Physio CNS and Special Senses PDF
Document Details
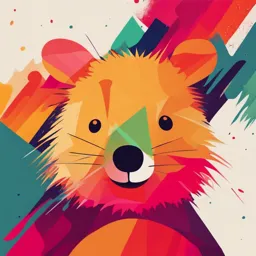
Uploaded by Raelag2502
Uniwersytet Warmińsko-Mazurski w Olsztynie
Tags
Summary
This document contains information about the nervous system, including resting membrane potentials, action potentials, and related concepts. It also includes questions relevant to the topic.
Full Transcript
- Because fat requires more time to become fully saturated with nitrogen – due to poor blood supply ▪ If a person remains at deep levels for few hours – both body water and fat become saturated with nitrogen...
- Because fat requires more time to become fully saturated with nitrogen – due to poor blood supply ▪ If a person remains at deep levels for few hours – both body water and fat become saturated with nitrogen NERVOUS SYSTEM (24) 82. What is a resting membrane potential. Name and describe in detail all the factors contributing to the resting membrane potential in neurons. RESTING POTENTIAL OF NEURON - It is a voltage difference across the plasma membrane of a neuron (a difference between inside and outside of the cell) - It measures about 70 millivolts (with the negative inside of the membrane compared to the outside). - It is called a resting potential because it occurs when a membrane is not being stimulated or conducting impulses. FACTORS CONTRIBUTING TO RESTING POTENTIAL OF NEURON: - Unequal distribution of ions across the plasma membrane, especially Na+ and K+ ▪ Relative permeability of the cell membrane for K+, Cl- and Na+ ions (10:4:1) - Each permeant ion attempts to drive the membrane potential toward its own equilibrium potential. Ions with the highest permeabilities at rest will make the greatest contributions to the resting membrane potential, and those with the lowest permeabilities will make little or no contribution. - The resting membrane potential is close to the equilibrium potentials for K+ and Cl- because the permeability to these ions at rest is high. - At rest the Na+ channels are closed and the Na+ conductance is low. ▪ Active transport of Na+ and K+ ions by sodium-potassium pump - Both sodium and potassium ions are moving from areas of low concentration to areas of high concentration (against the concentration gradient) - This pump is an antiport active transporter. It translocates 3 Na+ ions out of the cell and 2 K+ ions into the cell. - Na+- K+ ATPase is necessary to create and maintain the K+ concentration gradient, which establishes the resting membrane potential. - Impermeability of the cell membrane for the organic ions ▪ Often, organic anions are negatively charged amino acid side chains in proteins. The proteins are typically large and bulky and remain trapped inside the cell. Thus, organic anions generally cannot cross the membrane like Na+ and K+ 83. What is an action potential. Name all the phases of an action potential. Describe step by step activation and inactivation of sodium and potassium voltage gated channels during the phases of action potential in neuron. ACTION POTENTIAL - It is rapid change in the membrane potential that spread along the nerve fiber membrane. ▪ Each action potential begins with a sudden change from the normal resting negative membrane potential to a positive potential and ends with an almost equally rapid change back to the negative potential. - An action potential of neuron is an „ALL-OR-NONE” response. ▪ All action potentials are the same size. Therefore, the neuron either does not reach the threshold or a full action potential is fired ▪ The amplitude of propagating action potential is always the same (about 120 mV) and it does not diminish with distance. - A typical action potential begins at the axon hillock since the axon hillock has the highest concentration of sodium ion channels. - If the depolarizing stimulus causes membrane potential to become more positive than a threshold voltage, the depolarization triggers an action potential. Phases of action potential: - Resting membrane potential: ▪ K+ conductance is high, K+ channels are almost fully open ▪ Cl+ conductance is high as well ▪ Na+ conductance is low because Na+ channels are closed. - Depolarization: ▪ Membrane suddenly becomes permeable to sodium ions, allowing tremendous numbers of positively charged sodium ions to diffuse to the interior of the axon. ▪ Membrane potential rises rapidly in the positive direction - Repolarization: ▪ The sodium channels begin to close and the potassium channels open to a greater degree than normal. ▪ Rapid diffusion of potassium ions to the exterior re-establishes the normal negative resting membrane potential. - Hyperpolarizing afterpotential: ▪ For a brief period following repolarization, the K+ conductance is higher than at rest and the membrane potential is driven even closer to the K+ equilibrium potential. ACTIVATION AND INACTIVATION OF NA+ AND K+ CHANNELS: - Voltage-gated sodium channels has two gates – activation gate and inactivation gate ▪ When the membrane potential becomes less negative than during the resting state (−70 and −50 millivolts) that causes a sudden conformational change in the activation gate, flipping it all the way to the open position. This allows Na+ ions to flow inside the cell. ▪ The same increase in voltage that opens the activation gate also closes the inactivation gate. However, the conformational change that flips the inactivation gate to the closed