PMB313.docx
Document Details
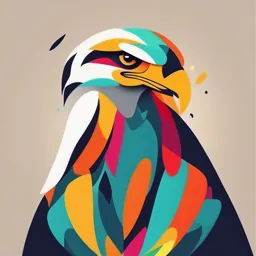
Uploaded by ComfyRomanArt
University of Benin
Tags
Full Transcript
**Department of Pharmaceutical Microbiology,** **Faculty of Pharmacy, University of Benin, Benin City.** **Dr. Godfrey E. Umhenin(B.Pharm, PharmD, M.Sc, MPSN)** [**godfrey.umhenin\@uniben.edu**](mailto:[email protected]) **COURSE CODE: PMB313** **Learning objectives:** At the end of t...
**Department of Pharmaceutical Microbiology,** **Faculty of Pharmacy, University of Benin, Benin City.** **Dr. Godfrey E. Umhenin(B.Pharm, PharmD, M.Sc, MPSN)** [**godfrey.umhenin\@uniben.edu**](mailto:[email protected]) **COURSE CODE: PMB313** **Learning objectives:** At the end of this module, students should be aware of: - The meaning of Sterilisation - Choice of methods for manufacturing a sterile product - Desirable properties of an ideal sterilisation method - Validation of sterilisation process - The various types of sterilisation method - Biological indicators - Reliable in terms of achieving the required sterility assurance level of 10^-6^ - Safe for the operators - Safe in terms of inducing damage to the product or its container or inducing the formation of toxic residues - An easily understood process that can readily be controlled and monitored by physical instruments - Short exposure time - Low cost This is the most commonly used terminal sterilization method and, together with ionizing radiation, the most reliable. The autoclaves used for steam sterilization vary in size from bench top models of the type commonly found in dental surgeries to large floor-standing industrial machines that are loaded with forklift trucks. Autoclaves used in quality assurance laboratories and hospitals typically have chambers of 0.2--0.5m^3^ -- about the capacity of a large refrigerator. Benchtop and smaller, floor standing machines usually generate their own steam from purified water added before the sterilization cycle starts, whereas the larger models are usually supplied with steam from an external boiler. Autoclaves can have circular or rectangular chambers, be top-loading or front-loading and have doors at one end or, less frequently, at both ends of the chamber (to permit transfer of materials from a non sterile to a sterile area for example). The doors of modern autoclaves are fitted with thermal locks which prevent the door being opened until the chamber contents are below a preset temperature, for example 80^0^C; this affords greater operator safety but substantially increases the cycle time compared with older machines without this facility. Some autoclaves have an external jacket surrounding the chamber; this may be filled with steam to afford insulation to the chamber itself which, in turn, may result in faster heat-up times. Steam is very much better as a sterilizing agent than water at the same temperature, because steam has a high latent heat content which is transferred to the objects being sterilized when the steam condenses on them. Consequently, steam quality is particularly important: it should be dry (containing no liquid water droplets) and saturated (containing the maximum amount of water vapour possible for the given temperature). Superheating should be avoided; this occurs when the steam is not in equilibrium with the water from which it was generated, for example where the steam pressure is rapidly reduced without a corresponding fall in temperature, or where the pressure is kept constant but the steam temperature is artificially raised (because this would mean the steam was no longer saturated). Steam releases its latent heat on contact with the cooler items to be sterilized in the autoclave, but superheated steam will not do this until it has cooled to the 'correct' temperature so it behaves like hot air and is less efficient than saturated steam. High-temperature, short-time cycles (for example, 134^0^C for 3--5 minutes) may afford the double advantage compared with lower temperature, longer time cycles (for example, 121^0^C for 15 minutes) of causing less degradation of the active ingredient whilst providing a greater level of sterility assurance because of the higher inactivation factors achieved. Despite this, the latter is much more commonly used because it is the reference cycle quoted in the pharmacopoeias. It is essential that air is removed from the autoclave chamber and completely replaced by steam during the operating cycle. Failure to remove all the air results in the temperature being lower than that for pure steam at the same pressure (lower than it should be), so there would be a risk of sterilization failure. The correspondence between temperature and pressure in the autoclave chamber is a good indication of steam quality. The relevant SI unit of pressure is the kilopascal (kPa), although even modern autoclaves do not necessarily use this scale. Instead, the gauge might display pressure in the units of pounds per square inch (psi) or bar (a unit of pressure equal to100 kPa). Pure steam at 15 psi (103 kPa) has a temperature