Personalized Medicine for ASD PDF
Document Details
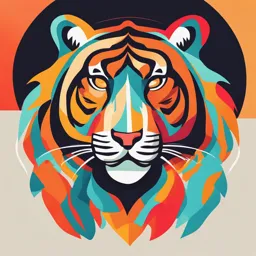
Uploaded by ShinyTropicalIsland
Tags
Related
- Lecture Set 17 - Autism Spectrum Disorder (ASD) DSM-5 PDF
- Autism Spectrum Disorder PDF
- Autism Spectrum Disorder PDF
- Autism Spectrum Disorder Prevalence PDF
- Autism Spectrum Disorder (ASD) Prevalence by Gender, Ethnicity, and Socioeconomic Status
- Autism Spectrum Disorder, Visual and Hearing Impairments PDF
Summary
This document presents a comprehensive overview of personalized medicine approaches to autism spectrum disorders (ASD). It details various aspects of ASD, including its learning outcomes, causes, treatment approaches, and precision medicine considerations.
Full Transcript
Personalized Medicine Approaches to Autism Spectrum Disorders Expected Learning Outcomes : Understanding Autism spectrum of disorders Identify the causes and current treatment approaches Understand the concepts and challenges in the Precision medicine approaches to ASD Overview of Neurodevelopmental...
Personalized Medicine Approaches to Autism Spectrum Disorders Expected Learning Outcomes : Understanding Autism spectrum of disorders Identify the causes and current treatment approaches Understand the concepts and challenges in the Precision medicine approaches to ASD Overview of Neurodevelopmental Disorders Developmental disorders First diagnosed in infancy, childhood, adolescence Attention deficit hyperactivity disorder (ADHD) Specific learning disorders Autism spectrum disorders Intellectual Disability Attention Deficit/Hyperactivity Disorder (ADHD) Central features Inattentive Hyperactive Impulsivity DSM-5 (Diagnostic and Statistical Manual of Mental Disorders ) differentiates two categories of symptoms Problems of inattention Problems of Hyperactivity and impulsivity Attention Deficit/Hyperactivity Disorder (ADHD) Impairments: Behavioral Cognitive Social Academic Adults with ADHD: Lower-level jobs 2.5 fewer years of education More likely to be divorced, have substance use problems and anti-social personality disorder High risk behaviors High comorbidity ODD ( Oppositional defiant disorder) Mood disorders Causes of ADHD Genetics Familial component Copy number variants—CNVs/ Polymorphisms Dopamine related genes - DRD4, DAT1, DRD5 Norepinephrine GABA Serotonin Neurobiological contributions -Smaller brain volume 3-4% Maternal smoking Increases risk Interacts with genetic predisposition Psychosocial and social factors Negative responses Teachers Adults Peer rejection Low self-esteem Poor self-image Treatment of ADHD Psychosocial intervention Improving academic performance, decreasing disruptive behavior, and improving social skills Behavioral interventions before medication Parent training Social skills training Biological intervention Biological Reduce impulsivity and hyperactivity Improve attention Effects of medications Improve compliance Decrease negative behaviors Do not affect learning and academic performance Possible abuse issues Side effects Autism spectrum disorder (ASD) is a neurodevelopmental disorder Two major characteristics: Communication and social interaction Restricted, repetitive patterns of behavior, interests, or activities Three levels of severity Level 1— “Requiring support” Level 2— “Requiring substantial support” Level 3— “Requiring very significant substantial support” Impairment in social communication and social interaction Fail to develop age-appropriate social relationships Deficits in nonverbal communication Restricted, repetitive patterns of behavior, interests, or activities Maintenance of sameness Stereotyped and ritualistic behaviors Causes of Autism Spectrum Disorders Psychological and Social Dimensions Failed parenting Perfectionistic, cold, and aloof High socioeconomic status Higher IQs Lack of self-awareness Limited self-concept Behavioral, Self-injury Biological Significant genetic component Familial component 20% risk of second child with autism Bonding and social memory Older parents increased risk Neurobiological influences: Amygdala ( integrative center of emotions in the brain) Larger size at birth = higher anxiety, fear Elevated cortisol Neuronal damage Fewer neurons Oxytocin Lower levels Treatment of Autism Spectrum Disorders Biological treatments: Psychosocial treatments: Medical intervention has little positive impact Decrease agitation- Tranquilizers Integrated treatments: Preferred model Multidimensional, comprehensive focus: Children, Behavioral approaches Skill building Reduce problem behaviors Communication and language training Increase socialization Naturalistic teaching strategies Early intervention is critical Families, Schools, Home Community