state is a slower process than the conformational change that opens the activation gate. ▪ The inactivation gate will not reopen until the membrane potential returns to or near the original resting membrane potential level. - Voltage-gated potassium channels have only one gate ▪ During the resting state, the gate of the potassium channel is closed and potassium ions are prevented from passing through this channel to the exterior. ▪ During depolarization (when potential rises from −90 millivolts toward zero) voltage change causes an opening of the gate and allows increased potassium diffusion outward through the channel ▪ Voltage-gated potassium channels are delayed. - Because of the slight delay in opening of the potassium channels, they open at the same time that the sodium channels are beginning to close because of inactivation. 84. Explain in detail an absolute and relative refractory periods in neurons (explain the terms, write how long do they last, explain in detail why do they exist according to activation and inactivation of the voltage gated channels). ABSOLUTE REFRACTORY PERIOD: - It is a time after initiation of an action potential when it is impossible to produce a second action potential, regardless of the stimulus strength or duration. - It occurs because most of the voltage-gated Na+ channels are closed - It lasts during the depolarizing phase and overshoot - In neurons this period lasts for about 1 ms RELATIVE REFRACTORY PERIOD: - During this period a second action potential can be evoked, but the minimal stimulus necessary for activation must be stronger or longer than normal. - It immediately follows absolute refractory period - It appears gradually in the repolarizing phase of an action potential - It corresponds to the period when the voltage-gated K+ channels are open. - It lasts approximately 0.8 ms - The return to the equilibrium resting potential marks the end of the relative refractory period. 85. Describe the mechanisms of initiation and propagation of the action potentials. What is an all-or- nothing principle. ACTION POTENTIAL PROPAGATION: - Action potential elicited at any one point on an excitable membrane usually excites adjacent portions of the membrane, resulting in propagation of the action potential along the membrane. - The action potential travels in all directions away from the stimulus until the entire membrane has become depolarized. - A typical action potential begins at the axon hillock since the axon hillock has the highest concentration of sodium ion channels. - Propagation of the action potential occurs by the spread of local currents from active regions to adjacent inactive regions. They cause the interior of the cell to become more positive due to increased Na+ inflow. ALL-OR-NOTHING PRINCIPLE: - All action potentials are the same size. Therefore, the neuron either does not reach the threshold or a full action potential is fired - The amplitude of propagating action potential is always the same (about 120 mV) and it does not diminish with distance. 86. Explain in detail the differences in structure and function of electrical and chemical synapses. The synapses allow nerve impulses to be transmitted from one neuron to the next one. CHEMICAL SYNAPSE: - Composed of: ▪ Presynaptic bouton (presynaptic terminal or bulb; varicosity), which contains vesicles with neurotransmitters. - Vesicles that store and release small molecule transmitters are continually recycled and used over and over again - One of the most used transmitter is ACH, norepinephrine, epinephrine, histamine, gamma-aminobutyric acid (GABA), glycine, serotonin, and glutamate. ▪ Synaptic cleft (200-300 Ångstrom = 20-30 nm) ▪ Postsynaptic membrane, which contains receptors for neurotransmitters. - Receptor proteins have 2 components: ▪ Binding component that protrudes outward into synaptic cleft and binds to the neurotransmitter ▪ Intracellular component, which faces the interior. - There are 2 types of receptors in the post synaptic terminal: ▪ Ionotropic receptors which gate ion channels ▪ Metabotropic receptors that act through a second messenger. - Mechanism of action: ▪ Action potential depolarizes the presynaptic membrane. ▪ Depolarization opens voltage gated Ca2+ channels, which allows entry of Ca2+ into the cell, where it binds to a specific release site. Calcium entry triggers exocytosis of synaptic vesicle contents to synaptic cleft. ▪ Neurotransmitter diffuse across the synaptic cleft and binds with receptors on the postsynaptic cell - One- way conduction, meaning that the neurotransmitter will always go in one direction: from the presynaptic neuron to the postsynaptic neuron. ▪ It allows signals to be directed to specific neurons, which allows for precise and distinctive CNS functions. - The most numerous synapses in the CNS are chemical synapses. ELECTRICAL SYNAPSE: - The cytoplasm of adjacent cells are connected via gap junctions, allowing free ion movement between cells ▪ Gap junctions are formed exclusively from hexameric pores, called connexons (Cx36) ▪ Each connexon is made from 6 protein subunits of connexin - Intercellular gap (20-40 Å = 2-4 nm) - Bidirectional transmission (impulse transmission in either direction), which allows them to coordinate the activities of large interconnected neurons. - Electrical synapses allow for quick transmission and control of large amounts of neurons ▪ Electrical synapses are often found in neural systems that require the fastest possible response, such as defensive reflexes. 