of 121^0^C, and any residual air in the chamber will result in a temperature lower than this. Data showing correspondence of temperature and pressure in accordance with published steam tables contributes to the assurance of sterility because it demonstrates that the process was operating correctly. Larger autoclaves may have vacuum pumps to remove the air, but many smaller ones allow the air to escape through a vent valve. Steam is lighter than air so it rises to the top of the autoclave chamber, and as it accumulates the air is gradually pushed down and out through the valve; autoclaves that remove air in this way are referred to as gravity-displacement or downward-displacement autoclaves. It is essential that the item to be sterilized is fully hydrated, because steam kills microorganisms by hydrolysing vital macromolecules; if there is insufficient water available the hydrolysis is less efficient. Consequently, vacuum-assisted autoclaves are required for the removal of air from dressings or from surgical and laboratory equipment in order for steam to penetrate to all parts of the load. On the same logic, autoclaves should not be used to sterilize the inside of sealed, empty, glass bottles because the organisms inside are simply being exposed to hot air, not to steam. A further benefit of a vacuum pump is that it can be used to dry the load at the end of the cycle -- particularly useful in the case of dressings. The validation of a new autoclave or a sterilization process for a new product is, itself, a subject which has been extensively discussed, so there is no scope here to provide all the details but the following points are fundamental: - Validation of a new autoclave begins at the planning stage when the machine's specification is being considered and continues throughout its installation, commissioning and subsequent testing. - Physical monitoring of the machine's performance in terms of pressure records and temperature data from multiple thermocouples would be required both for the autoclave operating empty and fully loaded with the materials it would be used to process. - The load configuration is important because that may have a bearing on the steam penetration, so any change to the configuration would necessitate revalidation. - Biological indicators are also used for validation to confirm that the autoclave is capable of achieving sterility in the worst case scenario -- one where the load is contaminated with high numbers of the most heat-resistant organisms; they would be placed free in the chamber and within the load itself. - Revalidation is normally undertaken at regular intervals to confirm the continued safe operation of the autoclave, but any change to the product formulation, container or packaging or the operating conditions of the autoclave would necessitate earlier revalidation. Dry heat sterilization This method simply involves heating the item to be sterilized in a hot air oven, typically for 2 hours at 160^0^C, but again, alternative combinations are available, for example 1 hour at 170^0^C or 30 minutes at 180^0^C. The temperatures and times required are longer than those for steam for two reasons: i. because dry heat kills microorganisms by oxidative processes which are less efficient than the hydrolytic mechanisms of steam and ii. because dry air does not possess latent heat. Items that can be successfully sterilized by dry heat are, obviously, those that do not contain water, such as metal instruments, glassware, oils, fats and waxes or any heat-stable non aqueous product like oily injections, ointments or powders. The method is also used for the depyrogenation of glassware required for the preparation or packaging of injections, but bacterial endotoxins (pyrogens) are very heat stable, so temperatures well in excess of 200^0^C are necessary to achieve convenient exposure times; 250^0^C for 30 minutes is common, though higher temperatures are used with shorter times. Ovens used for laboratory-scale dry heat sterilization are superficially similar to fan assisted domestic kitchen ovens though usually with 2--3 times the capacity, but they differ by having temperature recorders, thermocouple inlets, thermal locking doors with non interruptible operating cycles and the facility to filter the air that is drawn into the oven as it cools. When dry heat is used on an industrial scale, sterilizing tunnels employing infrared irradiation or forced convection are more common. These operate continuously, and conveyor belts pass the items to be sterilized through a zone of hot air at 250--300^0^C, with exposure periods of just a few minutes. Items to be sterilized by dry heat need to be appropriately wrapped or sealed in containers that prevent recontamination after processing, and thermocouples are normally incorporated into the load in locations that would be expected to be slowest in achieving the desired operating temperature. Because both heat-up and cool-down times can be several hours, the full cycle time from loading to emptying an oven can be a working