Physical Properties of Materials PDF
Document Details
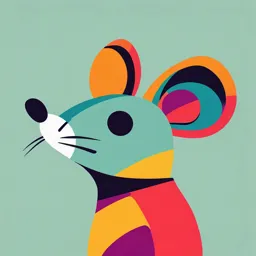
Uploaded by UnconditionalFluorite5972
Tags
Summary
This document provides a comprehensive overview of the physical properties of various materials, including metals, ceramics, and plastics. It explores factors like density, melting point, and thermal conductivity, and examines their roles in different applications.
Full Transcript
Physical Properties of Materials Physical properties have several significant roles in the selection, processing, and use of materials. They can also be key factors in determining a material’s suitability for specific applications, especially when considered simultaneously with...
Physical Properties of Materials Physical properties have several significant roles in the selection, processing, and use of materials. They can also be key factors in determining a material’s suitability for specific applications, especially when considered simultaneously with mechanical properties. Strength-to-weight and stiffness-to-weight ratios, as examples, are described in the context of lightweight designs, an important consideration especially in aerospace and automotive industries. Why is electrical wiring generally made of copper? Why are stainless steel, aluminum, and copper commonly used in cookware? Why are the handles of cookware usually made of plastic, while other types of handles are made of metal? What kind of material should be chosen for the heating elements in toasters and toaster ovens? Why does metal feel colder to the touch than plastic even though both are at room temperature? Why are the metallic components in some machines being replaced with ceramics? Why are commercial airplane bodies generally made of aluminum while others are made of reinforced plastics? From these questions it is apparent that a major criterion in material selection requires consideration of physical properties: density, melting point, specific heat, thermal conductivity, thermal expansion, electrical and magnetic properties, and resistance to oxidation and corrosion. Combinations of mechanical and physical properties, such as the strength- to-weight and stiffness-to-weight ratios of materials, are equally important, particularly for aircraft and aerospace structures. Also, high-speed equipment, such as textile and printing machinery, and forming and cutting machines for high-speed operations, require lightweight components to reduce inertial forces and prevent the machines from being subjected to excessive vibration. Physical Properties of Materials Density Melting Point Specific Heat Thermal Conductivity Thermal Expansion Electrical, Magnetic, and Optical Properties Corrosion Resistance The density of a material is its mass per unit volume; another term is specific gravity, which expresses a material’s density relative to that of water. Weight saving is particularly important for aircraft, aerospace, automotive structures, sports equipment, and for various other products where energy consumption and power limitations are significant concerns. Substitution of materials for weight savings and fuel economy is a major factor in the design both of advanced equipment and machinery and of consumer products, such as sporting goods, portable computers, and bicycles. A significant role that density plays is in the strength-to-weight ratio (specific strength) and stiffness-to-weight ratio (specific stiffness) of materials. Table 1: Physical Properties of Selected Materials at Room Temperature Figure 1 shows the ratio of maximum yield stress to density for a variety of metal alloys. Titanium and aluminum are at the top of the list; consequently, they are among the most commonly used metals for aircraft and aerospace applications. Figure 1: Ratio of maximum yield stress to density for selected metals. The ranges for specific tensile strength Table 3.2: Physical Properties of Materials, in Descending Order and specific stiffness at room temperature for a variety of metallic and nonmetallic materials are given in Fig. 2. Note the positions of composite materials, as compared to those of metals, with respect to these properties. These advantages have led composites to become among the most important materials. At elevated temperatures, specific strength and specific stiffness are likewise important considerations, especially for components operating at these temperatures, such as automotive and jet engines, gas turbines, and furnaces. Typical ranges for a variety Figure 2: Specific strength (tensile strength/density) of materials are given in Fig. 3. and specific stiffness (elastic modulus/density) for various materials at room temperature. Density is an important factor in the selection of materials for high-speed equipment, such as magnesium in printing and textile machinery, many components of which typically operate at very high speeds. Aluminum is used with some digital cameras for better performance in cold weather. Because of their low density, ceramics are being used for components in high-speed automated machinery and machine tools. On the other hand, there are applications where weight is desirable. Examples are Figure 3: Specific strength (tensile strength/density) for a variety of materials as a counterweights for various mechanisms (using function of temperature. Note the useful temperature range for these lead or steel), flywheels, ballasts on ships and materials and the high values for composite materials. MMC = metal-matrix composite; FRP = fiber-reinforced plastic. aircraft, and weights on golf clubs (using high- density materials such as tungsten). Melting Point The temperature range within which a component or structure is designed to function is an important consideration in selection of materials. Plastics, for example, have the lowest useful temperature range while ceramics, graphite, and refractory-metal alloys have the highest useful range. Pure metals have a definite melting point, whereas the melting temperature of a metal alloy can have a wide range (Table 1), depending on its composition. The melting point has several indirect effects on manufacturing operations. Because the recrystallization