87. Characterize chemical substances that function as synaptic transmitters. There are three groups of synaptic transmitters: small-molecule transmitters, neuropeptides and purines. SMALL-MOLECULE TRANSMITTERS: - They are rapidly acting - They are synthesized in the cytosol of the presynaptic terminal and absorbed by means of active transport into the many transmitter vesicles in the terminal - Each time an action potential reaches the presynaptic terminal, a few vesicles at a time release their transmitter into the synaptic cleft. - Most often the effect is to increase or decrease conductance through ion channels: ▪ Increased sodium conductance causes excitation ▪ Increased potassium or chloride conductance causes inhibition - Examples of small-molecule transmitters include: acetylcholine, norepinephrine, epinephrine, dopamine, serotonin, histamine, gamma-aminobutyric acid, glycine, glutamate, aspartate, nitric oxide Acetylcholine: - Synthesized in the presynaptic terminal from acetyl CoA and choline in the presence of choline acetyltransferase. ▪ When the vesicles release the acetylcholine into the synaptic cleft, the acetylcholine is rapidly split again to acetate and choline by the enzyme cholinesterase, which is present in the proteoglycan reticulum that fills the space of the synaptic cleft. ▪ Choline is actively transported back into the terminal to be used again for synthesis of new acetylcholine. - It is secreted by neurons in many areas of the nervous system, including: preganglionic and postganglionic neurons of ANS, pyramidal cells of motor cortex, neurons in basal ganglia. It is the only neurotransmitter secreted from neuromuscular junctions. ▪ In most instances, acetylcholine has an excitatory effect ▪ However, it is known to have inhibitory effects at some peripheral parasympathetic nerve endings, such as inhibition of the heart by the vagus nerves. Dopamine: - Synthesized from tyrosine - Secreted by neurons that originate in the substantia nigra - The effect of dopamine is usually inhibition - Dopaminergic neurons secrete dopamine due to the presence of Tyrosine Hydroxylase. Norepinephrine and epinephrine: - Synthesized from tyrosine. Dopamine is converted to norepinephrine. Norepinephrine is methylated to form epinephrine. - Secreted by the terminals of many neurons whose cell bodies are located in the brain stem and hypothalamus. - Both excitatory and inhibitory, depending on the organ that they innervate Serotonin: - It’s produced from tryptophan in serotogenic neurons in the brain and GI tract. - It serves as a precursor to melatonin in the pineal gland. - It acts as a inhibitor of pain pathways. It helps control the mood of a person or cause sleep. Histamine : - Synthesized from histidine by histidine decarboxylase. - Present in neurons of hypothalamus and in Mast cells and GI tract Glutamate: - An amino acid - Secreted by the neurons of the sensory pathways and cerebral cortex - Excitatory - It’s receptor has 4 types: 3 are ionotropic receptors and the 4th is metabotropic receptor. Glycine: - An amino acid - Secreted mainly at synapses in the spinal cord - Inhibitory - It increases Cl- conductance in postsynaptic cell membrane making it hyperpolarized. Gamma-aminobutyric acid (GABA): - An amino acid - Synthesized by the nerve terminals in the spinal cord, cerebellum, basal ganglia and cortex. - Inhibitory - Made from glutamic acid by glutamic acid decarboxylase - It does not have any metabolic function. - It has 2 types of receptors: ionotropic and metabotropic - Huntington disease is caused by GABA deficiency. Nitric Oxide (NO): - Made from Arginine by NO synthase, producing it and Citrulline - It is especially secreted by nerve terminals in areas of the brain responsible for long-term behavior and memory. - Nitric oxide is different from other small-molecule transmitters in its mechanism of formation in the presynaptic terminal and in its actions on the postsynaptic neuron ▪ It is not preformed and stored in vesicles in the presynaptic terminal. Instead, it is synthesized almost instantly as needed and it diffuses out of the presynaptic rather than being released in vesicular packets ▪ In the postsynaptic neuron, it does not greatly alter the membrane potential but instead changes intracellular metabolic functions that modify neuronal excitability NEUROPEPTIDES: - They are synthesized as integral parts of protein molecules by ribosomes in the neuronal cell body ▪ The protein molecules enter the ER and Golgi apparatus, where two changes occur: - The protein is split into smaller fragments (either the neuropeptide itself or a precursor of it) - The Golgi apparatus packages the neuropeptide into vesicles ▪ Vesicles are released into the cytoplasm and transported to tips of nerve fibers (axonal streaming), where they release neurotransmitters in response to action potentials. The vesicles are autolyzed, not reused. - Neuropeptides are acting much slower than small-molecule transmitters - They are much more potent than small-molecule transmitters - They cause much more prolonged actions - Examples of neuropeptides include: Somatostatin, prolactin, LH, growth hormone, vasopressin (ADH), oxytocin, gastrin, cholecystokinin (CCK), insulin, glucagon, angiotensin II, calcitonin. PURINES: - Adenosine triphosphate (ATP) and adenosine function as neuromodulators in the autonomic and central - ATP is synthesized in the sympathetic neurons that innervate vascular smooth muscle. - ATP is co-stored and co-secreted with the regular neurotransmitter of these neurons, norepinephrine 88. Describe the mechanisms used by excitatory and inhibitory receptors in the postsynaptic membrane. EXCITATORY POSTSYNAPTIC RECEPTORS: - Excitatory postsynaptic potentials (EPSP) depolarize the postsynaptic cell, bringing the membrane potential closer to threshold ▪ Increase the membrane’s permeability to Na+. The rapid influx of positively charged sodium ions to the interior causes resting membrane potential to increase in the positive direction toward the threshold level for excitation ▪ Decreased conduction through Cl- channels. This action decreases the diffusion of negatively charged chloride ions to the inside of the postsynaptic neuron, which causes the internal membrane potential to be more positive than normal, which is excitatory. ▪ Decreased permeability of K+ channels. This will prevent K+ ions from moving to the exterior of the cell, which will cause internal membrane potential to be more positive. - If ESPS potential rises high enough in the positive direction, it will elicit an action potential in the postsynaptic neuron. ▪ Discharge of a single presynaptic terminal can never increase the neuronal potential enough to elicit action potential. An increase of this magnitude requires simultaneous discharge of many terminals (40-80) ▪ Action potential does not begin adjacent to the excitatory synapses. It begins in the initial segment of the axon where the axon leaves the soma, because of large numbers of Na+ channels. INBHIBITORY POSTSYNAPTIC RECEPTORS: - Inhibitory postsynaptic potentials (IPSP) cause hyperpolarization of the cell, by increasing the intracellular negativity ▪ Opening of Cl- channels. This will allow negatively charged chloride ions to move to the interior, which will make interior membrane potential more negative than normal. ▪ Opening of K+ channels. This will allow positively charged potassium to move to the exterior, which will cause the interior membrane potential to be even more negative. 89. What are astrocytes? Describe their functions. ASTROCYTES (astroglia) – the most abundant glial cells in the brain that are closely associated with neuronal synapses FUNCTIONS OF ASTROCYTES: - They are involved in the physical structuring of the brain. - They regulate the transmission of electrical impulses within the brain. - They provide neurons with nutrients such as lactate (from glucose). - Astrocyte activity is linked to blood flow in the brain. However, astrocyte end-feet encircling endothelial cells DO NOT aid in the maintenance of the blood–brain barrier. Instead, it is the tight junctions and basal lamina of the cerebral endothelial cells that play the most substantial role in maintaining the barrier. - Astrocytes express plasma membrane transporters for several neurotransmitters, including glutamate, ATP, and GABA. Astrocytes were shown to release glutamate or ATP in a vesicular, Ca2+-dependent manner. - Regulation of K+ ion concentration in the extracellular space: Because astrocytes are highly permeable to K+, they rapidly clear the excess of these ions in the extracellular space when neurons are active. ▪ If this function is interfered, the extracellular concentration of K+ will rise, leading to neuronal depolarization. ▪ Abnormal accumulation of extracellular potassium is well-known to result in epileptic neuronal activity! - Modulation of synaptic transmission ▪ In the supraoptic nucleus of the hypothalamus, rapid changes in astrocyte morphology have been shown to affect synaptic transmission between neurons. ▪ In the hippocampus, astrocytes suppress synaptic transmission by releasing ATP, which is hydrolyzed to adenosine that acts on its receptors to inhibit synaptic transmission during LTP. - Astrocytes may serve as intermediaries in neuronal regulation of blood flow. - Promotion of the myelinating activity of oligodendrocytes by secreting leukemia inhibitory factor (LIF) under influence of ATP, which is released from neurons due to their electrical activity - Upon injury to nerve cells within the central nervous system, astrocytes fill up the space to form a glial scar, repairing the area and replacing the CNS cells that cannot regenerate. - Astrocytes are in close association with presynaptic and postsynaptic terminals, and they have the ability to integrate synaptic activity and release neuromodulators (= tripartite synapse). ▪ Within the dorsal horn of the spinal cord, activated astrocytes have the ability to respond to almost all neurotransmitters released by afferent cells of dorsal root ganglia and interneurons and, upon activation, astrocytes release neuroactive molecules such as: glutamate, ATP, nitric oxide (NO), prostaglandins (PG) or D-serine, which in turn influences neuronal excitability. 