalian receptors, making them particularly effective as insecticides while posing lower risks to non-target species. 23.Cocaine affects synaptic transmission in the reward pathway by binding to dopamine reuptake transporters, preventing dopamine from being cleared from the synaptic cleft. This causes dopamine to build up in the synapse, enhancing its activity at receptors. The accumulation of dopamine leads to feelings of being euphoric and energetic, which are key features of cocaine's effects. This mechanism underlies the drug's ability to reinforce addictive behaviors. 24.Excitatory and inhibitory neurotransmitters differ in their effects on the postsynaptic neuron. Excitatory neurotransmitters cause depolarization of the postsynaptic cell membrane, making it more likely to send an action potential, as seen with glutamate. In contrast, inhibitory neurotransmitters like GABA cause hyperpolarization of the postsynaptic cell membrane, making it less likely to send an action potential. The summation at the axon hillock combines the effects of multiple excitatory and inhibitory stimuli from multiple presynaptic neurons. If the combined signals reach the threshold potential, an action potential is triggered due to the all-or-nothing principle. This balance ensures precise control of neuronal activity. 25.Neurons with free nerve endings in the skin perceive pain through ion channels that respond to stimuli such as high temperature, acid, or chemicals. These channels open, allowing positively charged ions to enter the neuron, causing depolarization. As the membrane potential becomes more positive and moves closer to threshold, an action potential is triggered. This action potential travels down the axon to the brain, where the signal is processed, and pain is perceived. 26.Consciousness is a prime example of an emergent property, as it arises through the complex interactions of neurons and neural networks in the brain, yet is not present in the individual component parts. This phenomenon demonstrates how the collective behavior of simpler elements can give rise to a more complex and sophisticated property that cannot be explained by examining each part in isolation. Another example of an emergent property is the behavior of ant colonies. While individual ants follow simple rules, the colony as a whole exhibits complex problem-solving abilities and organizational structures that are not present in any single ant. Both consciousness and ant colony behavior illustrate how emergent properties arise through interaction and cannot be fully understood by studying the component parts individually. 27.Osmoregulation and excretion are distinct but related processes carried out by the kidney. Osmoregulation involves the regulation of osmotic concentration, measured in osmoles per liter, to maintain homeostasis of water and electrolytes in the body. Excretion, on the other hand, is the removal of waste products of metabolism, particularly nitrogenous waste produced by protein breakdown. The kidney plays a crucial role in both processes by filtering blood and producing urine. For osmoregulation, the kidney adjusts the concentration of urine to be more dilute or less dilute, depending on the body's needs, to maintain proper fluid balance. In terms of excretion, the kidney removes urea and other metabolic wastes from the blood, concentrating them in urine for elimination from the body. Through these functions, the kidney helps maintain both the proper osmotic balance and the removal of potentially harmful waste products. 28.Ultrafiltration occurs in the nephron, the functional unit of the kidney. The process begins in the glomerulus, a cluster of capillaries where blood flows at high pressure. This pressure forces water and small solutes out of the blood plasma through the capillary walls. The resulting filtrate contains toxins such as urea, as well as nutrients like glucose and salt. This fluid then enters the Bowman's capsule, which surrounds the glomerulus. The high pressure in the glomerulus, combined with the selective permeability of the capillary walls, ensures that larger molecules like proteins and blood cells remain in the bloodstream while smaller molecules pass through to form the filtrate. This initial filtration step is crucial for removing waste products and regulating the body's fluid and electrolyte balance. 29.The proximal convoluted tubule plays a vital role in reabsorbing important substances from the filtrate back into the blood. Through active transport of sodium, sodium ions are moved out of the tubule, creating an electrochemical gradient. This gradient drives co-transport mechanisms that reabsorb glucose and amino acids alongside sodium. These reabsorbed substances are then returned to blood plasma in peritubular capillaries, ensuring essential nutrients and ions are retained while waste is excreted. 30.The loop of Henle and collecting ducts play key roles in water reabsorption. In the ascending limb, the active transport of sodium ions creates a high osmotic concentration in the kidney medulla, while this limb is impermeable to water. This gradient drives osmosis in the descending limb and later in the collecting ducts, where water is reabsorbed. In the collecting ducts, water reabsorption is regulated by hormones like ADH, ensuring the body maintains proper hydration and concentrates urine effectively. 31.Osmoregulation in the collecting ducts involves the regulation of water reabsorption. Osmoreceptors in the hypothalamus detect increased osmolarity during dehydration, triggering the secretion of antidiuretic hormone (ADH) by the pituitary gland. ADH promotes the movement of aquaporins from intracellular vesicles to the cell membrane of collecting duct cells. These aquaporins are incorporated into the membrane, increasing its permeability to water. As a result, more water is reabsorbed, reducing water loss and concentrating the urine.