temperature of a metal is related to its melting point, operations such as annealing and heat treating and hot working require knowledge of the melting points of the materials involved. These considerations are also important in the selection of tool and die materials. In casting operations, melting point plays a major role in the selection of the equipment and the melting practice employed. Specific Heat: A material’s specific heat is the energy required to raise the temperature of a unit mass by one degree. Alloying elements have a relatively minor effect on the specific heat of metals. The temperature rise in a workpiece, such as those in forming or machining operations, is a function of the work done and of the specific heat of the workpiece material. An excessive temperature rise in a workpiece can a) decrease product quality by adversely affecting its surface finish and dimensional accuracy, b) cause excessive tool and die wear, and c) result in undesirable metallurgical changes in the material. Thermal conductivity indicates the rate at which heat flows within and through a material. Metallically bonded materials (metals) generally have high thermal conductivity, while ionically or covalently bonded materials (ceramics and plastics) have poor conductivity. Alloying elements can have a significant effect on the thermal conductivity of alloys, as can be seen by comparing pure metals with their alloys. In general, materials with high electrical conductivity also have high thermal conductivity. Thermal conductivity is an important consideration in numerous applications. For example, high thermal conductivity is desirable in cooling fins, cutting tools, and die-casting molds to extract heat quickly. In contrast, materials with low thermal conductivity are used, for instance, in furnace linings, insulation, coffee cups, and handles for pots and pans. One function of a lubricant in hot metalworking is to act as an insulator to keep workpieces hot and formable. The thermal expansion of materials can have several significant effects, particularly the relative expansion or contraction of different materials in assemblies. Generally, the coefficient of thermal expansion is inversely proportional to the melting point of the material. Alloying elements have a relatively minor effect on the thermal expansion of metals. Thermal expansion in conjunction with thermal conductivity plays the most significant role in the development of thermal stresses (due to temperature gradients), To reduce thermal stresses, a combination of high thermal conductivity and low thermal expansion is desirable. Thermal stresses also can be caused by anisotropy of thermal expansion; that is, the material expands differently in different directions. Thermal expansion and contraction can lead to cracking, warping, or loosening of assembled components during their service life, as well as cracking of ceramic parts and in tools and dies made of relatively brittle materials. Thermal fatigue results from thermal cycling and causes a number of surface cracks. Thermal shock is the term generally used to describe the development of a crack or cracks after being subjected to a single thermal cycle. To alleviate some of the problems caused by thermal expansion a family of iron-nickel alloys with very low thermal-expansion coefficients are available, called low-expansion alloys. The low thermal expansion characteristic of these alloys is often referred to as the Invar effect, after the metal Invar. Their thermal coefficient of expansion is typically in the range of from 2 to 9 µm per ºC. Low-expansion alloys also have good thermal-fatigue resistance, and because of their good ductility they can easily be formed into various shapes. Electrical conductivity and the dielectric properties Electrical conductivity and the dielectric properties of materials are important not only in various electrical equipment and machinery, but also in such manufacturing processes as magnetic-pulse forming, resistance welding, and electrical-discharge machining and electrochemical grinding of hard and brittle materials. Alloying elements have a major effect on the electrical conductivity of metals: The higher the conductivity of the alloying element, the higher is the electrical conductivity of the alloy. Dielectric Strength. An electrically insulating material’s dielectric strength is the largest electric field to which it can be subjected without degrading or losing its insulating properties. This property is defined as the voltage required per unit distance for electrical breakdown, and has the units of V/m. Conductors. Materials with high electrical conductivity, such as metals, are generally referred to as conductors. Electrical resistivity is the inverse of electrical conductivity. Materials with high electrical resistivity are referred to as dielectrics or insulators. Superconductors. Superconductivity is the phenomenon of near-zero electrical resistivity that occurs in some metals and alloys below a critical temperature. The temperatures involved often are near absolute zero (0 K, –273◦C). The highest temperature at which superconductivity has to date been exhibited, at about –123◦C, is with an alloy of thallium, barium, calcium, copper, and oxygen; other material compositions are continuously being investigated. The main application of superconductors is largely for high-power magnets. Superconductors are the enabling technology for magnetic resonance imaging (MRI), used for medical imaging. Other applications envisioned for superconductors include magnetic levitation (maglev) trains, efficient power transmission lines, and extremely fast computer components. Semiconductors. The electrical properties of semiconductors, such as single-crystal silicon, germanium, and gallium arsenide, are extremely sensitive to temperature and to the presence and type of minute impurities. Thus, by controlling the concentration and type of impurities (called dopants), such as phosphorus and boron in silicon, electrical conductivity can be controlled. This property is utilized in semiconductor (solid-state) devices, used extensively in miniaturized electronic circuitry. Ferromagnetism