Plant Physiology PDF
Document Details
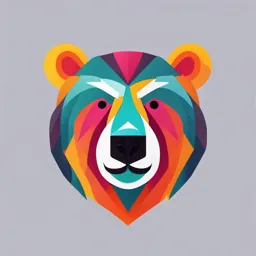
Uploaded by FoolproofSard7720
Tags
Summary
This document provides an overview of plant structure, focusing on water absorption and transport across plant tissues. It details the different pathways of water movement from the soil to leaves, including apoplast, symplast, and transmembrane pathways. In addition, the mechanism of water transport in the xylem and root pressure is also explained.
Full Transcript
OVERVIEW OF PLANT STRUCTURE WATER IN THE SOIL The water content and the rate of water movement in soils depend to a large extent on soil type and soil structure When a soil is heavily watered by rain or by irrigation, the water percolates downward by gravit...
OVERVIEW OF PLANT STRUCTURE WATER IN THE SOIL The water content and the rate of water movement in soils depend to a large extent on soil type and soil structure When a soil is heavily watered by rain or by irrigation, the water percolates downward by gravity through the spaces between soil particles, partly displacing, and in some cases trapping, air in these channels. Water in the soil may exist as a film adhering to the surface of soil particles, or it may fill the entire channel between particles. Field capacity: is the water content of a soil after it has been saturated with water and excess water has been allowed to drain away. WATER ABSORPTION BY ROOTS WATER ABSORPTION BY ROOTS Intimate contact between the surface of the root and the soil is essential for effective water absorption by the root. This contact provides the surface area needed for water uptake and is maximized by the growth of the root and of root hairs into the soil. Root hairs are microscopic extensions of root epidermal cells that greatly increase the surface area of the root, thus providing greater capacity for absorption of ions and water from the soil In the soil, water is transported predominantly by bulk flow. However, when water comes in contact with the root surface, the nature of water transport becomes more complex. From the epidermis to the endodermis of the root, there are three pathways: Apoplast pathway Transmembrane pathway Symplast pathway In the apoplast pathway, water moves exclusively through the cell wall without crossing any membranes. The apoplast is the continuous system of cell walls and intercellular air spaces in plant tissues. The transmembrane pathway is the route followed by water that sequentially enters a cell on one side, exits the cell on the other side, enters the next in the series, and so on. In this pathway, water crosses at least two membranes for each cell in its path (the plasma membrane on entering and on exiting). Transport across the tonoplast may also be involved. In the symplast pathway, water travels from one cell to the next via the plasmodesmata. The symplast consists of the entire network of cell cytoplasm interconnected by plasmodesmata. At the endodermis, water movement through the apoplast pathway is obstructed by the Casparian strip. The Casparian strip is a band of radial cell walls in the endodermis that is impregnated with the wax like, hydrophobic substance suberin. Casparian strip breaks the continuity of the apoplast pathway, and forces water and solutes to cross the endodermis by passing through the plasma membrane. Thus, despite the importance of the apoplast pathway in the root cortex and the stele, water movement across the endodermis occurs through the symplast. Roots generate positive hydrostatic pressure by absorbing ions from the dilute soil solution and transporting them into the xylem. The buildup of solutes in the xylem sap leads to a decrease in the xylem osmotic potential (Ys) and thus a decrease in the xylem water potential (Yw). This lowering of the xylem Yw provides a driving force for water absorption, which in turn leads to a positive hydrostatic pressure in the xylem. In effect, the whole root acts like an osmotic cell; the multicellular root tissue behaves as an osmotic membrane does, building up a positive hydrostatic pressure in the xylem in response to the accumulation of solutes. Root pressure is most likely to occur when soil water potentials are high and transpiration rates are low. When transpiration rates are high, water is taken up so rapidly into the leaves and lost to the atmosphere that a positive pressure never develops in the xylem. Plants that develop root pressure frequently produce liquid droplets on the edges of their leaves, a phenomenon known as Guttation. Guttation: causes exudation of xylem sap through specialized pores called hydathodes that are associated with vein endings at the leaf margin. The “dewdrops” that can be seen on the tips of grass leaves in the morning are actually guttation droplets exuded from such specialized pores. Guttation is most noticeable when transpiration is suppressed and the relative humidity is high, such as during the night. WATER TRANSPORT THROUGH THE XYLEM In most plants, the xylem constitutes the longest part of the pathway of water transport. In a plant 1 m tall, more than 99.5% of the water transport pathway through the plant is within the xylem, and in tall trees the xylem represents an even greater fraction of the pathway. Compared with the complex pathway across the root tissue, the xylem is a simple pathway of low resistance. Tracheary elements in the xylem: Tracheids and Vessel elements. The maturation of both tracheids and vessel elements involves the “death” of the cell. Thus, functional water-conducting cells have no membranes and no organelles. What re-mains are the thick, lignified cell walls, which form hollow tubes through which water can flow with relatively little resistance. XYLEM STRUCTURE Tracheids are elongated, spindle-shaped cells that are arranged in overlapping vertical files. Water flows between tracheids by means of the numerous pits in their lateral walls. Pits of one tracheid are typically located opposite pits of an adjoining tracheid, forming pit pairs. Vessel elements tend to be shorter and wider than tracheid and have perforated end walls that form a perforation plate at each end of the cell. Pit membranes in tracheids of some Like tracheids, vessel elements have pits on species of conifers have a central their lateral walls. thickening, called a torus Unlike tracheids, the perforated end walls The torus acts like a valve to close the allow vessel members to be stacked end to end pit by lodging itself in the circular or to form a larger conduit called a vessel. oval wall thickenings bordering these Maximum vessel lengths range from 10 cm to pits. many meters. The Cohesion–Tension Theory Explains Water Transport in the Xylem In theory, the pressure gradients needed to move water through the xylem could result from the generation of positive pressures at the base of the plant or negative pressures at the top of the plant. We mentioned previously that some roots can develop positive hydrostatic pressure in their xylem—the so-called root pressure. However, root pressure is typically less than 0.1 MPa and disappears when the transpiration rate is high, so it is clearly inadequate to move water up a tall tree. Instead, the water at the top of a tree develops a large tension (a negative hydrostatic pressure), and this tension pulls water through the xylem. This mechanism, first proposed toward the end of the nineteenth century, is called the cohesion–tension theory of sap ascent because it requires the cohesive properties of water to sustain large tensions in the xylem water columns. The tensions needed to pull water through the xylem are the result of evaporation of water from leaves. In the intact plant, water is brought to the leaves via the xylem of the leaf vascular bundle, which branches into a very fine and sometimes intricate network of veins throughout the leaf. venation pattern From the xylem, water is drawn into the cells of the leaf and along the cell walls. The negative pressure that causes water to move up through the xylem develops at the surface of the cell walls in the leaf. The situation is analogous to that in the soil. The cell wall acts like a very fine capillary wick soaked with water. Water adheres to the cellulose microfibrils and other hydrophilic components of the wall. The mesophyll cells within the leaf are in direct contact with the atmosphere through an extensive system of intercellular air spaces. Initially water evaporates from a thin film lining these air spaces. As water is lost to the air, the surface of the remaining water is drawn into the interstices of the cell wall, where it forms curved air–water interfaces. Because of the high surface tension of water, the curvature of these interfaces induces a tension, or negative pressure, in the water. As more water is removed from the wall, the radius of curvature of the air–water interfaces decreases and the pressure of the water becomes more negative. Thus the motive force for xylem transport is generated at the air–water interfaces within the leaf.