day or more despite the fact that the 'holding' time may be just 2 hours or less. Radiation sterilization Gamma-rays or high-energy electrons are used to sterilize heat-sensitive materials and products like medical devices (for example, prostheses, intravenous giving sets, syringes and sutures), surgical instruments, anhydrous medicines (such as ointments) and powders. Gamma radiation is rarely used for water-containing products because the products of radiolysis of water usually cause too much damage to the drug and, even in the case of medical devices, damage to plastics in terms of discolouration and brittleness is a potential problem. Ionization of atoms or molecules by gamma rays and high-energy electrons occurs without inducing radiation in the exposed material. It should be distinguished from non ionizing radiation, for example ultraviolet (UV) light, which possesses sufficient energy merely to produce molecular excitation, although this, in itself, may be sufficient to cause cell damage and death so UV light is used for surface disinfection. The unit for absorbed radiation dose is the gray (Gy) which has replaced the older unit of the rad (which equalled 0.01 Gy). In the United Kingdom, the standard radiation dose recommended for sterilization of pharmaceutical products is 25 kGy (2.5 Mrad in older textbooks) and it is worth noting that, as the complexity of living organisms increases, their susceptibility to ionizing radiation is increased, so the dose necessary to kill microorganisms by radiation sterilization, 25 kGy, is approximately 2500 times the lethal dose for a human (10 Gy or less). The mechanism by which radiation kills cells is that of ionization causing free radical production and damage to the DNA, although both human and microbial cells have damage-repair mechanisms. Gamma rays, which are the more commonly used means of radiation sterilization, are produced from fuel rods normally containing 60cobalt pellets. This isotope has a half-life of 5.25 years, so the rods have to be regularly replaced on a rotational basis to maintain the source strength. In contrast, high-energy electrons are generated electrically so no radioactive source is required. In both cases the items to be irradiated are passed by the radiation source on a conveyor belt or monorail in such a way that all sides of the object are irradiated. Both gamma rays and electrons are more effective when oxygen is present and their activity increases with an increase in temperature although they do not, themselves, normally induce any significant temperature rise in the product being irradiated. Radiation sterilization facilities are expensive to construct and to operate, so companies manufacturing products to be sterilized in this way normally send them to one of a small number of specialist contractors. Health and safety considerations are paramount at radiation processing sites so thorough staff training and monitoring of radiation doses received by personnel are essential. Ultraviolet light is a non ionizing form of electromagnetic radiation that has even poorer penetrating power than accelerated electrons. Light of a wavelength of 260nm is the most effective because that is near to the absorption maxima for nucleic acids. It is commonly used for the disinfection of surfaces in aseptic work areas, air (as in microbiological safety cabinets and operating theatres for example) and for decontamination of water to be used both as an ingredient of medicines and for cleaning purposes. However, endotoxins, as well as other components or products of microbial cells like antigens and enzymes, are likely to remain even after the cell has been killed, so UV treatment could not be used to produce endotoxin-free water for injection. Gaseous sterilization Several microbicidal gases have been used for sterilization including ethylene oxide, formaldehyde, hydrogen peroxide and peracetic acid but, of these, ethylene oxide (sometimes referred to as EtOx) is by far the most common and will be the only one considered here. It is not a favoured method because it is less reliable than heat and radiation, so it needs rigorous in process monitoring to confirm that sterilizing conditions have been achieved; it is also slow and there are several safety issues concerning its use, so it is only employed when there is no alternative. Its use is rather more common in the United States than in the United Kingdom and Europe. Ethylene oxide is suitable for sterilizing materials that are both heat and radiation sensitive, so it is used primarily for disposable medical devices. It is also infrequently used in hospitals for surgical instruments and the sterilization of isolators and chambers, although hydrogen peroxide is now preferred. Ethylene oxide diffuses easily into paper, rubber and many plastics, but it cannot easily penetrate into crystalline materials and its activity is significantly reduced by the presence of organic material (blood, pus or faeces) so it cannot be used to sterilize crystalline raw materials or