Seamount Evolution & Sedimentary Processes in Marine Environments
Document Details
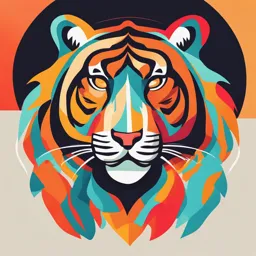
Uploaded by ResponsiveAgate9401
2024
Tags
Summary
The document discusses seamount evolution, sedimentary processes in submarine environments, and contourite depositional systems. It covers topics such as bottom currents, contourite drifts, and seismic criteria for identifying contourite drifts. The document also touches on submarine mass movements and glacial sedimentary processes.
Full Transcript
75 36.5 Seamount Evolution and Geological Implications Recent advancements in mapping seamounts with high-resolution multi-beam sonar have revealed that many seamounts have flat tops. These findings have led to a growing interest in seamounts as they often harbor new species, unique habitats, an...
75 36.5 Seamount Evolution and Geological Implications Recent advancements in mapping seamounts with high-resolution multi-beam sonar have revealed that many seamounts have flat tops. These findings have led to a growing interest in seamounts as they often harbor new species, unique habitats, and important geochemical discoveries. Addition- ally, seamounts have become significant in the context of mineral exploration, as they are potential sources of valuable minerals, such as cobalt, which are sought after by various industries. The scientific community has recognized the importance of seamounts, and recent studies have cataloged approximately 25,000 seamounts globally. The discovery of new species and ecosystems around seamounts highlights their ecological importance, making them a key area of interest in marine research. 37 Lecture 12, 11.12.2024 38 Sedimentary Processes in the Submarine Environment The submarine environment is shaped not only by endogenic processes such as tectonics and volcanism but also by sedimentary processes. These processes are responsible for the redistribution of sediments, which originate from various sources. The marine environment can be visualized as a basin filled with sediment that is transported and deposited through different mechanisms. 38.1 Classification of Sedimentary Processes Sedimentary processes in the submarine environment can be classified into two main types: Along-slope sedimentary processes: These processes transport and deposit sediment along the slope, maintaining a relatively constant depth. Down-slope sedimentary processes: These involve the transport of sediment from shallow environments to deeper regions. This distinction is fundamental in understanding sediment dynamics in the marine environment. 76 38.2 Bottom Currents and Their Role Bottom currents play a crucial role in shaping the continental margin at various spatial and temporal scales. Unlike wave motions, which influence only shallow depths near the coastline (down to a few tens of meters), bottom currents occur in deeper environments and significantly impact the seafloor. Marine geologists identify four main types of bottom currents: 1. Thermohaline-induced geostrophic bottom currents (contour currents) 2. Wind-driven bottom currents 3. Deep-water tidal bottom currents 4. Internal waves and tides Thermohaline-induced geostrophic bottom currents are primarily responsible for the general circulation of ocean water masses, often referred to as the conveyor belt circulation. This process is driven by temperature and salinity variations, particularly in polar regions, where cooling and ice formation increase water density, causing it to sink. The movement of these dense water masses towards the equator along the seafloor is influenced by Earth’s rotation, which results in a deflection known as the geostrophic effect. This deflection pushes water masses against the continental margin, affecting sediment erosion, transport, and deposition. 38.3 Contourite Deposits The interaction between bottom currents and the seafloor leads to the formation of depositional features called contourites. These sedimentary bodies accumulate along continental margins due to the sustained action of bottom currents. A typical contourite system consists of: 77 An erosional feature where bottom currents reach maximum velocity. A depositional area where sediment accumulates over time, forming distinct sedimentary bodies known as contourite drifts. Among the four types of bottom currents, only thermohaline-induced geostrophic currents and wind-driven bottom currents are capable of forming contourites. Deep-water tidal currents and internal waves, on the other hand, do not generate contourite systems independently. These processes play a fundamental role in shaping the deep-sea environment, influencing sediment distribution, and contributing to the complexity of submarine landforms. 39 Contourite Depositional Systems Contourite depositional systems are influenced by bottom currents, which play a crucial role in shaping sedimentary structures and bedforms. These systems can form independently or in combination with other hydrodynamic processes, leading to the creation of distinct morphological features. 