and social support Prevention of Neurodevelopmental Disorders: Early intervention At-risk children, families Head Start Program Educational Medical Social supports Genetic screening Detection and correction Prenatal gene therapy Precision medicine approaches to ASD – Challenges Autism spectrum disorder (ASD) is a multifactorial neurodevelopmental disorder characterized by impairments in two main areas: social/communication skills and repetitive behavioral patterns. Due to the complexity and heterogeneity of ASD, symptoms vary in severity and may be accompanied by comorbidities such as epilepsy, attention deficit hyperactivity disorder (ADHD), and gastrointestinal (GI) disorders. Identifying biomarkers of ASD is not only crucial to understanding the biological characteristics of the disorder, but also as a detection tool for its early screening. In most cases, ASD is diagnosed at school age , with a mean age of 6 years old , although it should be diagnosed earlier. Given the lack of effective drugs to alleviate or reduce the core symptoms of ASD, early behavioral interventions are key for better outcomes, as it improves cognitive performance as well as behavioral and language skills. Identifying biomarkers of ASD is not only crucial towards understanding the biological characteristics of the disorder, but also as a detection tool for its early screening. It supports the currently available diagnostic methods and to establish a platform for a more robust and objective methodology. Common clinical profiles associated with ADHD Assessment and management of neurodevelopmental problems—the potential for fragmentation of services Precision Medicine approaches to ASD – Biomarkers To date, no effective medical treatments are available that significantly improve the core symptoms of ASD. Recognition of the phenotypic and etiological variability between individuals in the autism spectrum and the lack of effective treatments necessitates a precision medicine approach. This approach aims to identify targeted treatments based on the understanding of the underlying pathophysiology ; combine the drug (or intervention) with a companion diagnostic (stratification biomarker) to select or exclude patients for a particular treatment. Stratification biomarkers divide a group of patients into subgroups with shared biological characteristics. These subgroups may differ in terms of their clinical symptom profile and/or etiology. Stratification biomarkers may be primarily clinically relevant if they have either prognostic value, i.e., they assess the (untreated) progression and outcome of the disorder, or predictive value, i.e., they estimate the probability of response to a given treatment. Stratification biomarker research for ASD is still in the early stages. Interplay between the main determinants of Autism Spectrum Disorder MD, Mitochondrial dysfunction; MIA, Maternal Immune Activation; ROS, Reactive Oxygen Species. In the newborn, matrilinear transfer of mitochondria and microbiota adds to the genetic information stored in the maternal and paternal germinal cells. Environmental factors may directly affect the epigenetic machinery or may influence the interconnected molecular pathways involved in the ‘bad trio’ (mitochondrial dysfunction [MD)/oxidative stress (ROS) / maternal immune activation (MIA) /dysbiosis]. The placenta drives the metabolic and epigenetic regulation of fetal programming, hence influencing the crucial early stages of neurodevelopment. The epigenome is programmed in an adaptive and predictive sense by the intrauterine and cellular microenvironment, setting the limits of physiological adaptations to the postnatal environment and influencing the lifelong risk for diseases. After birth, the same mechanisms involving environmental factors and the ‘bad trio’ are at play and may continue to undermine human health lifelong. As for neurodevelopment, the maximum impact occurs in the first two years of life, which is the crucial time window for brain wiring. Common Variants and Genomic Background The impact of rare mutations on the phenotypic outcome may depend on genomic background (the sum of common variants). It may act as a “buffer” that diminishes or increases the deleterious effect of rare variants (CNVs) – maybe by modulating synaptic homeostasis. For an individual with a genetic background that contains a high load of common ASD risk variants, a small burden of rare risk genes may suffice to cause ASD. An individual whose genetic background only includes a small number of common risk variants may require a higher burden of deleterious mutations to cause ASD. Environmental Risk Factors In addition to genetic factors, it