unwashed surgical instruments. Hospital ethylene oxide sterilizers are similar to conventional autoclaves, being steel chambers of varying capacities from about 65 litres upwards, whilst industrial scale sterilizers are very much larger. In contrast to heat and radiation methods, there is no single widely adopted set of sterilizing conditions for ethylene oxide. Its activity increases with temperature, humidity and gas concentration, so these parameters may be varied generally within the ranges with exposure periods varying from 1 to 10 hours although 3 to 4 hours is typical. Because materials absorb the gas so readily it is common for the concentration to fall during the cycle, so additional gas, and, possibly, steam, may need to be introduced into the sterilizing chamber during the cycle. It is normal for the materials being sterilized to be prehumidified before the gas exposure begins. Ethylene oxide is a colourless gas that is explosive when mixed with air in proportions greater than 3.6% by volume, so it is normally used as a mixture with carbon dioxide (8.5--80% of ethylene oxide), nitrogen or dichlorodifluoromethane (12% ethylene oxide) to minimize the risk. Alternatively it is introduced into an evacuated sterilization chamber as the pure gas at subatmospheric pressure. The sterilization cycle starts with an initial vacuum to remove the air, followed by preliminary heating and humidification with pulsed subatmospheric pressure steam, which would be removed by a second vacuum prior to admission of the ethylene oxide gas into the chamber. After exposure is complete the gas is pumped out and replaced by sterile, filtered air and, usually, the materials are then aired for several hours or even days before use, in order for the absorbed ethylene oxide to dissipate. Ethylene oxide is thought to kill microorganisms by alkylating essential proteins and nucleic acids in the cell; this mechanism of action means that the gas is both mutagenic and carcinogenic. It also causes acute eye, skin and bronchial irritation at concentrations above 200 parts per million (ppm) but, crucially, many people are unable to detect it by smell until the concentration is about three times that value, or more. Health and safety aspects of ethylene oxide sterilization are therefore a major consideration. In addition to the long cycle times and the need for extended airing, the other drawbacks are its inability to inactivate pyrogens and the method does afford the advantages of being well recognized by regulatory authorities and causing only a low requirement for the routine use of biological indicators in every load (not just for initial validation) and all loads being subjected to sterility tests. Filtration sterilization and aseptic manufacture Many drugs and pharmaceutical materials are damaged both by heat and radiation and are not suitable for gaseous sterilization either. Advances in genetic engineering and other aspects of biotechnology have resulted in the introduction of a large number of drugs containing proteins (for example, monoclonal antibodies,interferons) and other biological polymers (such as polysaccharide- or DNA-vaccines), and for many of them the only sterilization option is to use filtration to physically remove the contaminating microorganisms and then manufacture the product aseptically using sterile ingredients. However, filters are available for the removal not only of bacteria and larger organisms like yeasts and mould spores, but viruses too, and they can even remove pyrogens (endotoxins), so filtration is a common means of sterilizing injections and eye drops as well as air and other gases. It should be emphasized, though, that filters designed specifically for endotoxin or virus removal are not routinely used for sterilization purposes. An incidental advantage of filtration sterilization is that pharmacopoeias set limits for the number of particles (of fibres, dust and so forth) that are permissible in injections, and so sterilizing filters, which, of course, remove nonmicrobial particles too, do two jobsin one. In the pharmaceutical industry, the most common materials used for modern sterilization-grade filters are cellulose derivatives and polymers like PTFE, polycarbonate and polyethersulfone. These can all be made as flat sheets, which are often circular so that they fit into purpose-made cylindrical holders, or are pleated to increase their surface area. Commonly they are made into cylindrical cartridges. The advantage of using synthetic polymers is that the pore diameter and density (pores per square millimetre) can be quite precisely controlled; this is important not just from the perspective entrapment of particles within these tortuous channels. The largest common viruses are about 0.3 mm diameter, and go they down in size to less than one-tenth of that value, so it is clear that sieving by a 0.2 mm filter is unlikely to be an effective means of removal of the smallest viruses. Instead, virus-retentive filters are available, which have nominal pore sizes of 0.01--0.02 