39.1 Structure of Contourite Depositional Systems A contourite drift consists of various sedimentary features that evolve due to variations in bottom current velocities. In a three-dimensional view, these drifts exhibit complex formations, while a two- dimensional cross-section highlights the areas where bottom currents reach maximum velocities. The interaction of currents with the seafloor leads to smaller-scale features on the drift surface, which change according to velocity fluctuations. The contourite depositional system is characterized by different sedimentary structures, in- cluding dunes, giant sediment waves, and erosional features such as furrows and moats. The positioning of these features is controlled by current velocity, with higher-energy regions dominated by larger bedforms and lower-energy areas exhibiting finer sediment deposits. 39.2 Sedimentary Features and Morphological Variability The main sedimentary features in contourite systems include: 78 Dunes and Giant Sediment Waves: These dominate the upper sections of the system where currents are strongest. Furrows: Linear incisions on the seafloor, indicative of erosive activity. Moats: Depressions associated with current-controlled sediment transport. Ripples and Faint Lineations: Found in slower-current regions, indicative of finer sediment accumulation. It is essential to recognize that contourite depositional systems exhibit both depositional and erosional characteristics. The variations in current strength along the system lead to a range of bedforms that provide insights into past and present oceanographic conditions. 39.3 Geostrophic Bottom Currents and Seismic Representation Semi-permanent geostrophic bottom currents, particularly those driven by thermohaline circulation, play a fundamental role in shaping these sedimentary environments. These currents generate a wide range of bedforms and contribute to the development of large-scale sedimentary bodies. Seismic reflection methods are commonly used to analyze contourite systems. Seismic data reveal stratigraphic structures where sedimentary strata converge towards moats, demonstrating the depositional nature of these systems. The dimensions of contourite drifts vary significantly, with some systems reaching up to 10,000 km² in area and thicknesses of up to 2 km. 39.4 Correlation Between Seismostratigraphy and Water Masses Studying contourite systems requires integrating seismostratigraphic data with oceanographic observations. Scientists analyze seismic reflections alongside water column characteristics, such as temperature and salinity, often measured using CTD (Conductivity, Temperature, Depth) profiles. These correlations help identify the cores of water masses responsible for sediment transport and deposition along continental slopes. A key observation is that contourite drifts are typically associated with specific water masses. Dense shelf water cascading along the slope contributes to the formation of these sedimentary bodies, creating a direct link between ocean circulation and deep-sea sedimentation. 39.5 Study Approaches and Key Considerations To fully comprehend contourite depositional systems, researchers employ a combination of seismic surveys, sediment sampling, and oceanographic measurements. The integration of these data sources provides a comprehensive understanding of the mechanisms governing sediment transport and deposition in deep-sea environments. Visual representation is a crucial aspect of studying these systems. Drawing schematic repre- sentations of contourite systems enhances the ability to describe their characteristics accurately. Understanding these sedimentary environments requires familiarity with key terminologies and the ability to interpret seismic and oceanographic data in a holistic manner. 79 40 Seismic Criteria for Identifying Contourite Drifts and Erosional Elements Seismic criteria play a crucial role in identifying contourite drifts and erosional elements. The resolution of different seismic systems influences the level of detail captured in representing contourite depositional systems. 41 Morphology of Contourite Drifts Previously, the discussion focused on stratigraphy; now, attention shifts to morphology. In seismic imagery, contourite drifts appear as elongated, mounded features along slopes. These features can resemble a snail attached to the slope and exhibit various morphological expressions. In literature, four primary types of contourite drifts are commonly described: Sheeted drifts Mounded elongated drifts Channel-related drifts Confined drifts 41.1 Sheeted Drifts Sheeted drifts occur in deep environments and are characterized by low thickness. They are typically found on the lower continental slope or rise. When present on the continental rise, they are referred to as plastered sheeted drifts, whereas in abyssal plains, they are termed abyssal 80 sheeted drifts. These deposits, while influenced by bottom currents, are also shaped by pelagic settling. Seismic data of sheeted drifts reveal strata that converge towards a subtle moat, which may be difficult to discern. This characteristic stratigraphy is a key identifier of these depositional features. 41.2 Mounded Elongated Drifts Mounded elongated drifts are among the most common types of contourite drifts. These features are distinctly mounded and can be either detached from the margin or separated by a clear moat. Seismic imaging of these drifts displays well-defined strata converging toward the limits of the feature. These drifts vary significantly in size and are often found in deep-sea environments. 