is likely that environmental influences during the embryonic stage – modulate risk for ASD. This includes maternal infections and prenatal exposure to toxins, and dysfunction of the immune system. Human studies comparing monozygotic and dizygotic twins have consistently revealed significant environmental influences that account for approximately 5–6% of the observed variance in ASD. Critical Periods and the associated challenges The time when different factors impact on neuronal development plays a crucial factor in determining developmental outcome. Brain development is initially determined by distinct temporal and spatial stages of gene expression and intrinsic neuronal activity then becomes actively refined by interactions with the environment. Timing could be influenced either by genetic factors as different genes are expressed at different times in brain development [in humans, genes involved in cell proliferation are expressed earlier than those involved in synaptogenesis or myelination] or the time when an environmental insult occurs. By altering the developmental window during which genetic/environmental insults are applied, animal studies could trace these effects across cellular, molecular, brain systems, and behavioral levels. However, most animal studies have been tested only in adult animals. This indicates only the end state – and not how abnormalities developed or changed across development. It is possible that some treatment effects may be different in developing vs. adult brains. A treatment that is likely only effective in early development would raise important ethical implications for clinical trial designs that usually first test safety, efficacy and side-effects in adults. Interplay between four factors that may impact on developmental outcome Rare and common genetic variants, as well as environmental risk factors (e.g., toxins, maternal infection) acting at different time points impact on the developmental outcome. The same rare genetic mutation can either lead to ASD, ASD + ADHD, or ADHD, depending on the person’s genomic background (the sum of common variants). Or the effect of a genetic variant could be modulated by environmental insults that occur during the first, second or third trimester of the pregnancy. Many ASD risk genes are involved in essential developmental processes including proliferation, neuronal growth and differentiation, and synapse formation. These genes are expressed at different times in brain development. ASD manifestations have been linked to a variety of well-known single-gene (monogenic) conditions. Common examples are fragile X syndrome (FXS) caused by a mutation in the FMR1 gene Tuberous sclerosis complex (TSC) caused by a mutation in TSC1 and TSC2 genes. ASD can be caused by single nucleotide variants (SNV), structural variants (SV), or copy number variants (CNV) that may affect multiple genes. Large SVs are linked to ASD along with other co-morbidities such as epilepsy, hyperactivity, behavioral problems, schizophrenia, and dysmorphic features. SVs are detected using cytogenetic and comprehensive genomic hybridization (CGH) techniques in addition to next-generation sequencing (NGS). A genome-wide CNV study done on ASD patients and healthy controls showed that SVs tend to encompass NLGN1, ASTN2, UBE3A, PARK2, RFWD2, and FBXO40 in ASD patients. These genes are known to be involved in cell-adhesion and ubiquitin pathways, as these pathways are important for synaptic formation, neuronal connection, and proper neuronal cell functions. Non-Coding RNA as Biomarkers for ASD MicroRNAs (miRNAs) are a group of non-coding RNAs (ncRNA) family. Other forms of ncRNA include long non-coding RNAs (lncRNA), small nuclear RNA (snRNA), small nucleolar RNA (snoRNA), ribosomal RNA (rRNA), and pseudogenes. The main function of miRNA is to regulate gene expression at the posttranscriptional level. Using a miRNA PCR array specific for neurological miRNAs, five potential miRNAs that could differentiate healthy subjects from ASD subjects were identified in the serum of ASD patients and matched controls, these miRNAs are miR-19b-3p, miR130a-3p, miR-181b-5p, miR-320a, and miR-572. miR-140-3p was found to be upregulated in both serum and saliva detected by RNA-Seq in saliva and TaqMan low-density array (TLDA) in serum, suggesting that miR-140-3p might have a particular role in ASD. It was observed that miR-140-3p and other miRNAs’ expression were dysregulated in ASD cerebral cortex, examined using multiplex reverse transcriptase-polymerase chain reaction (RT-PCR) on ASD postpartum samples Some of these ncRNA, such as POLR2KP2, TUBB2BP1, RNU1-16P, and RNVU1-15 are moderately to highly expressed