mm, although pore size is a less reliable guide to the efficiency of viral removal than the log reduction value (LRV), which, as the name suggests, is the logarithm of the ratio of the number of organisms challenging the filter to the number passing through it; LRV values of 3 to 6 are common but there is often a tradeoff between viral reduction and adsorption of the active ingredients of the solution, particularly proteins. An advantage afforded by filtration is that the method physically removes both living and dead cells from solution and in this respect it differs from heat and radiation methods where the dead cells remain in the product and possibly contribute to the pyrogen load. Thus a filter-sterilized solution would be expected to have a greater probability of passing a pyrogen or endotoxin test anyway, but this can be further enhanced by the use of positively charged polyvinylidene fluoride or nylon filters, which attract the negatively charged endotoxins. Filtration is the most convenient method of sterilizing air and other gases and it is used to supply air to pharmaceutical manufacturing suites ('clean rooms') and isolators, operating theatres and microbiological safety cabinets. Depth filters, typically made of glass microfibres separated by aluminium sheets, are normally used for gas filtration and they attract particles of different sizes by the interception, impaction and diffusion mechanisms. High efficiency particulate air (HEPA) filters typically remove 99.97% of airborne particles of 0.3 mm in diameter, although some with higher specifications exist. There is no simple method of monitoring the operation of a filtration sterilizing process as there is with heat and radiation, although a sudden fall in pressure on the upstream side of the filter might indicate a fracture of the filter itself. Representative filters from a manufactured batch are subjected to a microbial challenge test using Brevundimonas (Pseudomonas) diminuta, but this is a destructive test that could not be applied to a filter intended for the manufacture of a medicine; instead, bubble point and diffusive flow tests are performed on filters prior to use. The former, which is less frequently used, measures the pressure required to cause bubbles of gas to emerge through a water-covered filter, whereas the latter determines the pattern of gas diffusion through a filter at progressively increasing pressures. It should be emphasized that filtration is not a terminal sterilization process, defined as one in which the product is sealed then sterilized in its final container. Solutions that are filter sterilized still have to be dispensed into their containers and, despite the operation being undertaken in a class A atmosphere, there is the opportunity for contamination to arise during this process. of removing particles but because it markedly affects the flow rate of the liquid through the filter. Filter units can vary considerably in size from industrial-scale cartridges a metre or more in length to miniaturized disposable syringe filters that can be used for sterilizing small volumes of drug solutions, such as eye drops. A distinction is made between so-called depth filters and membrane filters. The former are more likely to be made from ceramics, glass or metal and have a significant thickness relative to their pore size, whereas a membrane filter, normally of a synthetic polymer, is comparatively thin, although there are no well defined size rules for distinguishing them. These two categories fulfil, to different degrees, the characteristics of an ideal filter: both afford good particle removal (sterilizing efficiency) and mechanical strength and they are easily sterilized in situ by steam, but the membrane filter affords the additional advantages (not possessed by depth filters) of low fluid retention, low solute absorption, no grow-through of microorganisms and no shedding of fibres into the filtrate. Depth filters on the other hand have a high dirt-handling capacity and operate for a long period before clogging; for this reason they are often used as prefilters in front of a membrane filter to extend its life. They cannot easily be compared in terms of speed of filtration because this depends on the characteristics of the filter material itself. Filters are available having a wide variety of pore sizes, and for sterilizing purposes 0.2 mm or 0.22mm diameter pores are recommended but 0.1 mm membranes are becoming more popular. Sieving is one of the principal filtration mechanisms by which microorganisms and other particles are removed from solution and it is considered to be the most reliable. Adsorption of particles onto the surface of the filter or the sides of the pore channels is also a significant mechanism, however, as is the simple entrapment of particles within these tortuous channels. The largest common viruses are about 0.3 mm diameter, and go they down in size to less than one-tenth of that value, so it is clear that sieving by a 0.2 mm filter is unlikely to be an effective means