41.3 Channel-Related Drifts Channel-related drifts form when bottom water accelerates through a passage or strait. As the water exits the passage and velocity decreases, sediment deposition occurs, often forming a contourite fan. Within channels or gateways, both sheeted and mounded patch drifts may develop. However, the most significant contourite deposits are typically located at the exit of these channels. 41.4 Confined Drifts Confined drifts develop in areas where tectonic activity or seabed irregularities create topographic highs that constrain water flow. This confinement accelerates the current within depressions, leading to sediment deposition. Seismic data of confined drifts often show these features trapped between elevated seafloor structures. 81 42 Additional Types and Influencing Factors Beyond the four main drift types, literature describes additional variations, such as infill drifts, full-controlled drifts, and mixed drift systems. The presence of topographic or tectonic elements can contribute to the distinction of new contourite drift types. Moreover, contourite depositional systems often interact with other geological processes, par- ticularly downslope sediment transport. In some regions, turbidity currents transport sediments from the shelf to deeper environments, interacting with contourite-forming bottom currents. This interaction results in sedimentary bodies that exhibit characteristics of both contourite and turbidite systems, affecting their final configuration. Key Takeaways Understanding contourite depositional systems requires knowledge of their formation mechanisms along continental margins, their representation in seismic data, and their morphological classification. The four primary contourite drift types—sheeted, mounded elon- gated, channel-related, and confined—serve as a fundamental framework for classification, though variations exist due to geological influences. Additionally, contourite systems do not exist in isolation; they are subject to interactions with regional tectonic features and other sedimentary processes, further diversifying their morphol- ogy and stratigraphy. A comprehensive approach, integrating seismic interpretation with water column analyses, remains essential for accurate characterization of these deep-sea depositional environments. 43 Contour Depositional Systems and Bedforms A contour depositional system includes both depositional and erosional elements. Erosional features indicate areas where sediment is removed, while depositional areas contain different types of bedforms, which are small-scale sedimentary structures formed on the seafloor by bottom currents. These bedforms include sedimentary waves, ripples, and dunes. 43.1 Deep-Sea Representation A typical representation of the deep-sea floor includes land, the continental shelf, and the slope leading to the abyss. Water masses and bottom currents influence sediment transport and deposi- tion. High-velocity currents, particularly through straits, contribute to the formation of contour drifts. Other processes, such as turbidity currents, play a role in shaping the continental margin by carving canyons and forming depositional lobes. Arrows in schematic representations indicate the direction of bottom currents and the movement of water masses. Features such as abyssal drifts, separated drifts, and seamounts interact with these processes, modifying sedimentary structures along the continental margin and abyssal plains. 43.2 Definition and Classification of Bedforms Bedforms are morphologies created on the seafloor by bottom currents. A contour drift is considered a large-scale bedform, while the contour depositional system is a broader sedimentary environment that includes multiple types of bedforms. Small-scale bedforms, visible through underwater cameras, include ripples and surface lin- eations. Larger bedforms, detectable via high-resolution bathymetric surveys, include sand waves, dunes, and sediment ribbons. These structures vary in scale from centimeters to kilometers. 82 43.3 Relationship Between Grain Size and Current Velocity Bedform formation depends on sediment grain size and bottom current velocity. A classification system developed by marine geologist Dorrik Stow correlates bedform type with these two factors. Fine sediments are more easily transported, while coarser sediments require stronger currents for mobilization. Bedform morphology depends on the remaining deposited sediment and the velocity of the bottom currents. A bedform velocity matrix represents this relationship, showing the transition between sedimen- tation, transport, and erosion based on current strength and sediment grain size. High velocities are necessary to transport coarse sediments, while lower velocities primarily result in deposition. 43.4 Classification of Bedforms Bedforms are categorized into linear and transverse types: 43.4.1 Linear Bedforms Surface Lineations: Small-scale features occurring on fine sediments, difficult to detect but visible in high-resolution imaging. Grooves and Ridges: Formed by moderate bottom current velocities, with more pronounced relief than lineations. Furrows: Larger-scale bedforms created by strong bottom currents, typically formed by coarser sediment. Crag-and-Tail Structures: Created by obstacles interacting with bottom currents. As currents hit an obstacle, velocity increases, followed by a decrease downstream, leading to sediment deposition forming a tail-like structure. 