in the neurons, which may suggest a possible association of these ncRNA to ASD pathogenesis in the brain. Proteins are the final products that carry function. Protein abnormalities reflect upstream molecular problems that occur at the DNA and RNA levels and these problems can be mirrored by a change in protein activity, structure, and abundance. Applying the MS-based proteomics approach for biomarker discovery has opened the door for neurodevelopmental and neuropsychiatric disorders providing an unbiased method for a better understanding of these complex conditions. Other methods of proteomic biomarker investigation are immunoassays. These methods require prior knowledge of the protein’s function and their expression in a particular tissue/body fluid. This allows for the generation of immunoassays to capture proteins and evaluate possible upregulation / downregulation as well as post translational modifications on a set of proteins. These techniques are used mostly in the validation phase after biomarker discovery phase, and they are easy to implement in a clinical setting. Proteomic investigations on the gray matter of the frontal lobe of ASD individuals identified single amino acid substitutions from alanine to glutamic acid in a protein called Glyoxalase I (Glo1). This noticeable change has a higher frequency in ASD postmortem brain tissue compared to healthy controls. Therefore, Glo1 substitution may affect certain crucial functions during neural development in early life. Synucleins are a family of proteins that are abundantly expressed in the presynaptic terminals of the neocortex, cerebellum, thalamus, and striatum. The synuclein family includes α, β, and γ-synucleins (syn). α-syn is thought to be involved in vesicle stabilization, synaptic plasticity, and regulates dopamine release. α-syn is known to be involved in Parkinson’s disease pathology through an intracellular aggregation process that results in Lewy body formation inside the neurons and eventually, cell death. A consistent decrease in α-syn concentration is observed in serum and plasma of ASD patients. β-syn was shown to be higher in ASD patient’s plasma compared to the age-matched controls. Autoantibodies against α-syn and other brain proteins were shown to increase in serum of ASD children and their corresponding mothers. Those autoantibodies may mask α-syn epitopes, which could result in α-syn being under-detected. Tau is a major microtubule-associated protein in mature neurons and was known to be hyperphosphorylated in Alzheimer’s disease patients. This hyperphosphorylation causes neurofibrillary tangles and neuronal death. Tau concentration decreases in the serum of ASD males. More studies need to be done to elucidate the role of synucleins and Tau in ASD. ASD risk is traced to around 1000 genetic factors and many environmental factors, but it may be impractical to assume that every individual proteins could be used as a universal biomarker for ASD. Thus, utilizing unbiased methods for proteomics profiling could be more beneficial for patient stratification and biomarker discovery. Metabolic abnormalities are known to be multidimensional as they cross many pathways such as mitochondrial, oxidative, cholesterol, fatty acid, and neurotransmitters metabolism. Studies suggest a level of dysfunction in these metabolic pathways in ASD patients. Gamma-aminobutyric acid (GABA) and glutamate are important neurotransmitters in the brain. A notable disturbance of the glutamatergic and GABAergic balance in individuals with ASD in comparison to controls has been identified, with a decreased glutamate to GABA ratio, making it a potential area of biomarker exploration for ASD. Short-chain fatty acids (SCFA), produced by intestinal microbiota, was reported to be lower in ASD fecal samples. SCFA act as histone deacetylase inhibitor (HDAC), which is important for glial cell function, and regulate tryptophan 5hydroxylase 1, which is important for serotonin and tyrosine hydroxylase, a rate-limiting enzyme for the synthesis of dopamine, noradrenaline, and adrenaline. Gut microbiota is known to impact many neurological processes such as blood-brain barrier formation, myelination, and synthesis of neurotransmitters including GABA, dopamine, and others. Those processes are mediated through the microbiota-gut-brain axis, which is a path of bidirectional communication between the CNS and the gut. The link between the gut microbiome and ASD was made because GI disturbances have been frequently detected in ASD. The autonomic nervous system that controls gut function is called the enteric nervous system (ENS) and this system shares many structural and functional characteristics