90. Describe in detail the function of the cerebellum in overall motor control. The functions of the cerebellum are coordination of movement, planning and execution of movement, maintenance of posture, and coordination of head and eye movements. The cerebellum helps control the rate, range, force, and direction of movements (collectively known as synergy). Damage to the cerebellum results in lack of coordination. There are three main divisions of the cerebellum: - The vestibulocerebellum controls balance and eye movements ▪ It functions in association with the brain stem and spinal cord to control equilibrium and postural movements ▪ Vestibulocerebellum is important in controlling balance between agonist and antagonist muscle contractions of the spine, hips, and shoulders during rapid changes in body positions ▪ The signals from the periphery tell the brain how rapidly and in which directions the body parts are moving. The vestibulocerebellum calculates in advance from these rates and directions where the different parts will be during the next few milliseconds. - The spinocerebellum controls synergy of movement ▪ It receives two types of information when a movement is performed: - Information from the cerebral motor cortex and from the midbrain red nucleus, telling the cerebellum the intended sequential plan of movement for the next few fractions of a second, - Feedback information from the peripheral parts of the body telling the cerebellum what actual movements is ▪ After receiving these informations it sends corrective output signals ▪ This part of the cerebellar motor control system provides smooth, coordinated movements of the agonist and antagonist muscles of the distal limbs for performing acute purposeful patterned movements ▪ Cerebellum damps the movement to prevent overshooting and tremor. - The cerebrocerebellum controls the planning and initiation of movements ▪ Cerebrocerebellum doesn’t communicate with primary cerebral motor cortex. It communicates mainly with premotor area, primary somatosensory area and association somatosensory area. ▪ An important function of the lateral zones of the cerebellar hemispheres is to provide appropriate timing for each succeeding movement. 91. Describe the basal ganglia and their motor functions. BASAL GANGLIA: - The basal ganglia are the deep nuclei of the telencephalon: caudate nucleus, putamen, globus pallidus, amygdala, subthalamic nuclei and substantia nigra. - They function in close association with the cerebral cortex and corticospinal motor control system - The main function of the basal ganglia is to influence the motor cortex via pathways through the thalamus. The basal ganglia aid in planning and execution of smooth movements and contribute to affective and cognitive functions. Motor functions of basal ganglia: - Executing learned patterns of movement (putamen circuit) ▪ The basal ganglia function in association with the corticospinal system ▪ e.g. writing letters of the alphabet, cutting paper with scissors, hammering nails, shooting a basketball through the hoop, passing a football, vocalization, controlled eye movements, skilled movements - Cognitive control of motor activity (caudate circuit) ▪ Instinctive knowledge ▪ Cognitive control of motor activity determines subconsciously, and within seconds, which patterns of movement will be used together to achieve a complex goal that might itself last for many second 92. Describe the role of the brainstem in the motor functions control. BRAINSTEM: - The brain stem consists of the medulla, pons, and mesencephalon. ▪ It contains motor and sensory nuclei that perform motor and sensory functions for the face and head regions - It provides special control functions such as: ▪ Control of respiration ▪ Control of cardiovascular system ▪ Partial control of GI function ▪ Control of many stereotyped movements of the body ▪ Control of equilibrium ▪ Control of eye movements MOTOR FUNCTIONS OF THE BRAINSTEM – SUPPORT OF THE BODY AGAINST GRAVITY Reticular nuclei: Reticular nuclei is divided into: pontine reticular nuclei and medullary reticular nuclei. These nuclei function antagonistically to each other. Pontine reticular nuclei: - Excites antigravity muscles (muscles of the vertebral column, extensor muscles of the limbs) which support the body against gravity - Pontine reticular nuclei receive excitatory signals from the vestibular nuclei, and from deep nuclei of the cerebellum - Excitatory signals from the pontine reticular system powerful enough that the antigravity muscles can be active without signals from the higher levels of the brain. Medullary reticular nuclei: - Relaxes antigravity muscles (= axial muscles) by transmitting inhibitory signals to their neurons ▪ Medullary reticular system counterbalances the excitatory signals from the pontine reticular system, so under normal conditions the body muscles are not abnormally tens - They receive strong input collaterals from: the corticospinal tract, the rubrospinal tract, and other motor pathways. Vestibular nuclei: - All the vestibular nuclei function in association with the pontine reticular nuclei to control the antigravity muscles - Transmit strong excitatory signals to the antigravity muscles - The specific role of the vestibular nuclei is to selectively control the excitatory signals to the different antigravity muscles to maintain equilibrium in response to signals from the vestibular apparatus 93. Describe the transmission of signals from the motor cortex to the skeletal muscles. Motor signals are transmitted directly from motor cortex to spinal cord via the corticospinal (pyramidal) tract and indirectly through multiple accessory pathways that involve the basal ganglia, cerebellum, and nuclei of the brain stem. CORTICOSPINAL (PYRAMIDAL) TRACT: - It is the most important output pathway from the motor cortex - It originates from: primary motor cortex (30%), premotor and supplementary motor areas (30%), and from the somatosensory areas posterior to the central sulcus (40%) - The majority of the pyramidal fibers cross in the lower medulla to the opposite side and descend into the lateral corticospinal tracts of the cord. Some of the fibers do not cross to the opposite side in the medulla but pass ipsilaterally down the cord in the ventral corticospinal tracts. - It terminates principally on the interneurons in the intermediate regions of the cord gray matter 94. Describe the formation, flow, and absorption of cerebrospinal fluid. CEREBROSPINAL FLUID (CSF): Characteristics of CSF: - Osmotic pressure is equal to that of plasma - Na+ concentration is equal to plasma Na+ concentration - Cl- concentration is higher than plasma Cl- concentration - K+ and glucose concentrations are lower than in plasma Formation of CSF: - It is formed at a rate of about 500 ml each day - Secreted from choroid plexus of ventricles (mainly from lateral ventricles) ▪ Choroid plexus projects into the temporal horn of each lateral ventricle, the posterior portion of the third ventricle, and the roof of the fourth ventricle ▪ It is covered by a thin layer of epithelial cells ▪ Choroid plexus actively secrete sodium ions through the epithelial cells, which pull along chloride ions. This results in osmosis of water through the membrane. Flow of CSF: Lateral ventricles → third ventricle → aqueduct of Sylvius → fourth ventricle → lateral foramina of Luschka and midline foramen of Magendie → cisterna magna → subarachnoid space → arachnoid villi → arachnoid granulations → sagittal venous sinus Absorption of CSF: - CSF is absorbed by arachnoid villi, which are projections of the arachnoid membrane - Fluid empties into the venous blood through pores of endothelial cells covering the villi ▪ Endothelial cells have vesicular passages directly through the bodies of the cells large enough to allow free flow of: cerebrospinal fluid, dissolved protein molecules, and even particles as large as red and white blood cells into the venous blood ▪ In the steady state, the movement of fluid from CSF to venous blood should equal the rate of CSF formation (500 ml/day) 95. What is a monosynaptic reflex. Describe its physiological function. Describe the structure and function of the receptor used in this reflex. Give an example of a monosynaptic reflex in our body and describe the reflex arc of this reflex. MONOSYNAPTIC REFLEX: - Monosynaptic refers to the presence of a single chemical synapse in the CNS. - A reflex consists of one sensory neuron, and one motor neuron. There is no interneuron, which allows the signal to return with the shortest possible time delay back to the muscle. - The receptor involved in a monosynaptic reflex is the muscle spindle. ▪ It is a sensory receptor that detects the length or rate of change of length of muscle. ▪ Its composed of: - Intrafusal fibers which are further divided into nuclear bag and chain fibers ▪ The intrafusal fibers are stimulated by type A gamma efferent fibers from type A gamma motor neurons in ant. Horn of SP - Extrafusal fibers ▪ The extrafusal fibers are stimulated by type A alpha efferent fibers from type A alpha motor neurons in anterior horn of spinal cord KNEE (PATTELAR) REFLEX: - The patellar reflex is a clinical and classic example of the monosynaptic reflex arc ▪ There is no interneuron in the pathway leading to contraction of the quadriceps muscle. Instead the bipolar sensory neuron synapses directly on a motor neuron in the spinal cord. - There is an inhibitory interneuron used to relax the antagonistic hamstring muscle ▪ The Ia axons also elicit inhibitory interneurons that reciprocally innervate the motor neurons of the antagonist muscle of the quadriceps (hamstring muscles), resulting in relaxation of the semitendinosus muscle. The reflex relaxation of the antagonistic muscle is polysynaptic - It is: ▪ Unconditional – an inborn, unlearned response to a stimulus ▪ Deep ▪ Somatic – skeletal muscle responds ▪ Proprioreceptive – receptors located in muscles detect subtle changes in movement, position, tension, and force, within the body ▪ Monosynaptic ▪ Myotatic - it is specific for the same muscle that is stretched Reflex arc: - Receptor: muscle spindle - Sensory neuron: primary sensory afferent nerves, which convey their information through group Ia axons - Control center: spinal cord, anterior horn - Motor neuron: α-motor neuron - Effector: quadriceps muscle 96. Characterize muscle stretch reflexes. Describe a reflex arc of a muscle stretch reflex. Explain the differences between dynamic and static stretch reflexes. STRETCH REFLEX: - A stretch reflex (myotatic) is a muscle contraction in response to stretching of the muscle. - It is a monosynaptic reflex which provides automatic regulation of skeletal muscle length. - Examples: jaw jerk reflex, biceps reflex, brachioradialis reflex, extensor digitorum reflex, triceps reflex, patellar reflex (knee-jerk), ankle jerk reflex Reflex arc: - Receptor: muscle spindle - Sensory neuron: primary sensory afferent nerves - Control center: spinal cord - Motor neuron: α-motor neuron - Effector: muscle Static stretch reflex: - It takes place as long as the muscle is stretched. The dynamic phase. - It functions to maintain a constant contraction of a muscle. Dynamic stretch reflex: - It occurs only when the muscle is stretched e.g. when the tendon is struck with a tendon hammer - It function to oppose sudden changes in muscle length - It is initiated by rapid stretch or unstretch of a muscle - This reflex is finished a fraction of a second after the muscle has been stretched or unstretched. 