83 43.4.2 Transverse Bedforms Sand Ribbons: Elongated accumulations of sand shaped by persistent bottom currents. Giant Sediment Waves: Large-scale features, sometimes extending kilometers, formed by interactions between current velocity and sediment supply. 43.5 Understanding Bottom Current Strength Bottom current velocity ranges from weak (centimeters per second) to strong (1-1.5 meters per second). A current of 2 meters per second is considered exceptionally strong. Understanding these velocity ranges helps in interpreting bedform distribution and formation. Data from side-scan sonar and multibeam surveys provide insights into bedform characteristics. For example, furrows and sand ribbons appear as distinct features in sonar imagery, illustrating their structure and distribution. Slides to take into account 43.6 Transverse Bedforms Transverse bedforms differ from linear bedforms in their orientation relative to the bottom current. While linear bedforms align with the current direction, transverse bedforms are positioned perpen- dicular or almost perpendicular to the current flow. These bedforms consist of regular undulations of the seafloor that vary significantly in scale. The key parameters used to describe transverse bedforms include wavelength, amplitude, crest height, symmetry, and planform. Among these, the smallest-scale transverse bedforms are ripples, which form under low-flow velocities over fine to medium sandy substrates. As the current velocity increases, larger bedforms develop, progressing from ripples to dunes and eventually to sand waves and giant sedimentary waves. 84 Dunes, which are a common type of transverse bedform, exhibit wavelengths ranging from 0.6 to 10 meters. However, in some marine environments, larger dunes, sometimes referred to as giant dunes, can be found with wavelengths exceeding 500 meters. Additionally, gravel waves can form in high-velocity environments, where bottom currents reach speeds of approximately 1.5 m/s. Acoustic data, such as side-scan sonar imagery, reveal how sedimentary waves appear on the seafloor. These images often show large-scale bedforms overlaid with smaller features, highlighting the dynamic nature of sediment transport and deposition. 85 43.7 Giant Sediment Waves and Mud Waves Within the category of transverse bedforms, giant sediment waves, also known as mud waves, represent the largest structures. These features are composed primarily of fine sediments such as silt and clay and can attain wavelengths ranging from 0.5 to 10 kilometers, with heights reaching up to 80 meters. These waves are commonly associated with large contourite depositional systems. Seismo-stratigraphic methods provide detailed imagery of giant mud waves, showing their extensive spatial distribution. A key characteristic of these waves is their migration direction, which occurs opposite to the flow direction of bottom currents. This behavior, known as anti-dune migration, is well-documented in sedimentology and also occurs in terrestrial dune systems. The formation and migration of giant sediment waves are directly linked to the action of bottom currents. These currents continuously transport and accumulate sediments, leading to a gradual shift in the position of the sedimentary body over time. This process, referred to as contour drift, underscores the dynamic nature of deep-sea sedimentary environments, where large-scale features are shaped and reshaped by persistent oceanic flows. 43.7.1 Transverse Bedforms and Bottom Current Effects Transverse bedforms are characterized by their orientation, which is perpendicular or almost perpendicular to the direction of the bottom currents. These bedforms exhibit regular undulations on the seafloor, varying significantly in size. The smallest transverse bedforms created by bottom currents are ripples. These form under lower flow velocities, typically over fine to medium sandy substrates. As the current velocity increases, larger bedforms such as dunes develop, with wavelengths ranging from 0.6 to 10 meters. In the marine environment, even larger structures can be found, such as giant dunes and sand waves, which can reach wavelengths of up to 500 meters. In high-energy environments, gravel waves can also form, requiring bottom current velocities of approximately 1.5 m/s. Seafloor imaging techniques, such as side-scan sonar, reveal the complexity of these bedforms, showing the superimposition of smaller bedforms over larger ones. This is evident in sedimentary waves, where giant waves transition into smaller dunes. Among the transverse bedforms, giant sediment waves or mud waves represent the largest 86 features. These structures, formed by fine sediments like silt and clay, can have wavelengths from 0.5 to 10 kilometers and heights up to 80 meters. They are associated with contourite depositional systems and are primarily shaped by bottom currents. A distinctive characteristic of these waves is their migration opposite to the flow direction, a phenomenon known