with the CNS. Individuals with ASD that exhibit mutations in the CHD8 (chromodomain helicase DNA binding protein 8) gene were reported to have constipation. Germ-free mice that received microbiota transfer from ASD donors showed autistic-like behaviors compared to mice that received a transfer from typically-developed donors. Those mice displayed differences in their metabolome and microbiome profiles evaluated using metagenomic analysis. The evidence that links ASD core symptoms to GI disturbances is strong and is currently being explored in clinical trials. Mitochondrial dysfunction is linked to ASD A systematic meta-analysis study showed that the prevalence of mitochondrial diseases in ASD was 4–5%, which is markedly higher than the general population (around 0.01%). Lactate was the first biomarker that was found to be elevated in ASD children’s serum. Other mitochondrial biomarkers that were shown to be elevated in children with ASD are AST, pyruvate, and creatine kinase. On the other hand, carnitine was shown to decline. Mitochondrial abnormalities such as increased hydrogen peroxide, reduced NADH, as well as mitochondrial DNA overreplication, were observed in lymphocytes isolated from ASD subject. Using an MS approach for mitochondria biomarker discovery, one study constructed a signature metabolomic pattern that is highly sensitive and specific in predicting ASD patients using plasma samples. Most of these signature molecules identified have been previously reported such as creatinine, fatty acids, 3aminoisobutyric acid, tricarboxylic acid, and BCAAs (Branch chained amino acid) More evidence is pointing towards an association between neurodevelopmental regression (NDR) with mitochondrial dysfunction. A recent study has shown that the mitochondrial respiratory rate is elevated in ASD with NDR compared to ASD with no NDR, suggesting a potential subtype of ASD. Altered immune response is also evident in ASD. Cytokines such as TNF-α is significantly expressed in the brain, CSF, and peripheral blood mononuclear cells (PBMCs) of individuals with ASD. This increase in TNF-α may suggest a dysregulation in the inflammatory response in ASD. An increased NF-kB binding activity to DNA is observed in the PBMCs of ASD patients. TNF-α is mainly produced by M1 macrophages ; it is important for NF-kB activation, a transcription factor and an essential regulator of inflammatory genes. Another essential cytokine is interferon-gamma (IFN-γ), which was found to be elevated in the brain and whole blood of ASD compared to controls. Mothers of individuals who were later diagnosed with autism showed an elevated level of serum IFN-γ, as well as interleukins (IL-4 and IL-5) during mid-gestation. IFN-γ is a pro-inflammatory cytokine that activates CD4+ T-helper 1 (Th1) response. Another pro-inflammatory cytokine interleukin-6 (IL-6) showed a significant production in monocytes of ASD compared to controls when their PBMCs were stimulated with lipopolysaccharide (LPS) in vitro. IL-6 is known to induce T-helper 17 (Th17). These findings highlight the importance of cytokines in discriminating ASD from controls and the possible link between immune dysfunction and ASD subtype. Schematic showing possible body fluids and tissues that may be essential for biomarker discovery in ASD patients. Blood is the most common site for biomarker discovery. However, saliva, urine, and feces are easily accessible and have been used recently for biomarker discovery studies. CSF and brain tissues can also be used for biomarker discovery in ASD, their accessibility is very difficult or even impossible. Collecting huge biological data from a range of body fluids may help in performing holistic molecular profiling in the area of genomics, transcriptomics, proteomics, epigenetics, metabolomics, gut microbiome, and immune system, which may enhance biomarker discovery and patient stratification. The goal of biomarker discovery is implementing biomarkers within clinical settings to provide ASD risk assessment, screening, diagnosis, monitoring, and stratifications for better therapeutic strategies. Biomarkers for early diagnosis and stratification are needed for ASD, especially for early diagnosis, hence, early intervention, an essential key for better outcomes. The extreme heterogeneity and complexity of ASD in terms of clinical manifestations, genetic background, and biological changes makes it hard for ASD to fit into a one size fits all treatment and diagnostic approach model; applying a multi-modal approach utilizing modern technologies is a key for proper stratification and achieving tailored therapy that is most fitted to an individual’s condition. Any Questions?