and Ferrimagnetism Ferromagnetism is a phenomenon characterized by high permeability and permanent magnetization that are due to the alignment of iron, nickel, and cobalt atoms into domains. It is important in such applications as electric motors, electric generators, electric transformers, and microwave devices. Ferrimagnetism is a permanent and large magnetization exhibited by some ceramic materials such as cubic ferrites. Piezoelectric Effect. The piezoelectric effect (piezo from Greek, meaning to press) is exhibited by smart materials. Two basic behaviors are involved: (a) When subjected to an electric current, they undergo a reversible change in shape, by as much as 4% and (b) when deformed by an external force, they emit a small electric current. Piezoelectric materials include quartz crystals and some ceramics and polymers. The piezoelectric effect is utilized in making transducers, which are devices that convert the strain from an external force into electrical energy. Typical applications are sensors, force or pressure transducers, inkjet printers, strain gages, sonar detectors, and microphones. As an example, an air bag in an automobile has a sensor that, when subjected to an impact force, sends an electric charge that then deploys the bag. Magnetostriction. The phenomenon of expansion or contraction of a material when subjected to a magnetic field is called magnetostriction. Pure nickel and some iron–nickel alloys exhibit this behavior. Magnetostriction is the principle behind ultrasonic machining equipment. Magnetorheostatic and Electrorheostatic Effects. When subjected to magnetic or electric fields, some fluids undergo a major and reversible change in their viscosity within a fraction of a second, turning from a liquid to an almost solid state. For example, magnetorheostatic behavior is attained by mixing very fine iron filings with oil. Called smart fluids, they are being developed for such applications as vibration dampeners, engine mounts, prosthetic devices, clutches, and valves. Optical Properties. Among various other properties, color and opacity are particularly relevant to polymers and glasses. Corrosion Resistance: Corrosion not only leads to surface deterioration of components and structures, such as bridges and ships, but also reduces their strength and structural integrity. The direct cost of corrosion to the U.S. economy alone has been estimated to be over $400 billion per year, approximately 2% of the gross domestic product; indirect costs of corrosion are estimated at twice this amount. Metals, ceramics, and plastics are all subject to forms of corrosion. The word corrosion itself usually refers to the deterioration of metals and ceramics, while similar phenomena in plastics are generally called degradation. Corrosion resistance is an important aspect of material selection for applications in the chemical, petroleum, and food industries, as well as in manufacturing operations. In addition to various possible chemical reactions from the elements and compounds present, environmental oxidation and corrosion of a wide range of components and structures is a major concern, particularly at elevated temperatures. Resistance to corrosion depends on the composition of the material and on its particular environment. Corrosive media may consist of various chemicals (acids, alkalis, and salts) and the environment (oxygen, moisture, pollution, and acid rain), including water (fresh or salt water). Nonferrous metals, stainless steels, and nonmetallic materials generally have high corrosion resistance. Steels and some cast irons generally have poor resistance and must be protected by a variety of coatings and surface treatments. Corrosion can occur over an entire surface or it can be localized, called pitting. Pitting is a term that is also used for fatigue wear or failure of gears and in forging. Corrosion can also occur along grain boundaries of metals as intergranular corrosion, and at the interface of bolted or riveted joints as crevice corrosion. Two dissimilar metals may form a galvanic cell (after L. Galvani, 1737– 1798); that is, two electrodes in an electrolyte in a corrosive environment that includes moisture and cause galvanic corrosion. Two-phase alloys are more susceptible to galvanic corrosion than are single-phase alloys or pure metals. As a result, heat treatment can have a significant influence on corrosion resistance. Stress-corrosion cracking is an example of the effect of a corrosive environment on the integrity of a product that, as manufactured, contained residual stresses. Likewise, cold-worked metals are likely to have residual stresses, thus making them more susceptible to corrosion than are hot- worked or annealed metals. Tool and die materials also can be susceptible to chemical attack by lubricants and coolants. The chemical reaction alters their surface finish and adversely influences the metalworking operation. One example is carbide tools and dies with cobalt as a binder; the cobalt is attacked by elements in the metalworking fluid, called selective leaching. The compatibility of the tool, die, and workpiece materials with the metalworking fluid, under actual operating conditions, is thus an important consideration. Chemical reactions should not always be regarded as having only adverse effects. Advanced machining processes, such as chemical and electrochemical machining, are indeed based on controlled chemical reactions. These processes remove material by chemical action in a manner similar to etching of metallurgical specimens. The usefulness of some level of oxidation is demonstrated also by the corrosion resistance of aluminum, titanium, and stainless steel. Aluminum, for example, develops a thin (a few atomic layers), strong and adherent hard-oxide film (Al2O3) that better protects the surface from further environmental corrosion. Titanium develops a film of titanium oxide (TiO2); a similar phenomenon occurs in stainless steels which, because of the chromium present in the alloy, develop a protective film. These processes are known as passivation. When the protective film is scratched and exposes the metal underneath, a new oxide film begins to form.