as anti-dune behavior. This behavior is observed both in marine environments and in desert dune systems. The continuous movement of bottom currents results in sediment transport and accumulation, leading to the migration of sedimentary bodies over time. Studies on sedimentary bedforms often involve mapping the seafloor, integrating literature 87 data, direct sediment sampling, and current velocity measurements. Such analyses provide mor- phological maps that classify the seafloor features and depict the influence of bottom currents, as demonstrated in research conducted in the Faroe-Shetland region and the Faroe Bank Channel. Seafloor imagery from high-energy bottom current environments reveals various sedimentary compositions and biological interactions. Examples include coarse sediments with abundant bio- logical colonization, indicating the role of bottom currents in food delivery to deep-sea organisms. Additionally, degraded ripples on muddy sands, fine gravel on low-backscatter sand, and poly- chaete worm tubes on gravel substrates highlight the complexity of sedimentary environments. Notably, filter-feeding organisms tend to settle on the upstream side of boulders, where they can directly capture food particles transported by the currents. These observations underscore the significant impact of bottom currents on sedimentary pro- cesses and benthic ecosystems, shaping the structure and dynamics of the seafloor. 44 Downslope Sedimentary Processes and Submarine Mass Movements Downslope sedimentary processes refer to the transport of large volumes of sediment along conti- nental margins, primarily driven by gravity. These processes include a wide range of submarine mass movements, such as submarine slides and turbidity currents. They play a crucial role in sediment redistribution from shallow continental shelves to deeper environments, including the continental rise and abyssal plain. Submarine mass movements encompass various types of sediment displacement, including rotational slumps, translational slides, debris flows, and turbidity currents. These processes can occur simultaneously or sequentially within a single event, demonstrating complex mechanical behaviors. The nature of each movement depends on factors such as velocity, sediment cohesion, and the ratio between sediment, rock, and water. 44.1 Classification of Submarine Mass Movements The classification of submarine mass movements has traditionally borrowed terminology from terrestrial landslides. However, due to differences in environmental conditions and observation limitations in the submarine realm, the direct application of terrestrial classifications can sometimes lead to confusion. Various authors have attempted to refine classification schemes specifically for submarine mass movements, but the influence of land-based nomenclature remains dominant. Among the most widely used classification systems is that proposed by Masson et al., which distinguishes between slides, debris flows, debris avalanches, and turbidity currents: Slides: Defined as movements of a coherent mass of sediment bounded by distinct failure planes. The sediment moves as a relatively intact unit, undergoing deformation but maintain- ing overall structural coherence. This category includes rotational slides, translational slides, and slumps. Debris Flows: Characterized by a laminar, cohesive flow of clasts within a fine-grained matrix of mud or sand. Water plays a significant role in sediment transport, but the flow remains cohesive. Debris Avalanches: Represent very rapid flows of cohesionless rock fragments, where individual clasts move independently, dissipating energy through grain-to-grain contact. This category also includes rock debris avalanches. 88 Turbidity Currents: Gravity-driven flows in which sediment grains remain suspended within a turbulent fluid. These currents erode, transport, and eventually deposit sediment, creating distinctive sedimentary structures. 44.2 Observational Challenges and Nomenclature Issues Unlike terrestrial landslides, submarine mass movements are typically studied using remote sensing techniques such as geophysical surveys, sub-bottom profilers, and side-scan sonar imagery. Direct observation and sampling are limited, making classification efforts challenging. The terminology used to describe submarine landslides is often imprecise, as different processes can coexist within a single event, leading to complex failure mechanisms. A single submarine mass movement event may involve multiple processes, beginning with sediment failure at the shelf edge and transitioning from slumping to turbidity current transport. Mapping these features on the seafloor reveals varying mechanical behaviors within the displaced sediment, emphasizing the complexity of submarine mass movement dynamics. Despite classification difficulties, some frameworks are more widely accepted in the scientific literature. The system developed by Masson et al. is particularly influential, as it provides clear distinctions between different types of submarine gravity-driven processes based on their mechanical characteristics and sediment behavior. 