of removal of the smallest viruses. Instead, virus-retentive filters are available, which have nominal pore sizes of 0.01--0.02 mm, although pore size is a less reliable guide to the efficiency of viral removal than the log reduction value (LRV), which, as the name suggests, is the logarithm of the ratio of the number of organisms challenging the filter to the number passing through it; LRV values of 3 to 6 are common but there is often a tradeoff between viral reduction and adsorption of the active ingredients of the solution, particularly proteins. An advantage afforded by filtration is that the method physically removes both living and dead cells from solution and in this respect it differs from heat and radiation methods where the dead cells remain in the product and possibly contribute to the pyrogen load. Thus a filter-sterilized solution would be expected to have a greater probability of passing a pyrogen or endotoxin test anyway, but this can be further enhanced by the use of positively charged polyvinylidene fluoride or nylon filters, which attract the negatively charged endotoxins. Filtration is the most convenient method of sterilizing air and other gases and it is used to supply air to pharmaceutical manufacturing suites ('clean rooms') and isolators, operating theatres and microbiological safety cabinets. Depth filters, typically made of glass microfibres separated by aluminium sheets, are normally used for gas filtration and they attract particles of different sizes by the interception, impaction and diffusion mechanisms shown in Figure 19.8. High efficiency particulate air (HEPA) filters typically remove 99.97% of airborne particles of 0.3 mm in diameter, although some with higher specifications exist. There is no simple method of monitoring the operation of a filtration sterilizing process as there is with heat and radiation, although a sudden fall in pressure on the upstream side of the filter might indicate a fracture of the filter itself. Representative filters from a manufactured batch are subjected to a microbial challenge test using Brevundimonas (Pseudomonas) diminuta, but this is a destructive test that could not be applied to a filter intended for the manufacture of a medicine; instead, bubble point and diffusive flow tests are performed on filters prior to use. The former, which is less frequently used, measures the pressure required to cause bubbles of gas to emerge through a water-covered filter, whereas the latter determines the pattern of gas diffusion through a filter at progressively increasing pressures. It should be emphasized that filtration is not a terminal sterilization process, defined as one in which the product is sealed then sterilized in its final container. Solutions that are filter sterilized still have to be dispensed into their containers and, despite the operation being undertaken in a class A atmosphere, there is the opportunity for contamination to arise during this process. To confirm the suitability of the environment for the purpose, sterile products' manufacturers are required to undertake process simulations (commonly known as 'media fills') in which the same factory filling line that would be used to fill the product in question is first tested by dispensing sterile culture medium into, typically, 5000--10 000 sterile containers. These are sealed and incubated, and should, ideally, yield none showing growth; one positive container would be deemed by the regulatory authorities to be sufficient to justify an investigation and two would require the whole process to be repeated. Because filtration is inherently less reliable than terminal sterilization processes, products sterilized in this way must be subjected to a test for sterility. Biological indicators For steam sterilization Geobacillus stearothermophilus spores are invariably used because of their extreme resistance, ease of cultivation and non pathogenicity (they are thermophiles so they will not grow at body temperature). The spores are dried onto paper strips or other carriers made of glass, plastic or metal, or they are used as aqueous suspensions in sealed ampoules. After exposure during the autoclave cycle under test, the indicators are incubated in culture medium according to the manufacturer's instructions and absence of bacterial growth is taken to mean the spores have been killed and the sterilization cycle was satisfactory. Incubation typically lasts for one week so confirmation of the safety of the cycle cannot be given before then, and this time delay is an inconvenience. Consequently, there are a number of chemical indicators on the market which are intended to closely mimic the performance of the biological indicator; these usually operate on the basis of a colour change occurring after exposure to sterilizing conditions. They are useful for routine day-to-day monitoring in terms of supplementing temperature and pressure data but their use is not permitted by the regulatory authorities as substitutes for biological indicators during autoclave validation.