97. Describe in detail the role of muscle spindles and Golgi tendon organs in muscle control. MUSCLE SPINDLE: - It is a sensory receptor that detects the length or rate of change of length of muscle. ▪ Stretching of the tendon pulls on the muscle spindle, exciting the primary sensory afferents, which convey their information through group Ia axons to the α motor neurons of spinal cord - The mammalian muscle spindle is a complex of modified skeletal muscle combined with both afferent and efferent innervation. - Its composed of: ▪ Intrafusal fibers which are further divided into nuclear bag and chain fibers - The intrafusal fibers are stimulated by type A gamma efferent fibers from type A gamma motor neurons in ant. Horn of SP ▪ Extrafusal fibers - The extrafusal fibers are stimulated by type A alpha efferent fibers from type A alpha motor neurons in anterior horn of spinal cord - The spindle does not contribute significant force generation to the muscle but serves a purely sensory function GOLGI TENDON ORGAN: - It measures the force generated by muscle by measuring the tension in its tendon - Golgi tendon organ prevents muscle damage by inhibiting the activity of motor neurons innervating the muscles ▪ Golgi tendon organ, are activated upon stretch of the tendon, which requires considerable force. These sensors synapse on interneurons in the spinal cord that inhibit further activity of the motor neurons innervating the muscle. This relaxation of the muscle prevents damage from excess force 98. Describe the reflex arc of a flexor reflex. What is the physiological function of this reflex? FLEXOR REFLEX (WITHDRAWAL REFLEX): - The withdrawal reflex (nociceptive or flexor withdrawal reflex) is a spinal reflex intended to protect the body from damaging stimuli (e.g. hot object) ▪ On the ipsilateral side (the same) the excitatory nerves will stimulate flexors of a limb to contract. Whereas inhibitory nerves will inhibit antagonist muscles (extensors). This is known as reciprocal inhibition. ▪ On the contralateral side of the pain stimulus, reflexes are activated that cause extensor muscles to contract and flexor muscles to relax, which causes extension on the contralateral side (crossed-extension reflex). Thus, if the painful stimulus occurs on the left side, the left arm and leg will flex or withdraw and the right arm and leg will extend to maintain balance. - It is: ▪ Defence ▪ Unconditional – inborn, unlearned response to a stimulus ▪ Somatic – skeletal muscle response ▪ Exteroreceptive – exteroreceptors receive information from the environment external to the organism ▪ Polysynaptic – pathways where one or more interneurons connect afferent (sensory) and efferent (motor) signals Reflex arc: - Receptor: any receptors in skin (e.g. temperature, pain receptors, chemoreceptors) - Sensory neuron: group Aδ axons enter dorsal horn of spinal cord - Control center: spinal cord, where afferent fibres synapse on interneurons - Motor neuron: α-motor neuron - Effector: biceps muscle (stimulation), triceps muscle (inhibition) 99. Describe the reflex arc of a crossed extensor reflex. What is the physiological function of this reflex? CROSSED EXTENSOR REFLEX: - It is a withdrawal reflex - It is polysynaptic - It functions in pushing the body away from an object that cause a painful stimulus. It maintains balance. - When the reflex occurs the flexors in the withdrawing limb contract and the extensors relax, while in the other limb, the opposite occurs Reflex arc: - Receptor: any receptors in skin (e.g. temperature, pain receptors, chemoreceptors) - Sensory neuron: group Aδ axons enter the dorsal horn of the spinal cord. Branches of the afferent nerve fibers cross from the stimulated side of the body to the contralateral side of the spinal cord - Control center: spinal cord, where afferent fibres synapse on interneurons - Motor neuron: α-motor neuron - Effector: muscles of contralateral limb 100. Describe the reflex arc of a pupillary light reflex. Explain what is a consensual light reflex, and why is it possible. PUPILLARY LIGHT REFLEX - It is not usually called a „reflex”!!! - It is a reflex that controls the diameter of the pupil, in response to the intensity of light that falls on the retina of the eye. ▪ Direct light reflex – pupillary contraction in 0.3 – 0.8 s when you shine light onto retina of this eye. ▪ Consensual light reflex – a normally present crossed reflex in which light directed at one eye causes the opposite pupil to contract. The contralateral efferent limb causes consensual light reflex of the contralateral pupil Reflex arc of the pupillary light reflex: - Receptors: rods and cones - Sensory neurons: I sensory neuron - rods and cones, II sensory neuron - bipolar cells, III sensory neuron - ganglion cells, IV sensory neuron - Pretectal nucleus - Control center: Edinger – Westphal nucleus (parasympathetic motor nucleus located in the midbrain) - Afferent pathway: Ciliary ganglion - Effector: Constrictor muscle of the iris 101. What is