45 Implications for Seafloor Morphology and Ecology Submarine mass movements significantly impact seafloor morphology, creating features such as slump scars, turbidite deposits, and sediment lobes. These processes also influence benthic ecosystems, as bottom currents associated with sediment transport can affect nutrient distribution and habitat structure. The interaction between bottom currents and sediment transport plays a crucial role in shaping deep-sea environments, with implications for both geological processes and marine life distribution. Continued research using high-resolution geophysical methods and sediment core analysis is essential for improving the understanding of submarine mass movements and their role in shaping continental margins. 46 Submarine Mass Movements and Their Geomorphic Expressions Submarine mass movements can exhibit distinctive geomorphic expressions. Through bathymetric data and imagery, it is possible to recognize and distinguish different types of failures, such as slumps, slides, and debris avalanches. Despite these differences, many common geomorphic features are present across various types of submarine landslides. These include the headscarp, irregular deposits, and lateral scarps. Terminologies may vary to describe the upper, transitional, and lower parts of these mass movements, but their fundamental features remain consistent. 47 Typologies of Submarine Mass Movements Submarine mass movements can be classified into different typologies, including creeping, failures, slides, slumps, rock falls, and rock debris avalanches. These represent significant geohazards, particularly for submarine infrastructure. Additionally, mass movements can be categorized based 89 on their interaction with water, leading to classifications such as water-dominated flows and turbulent flows, including turbidity currents. 48 Characteristics of Submarine Landslides All submarine landslides involve downslope movement of sediment along a basal shear surface. The basal shear surface, also known as the glide plane or slide plane, defines the movement of detached sediment, which can range from minor deformations to intensely folded and brecciated masses. Once a failure occurs, the shear surface may propagate upslope, leading to subsequent mass failures. 49 Creeping Creeping is a slow, gradual, and continuous deformation of seafloor sediments. It can affect various materials, including ice, soil, marine sediments, and rock under gravitational stress. Creeping is a long-term deformation process occurring under constant load on gentle slopes, with deformation progressing at a slow rate. Over time, creeping may lead to eventual failure. Seismic data often reveal creeping activity in sedimentary strata, where shallow deformations are visible while deeper strata remain undisturbed. This suggests that sediment layers are moving downslope, necessitating monitoring to assess long-term stability. 50 Slides and Slumps Slides and slumps involve the movement of large volumes of sediment or rock along a failure surface. These failures typically exhibit distinct morphological features: The head of the slide often shows extensional features such as tension cracks or normal faults. The toe of the failure is characterized by compressional structures, including pressure ridges, folds, and overthrusts. Different types of slides exist, including: Rotational slides: Movement occurs along a curved surface, causing rotational displacement of the sediment. Translational slides: Movement occurs along a relatively planar failure surface, maintaining the original stratigraphic structure with limited deformation. Slumps: These are characterized by high internal deformation, where original stratigraphy becomes highly disturbed. Bathymetric data often reveal the presence of headscarps and the general body of the submarine slide, which can be identified through acoustic turbidity. Tension cracks and small slumps can be detected using side-scan sonar, providing detailed imaging of failure features. 90 51 Lecture 13, 13.12.2024 51.1 Submarine Geomorphic Processes Overview Submarine geomorphic processes include gravity-driven resedimentation mechanisms that transport large amounts of sediment downslope, from the continental shelf to deeper parts of the submarine environment. These mechanisms are known collectively as submarine mass movements. Types of Mass Movements Submarine mass movements can be classified into two main cate- gories: Submarine landslides, which involve the downslope displacement of cohesive sediment masses. Gravity flows, which consist of fluid-like movement of sediment-water mixtures. Creeping Creep refers to the very slow deformation of surface sediments. Unlike landslides or slumps, creeping does not involve a sudden displacement. However, it can still result in visible surface deformation over time. It affects only the uppermost layers of the sediment and progresses gradually. Slides and Slumps A key distinction among mass movements is between slides and slumps. Both involve a cohesive mass of sediment moving downslope along a failure surface, also called a glide or slide surface. Slides Slides (sensu stricto) occur when the sediment mass moves along a relatively planar surface. These movements are typically translational and the ratio between the thickness of the deposit and the distance it travels is relatively low. Slumps Slumps involve rotational movement along a curved failure surface. This type of mass movement is characterized by the internal deformation of the sediment body and the formation of rotational features. The displaced material remains relatively coherent during the movement. Key Concept Both slumps and slides involve large, cohesive masses of sediment moving along identifiable failure surfaces. The distinction lies in the geometry of movement—rotational for slumps, translational for slides—and in the relationship between deposit thickness and displace- ment distance. These classifications are essential for understanding sediment dynamics in the submarine environment. Importance of Acoustic and Morphological Data High-resolution acoustic data such as size- consistent backscattering and multi-beam data are essential in the interpretation of submarine geomorphic features. These datasets allow for detailed visualization and characterization of landforms generated by gravity-driven sedimentary processes. 91 Interpretation Tools Images and graphs obtained through these techniques provide critical morphological details, such as slope breaks, scarps, and displaced sediment masses. They also help in identifying the specific terminology used to describe submarine landslides and associated features. Submarine Spreading Another form of mass movement discussed is submarine spreading. This phenomenon involves the deformation of surficial sediments caused by the development of a weak layer beneath them. Unlike slumps and slides, submarine spreading creates a distinct morphological pattern on the seafloor. Morphological Signature The most recognizable feature of submarine spreading is the for- mation of alternating ridges and troughs on the sediment surface. These structures are oriented approximately perpendicular to the direction of downslope movement. Their geometry results from the extension and compression caused by the spreading of the upper sediment layer. Triggering Mechanisms Submarine spreading is typically triggered by a combination of factors, including seismic loading and a reduction in structural support within subsurface layers. These conditions create instability, allowing the upper cohesive sediments to deform and spread laterally. Global Occurrence Submarine spreading is a widespread and significant style of subma- rine mass movement. Many well-known submarine landslides exhibit features consistent with spreading, particularly those characterized by the ridge-and-trough surface morphology. Despite its unique features, submarine spreading is still considered part of the broader category of cohesive sediment mass movements. Rockfall Within the broader category of submarine mass movements, another type is rockfall. This process involves the failure and downslope movement of consolidated rock material, rather than unconsolidated sediment. However, rockfalls are relatively uncommon in the marine environment, especially in deep marine settings. 92 Environmental Rarity The rarity of rockfall events in submarine environments is mainly due to the widespread presence of sediment covering the seafloor. These sediments can be of lithogenous or biogenic origin, depending on the geographic and environmental context. As a result, exposures of solid rock on the seafloor are infrequent. Typical Settings Although rare, rock outcrops can be found in specific environments: Submarine canyons: The erosional activity within these incised features can expose rocky formations, particularly along the canyon walls and scarps. Active faults: Tectonic activity can uplift and expose rocky surfaces at the seafloor. Carbonate margins: These areas, often formed by biogenic processes, can present lithified carbonate rock, creating potential for rockfall. Characteristics of Rockfall Rockfalls are distinct from other submarine mass movements in that they involve only the displacement of individual or clustered rock blocks, without large volumes of sediment. These blocks may detach and roll or slide downslope. The scale of rockfalls can vary, and in some cases, the exposed rocks may serve as hard substrates for colonization by benthic organisms. Due to the predominance of soft sediments on the seafloor, rockfall represents a less frequent but geologically important form of submarine mass movement. Its occurrence is typically linked to localized geological features that promote rock exposure. Rock Debris Avalanche Rock debris avalanche represents a transitional type of submarine mass movement, falling between the failure of cohesive sediment and the initiation of gravity flows. It is characterized by a high-energy, extremely rapid, and massive flow-like motion of fragmented rock. 93 Characteristics This phenomenon typically involves a heterogeneous mixture of materials: Various grain sizes, from fine sediment to large boulders. Presence of a matrix of water and sediment facilitating movement. Highly chaotic deposits, with no recognizable sedimentary layering. Often lobate in shape, especially in their distal portions. Unlike slumps or slides, the deposits are not merely deformed, but truly chaotic and porous, frequently containing large sediment blocks that can reach sizes from meters to hundreds of meters. These deposits also generate specific flow-related morphologies. Geological Settings Rock debris avalanches occur primarily in environments with: Steep submarine slopes or cliffs. Outcropping of consolidated rock or sediment. Volcanoclastic settings, where alternation of marine sediment and volcanic rocks is common. The most typical environments include: Submarine flanks of volcanic islands. Seamounts with volcanic origin. Regions affected by tectonic or seismic activity. 