accommodation. What changes are observed in accommodation reflex, when moving focus from a distant to near object – and what is a physiological meaning of these changes. ACCOMMODATION: - It is the ability of the eye to change its focus from distant to near objects Accommodation reflex: - The accommodation reflex is an action of the eye, in response to focusing on a near object, then looking at distant object (and vice versa), comprising coordinated changes in vergence, lens shape and pupil size. - It is controlled by parasympathetic nervous system. ▪ Stimulation of parasympathetic nerves → contraction of ciliary muscles → relaxation of lens ligaments → lens becomes thicker (more convex) → increased refractive power of the lens → eye focuses on near objects ▪ As distant objects move toward the eye, the number of parasympathetic impulses increase - The pupil constricts in order to prevent diverging light rays from hitting the periphery of the retina and resulting in a blurred image. Reflex arc: - Receptor: rods and cons - Sensory neuron: optic nerve (CN II) - Control center: superior centres (third nerve nucleus in brain stem) - Motor neuron: oculomotor nerve (CN III) - Effector: ciliary muscles 102. Describe the classifications of memories according to the time period during which the information is stored and according to the type of information stored. What is consolidation of memory? Memory is the ability to store and to recall learned changes KINDS OF MEMORY (DEPENDING OF THE TIME WHEN THE INFORMATION IS REMEMBERED): Sensory memory: - Sensory memory corresponds approximately to the initial 200–500 milliseconds after an item is perceived. - Iconic memory – type of visual memory, echoic memory – type of auditory memory - This type of memory cannot be prolonged via rehearsal Short-term memory (working memory): - It allows to recall information for a period of several seconds to a minute without rehearsal - Its capacity is very limited - The information is circulating between limbic system and some other parts of the brain. Long-term memory: - It can store much larger quantities of information for potentially unlimited duration (sometimes a whole life span). - Its capacity is immeasurably large. KINDS OF MEMORY (DEPENDING OF THE SORT OF REMEMBERED INFORMATION): Declarative memory (explicit memory): - It is long term human memory - It refers to memories which can be consciously recalled such as facts and events. - There are two types of declarative memory: ▪ Semantic memories are those that store general factual knowledge that is independent of personal experience. Examples include types of food, capital cities, lexical knowledge (vocabulary), etc. ▪ Episodic memories are those that store specific events such as attending a class or flying to France. Retrieval of these memories can be thought of as mentally reliving the past events they concern. Episodic memory is believed by many to be the system that supports and underpins semantic memory. Procedural memory: - It is memory for how to do things – it guides the processes we perform - It resides below the level of conscious awareness. - When needed, procedural memories are automatically retrieved and utilized for the execution of the integrated procedures involved in both cognitive and motor skills (e.g. tying shoes, reading) MEMORY CONSOLIDATION: - It is the process where our brains converts short-term memories into long-term ones. - Rehearsal of the same information again and again in the mind accelerates and enhances consolidation - During consolidation, the new memories are not stored randomly in the brain but are stored in direct association with other memories of the same type - This process occurs in hippocampus but the information are not stored there. 103. Characterize rapid eye movement and slow-wave sleep stages of sleep cycle. REM SLEEP (PARADOXICAL SLEEP): - It is a type of sleep where the brain is highly active while person is not fully aware of their surroundings meaning they are fully asleep. - It normally lasts 5-30 minutes and appears on average every 90 minutes. (25% of the time of sleep) - The more rested we are, the longer REM sleep is - REM sleep is an active form of sleep associated with dreaming and irregular muscle movements despite muscle tone is very depressed throughout the body. - The brain is very active during this type of sleep. ▪ EEG brain waves are similar to that of an awake person (beta waves) ▪ This is why REM sleep is also called paradoxical sleep, because it is a paradox that a person can still be asleep despite the brain being in such an active state. SLOW-WAVE (NON REM) SLEEP: - It occurs when the brain waves are strong and of low frequency which produces delta waves. - It is exceedingly restful - It is associated with decreases in both peripheral vascular tone and many other vegetative functions of the body. - Dreams and even nightmares do occur during non-REM sleep but they are usually not remembered because consolidation of the dreams in memory does not occur. 104. What is EEG? Characterize all waves observed in EEG in adults. ELECTROENCEPHALOGRAPHY (EEG) - It is the recording of electrical activity along the scalp produced by the firing of neurons within the brain. It is a graphic picture of different frequency waves of brain. Each these waves (or rhythms) provides information about different brain states. ▪ Beta waves (14 to 60 Hz;