94 Volcanic Islands as Common Settings Volcanic islands, due to their geological nature, present ideal conditions for rock debris avalanche formation: Alternating layers of fine marine sediment and solidified lava flows. Frequent instability caused by eruptions, earthquakes, or volcanic collapse. High slope gradients and rapid sediment accumulation. When such instabilities occur, they can produce large-scale rock debris avalanches, as seen in several examples: Canary Islands (La Palma, El Hierro, Tenerife): Mapping of the seafloor around these islands reveals widespread blocky features indicative of debris avalanche deposits. High-resolution sonar imagery shows scarps on the island connected to offshore lobate deposits filled with boulders. Ischia Island, Italy: Offshore studies documented a massive debris avalanche deposit. Re- search by Chiocci and co-authors (2006) detailed the morphological features, timing, and interaction with volcanic dynamics using seismic stratigraphy. Seafloor Morphology The terminal parts of these deposits are typically: Marked by distinct boundaries separating deformed and undeformed seafloor. Composed of coarse sediment mixed with lithified boulders. Recognizable in high-resolution seismic profiles by the presence of hyperbolic reflectors representing large blocks. These morphological and sedimentological features help identify and differentiate rock debris avalanche deposits from other submarine mass movement types. Morphological Elements of Submarine Mass Movements After describing the main types of submarine mass movements, it is crucial to recognize their morphological features, which are visible through tools like multibeam bathymetry, backscatter, and seismic stratigraphy. These features help define the geometry, dynamics, and extent of the movements. Key Morphological Zones We can divide the morphological structure of a mass movement into two main zones: Evacuation Area (Source Area): This is where the sediment detaches from the seafloor. – Well-defined headscarps, or escarpments at the upper margin of the failure zone. – Crown cracks (tension cracks) and minor scarps often present around the headscarp. Deposition Area (Failure Deposit): The area where the failed sediment accumulates. – Surface can be regular or irregular. – Irregular surfaces are described as amok surfaces, with: * Fractures and folds. 95 * Blocks of various sizes. * Transversal and longitudinal lineations. * Pressure ridges at the toe of the deposit. – Slide/glide planes are detectable via seismic profiles. Run-out Distance The run-out distance (L) is the horizontal distance between: The upper limit of the main slide scars (start of movement). The most distal downslope end of the deposit. Run-out distance is a key metric to quantify the dynamics of the movement. It often correlates with: – The volume of displaced sediment (longer runout = larger volume). – The degree of disintegration of the failed mass. – The presence of water and fluidization (e.g., turbidity currents or avalanches). Data Representation Multibeam and backscatter imagery are used to identify: – Headscarps. – Lateral scarps. – Main depositional zones. Digital Terrain Models (DTM) and contour line maps are essential tools to visualize these morphologies (as seen in the practical exercises). 96 Summary To properly describe submarine mass movement deposits and their dynamics, it is essential to: Identify evacuation and depositional areas. Recognize morphological markers such as scarps, cracks, and ridges. Measure run-out distances and sediment volumes. Use appropriate geophysical data (multibeam, backscatter, seismostratigraphy). From Mass Movements to Gravity Flows Submarine mass movements are not only limited to sliding or collapsing sediments. A fundamental distinction must be made between cohesive material failures (like slides and avalanches) and flows driven by gravity that involve disaggregated, often fluidized, sediments. Classification Summary Submarine mass movements can be divided into two main categories: 1. Mass Slides (Cohesive Failure Processes): Include: creep, translational slides, rotational slides, spreading, debris avalanches. Involve a significant proportion of cohesive material. Debris avalanches can contain less cohesive material than slumps or slides but are still part of this group. 2. Gravity Flows (True Flows): These are flows where sediment moves in a more fluidized state due to gravity, influenced by pore water pressure. (a) Laminar Flows: Include: debris flows, grain flows, sand flows, mud flows. Movement is orderly, layered (like river water in laminar conditions). 97 Flow type depends on grain size and sediment composition. (b) Turbulent Flows (Turbidity Currents): Highly chaotic and energetic flows. Can be low-density or high-density based on sediment concentration. Responsible for deep-sea sediment transport and large-scale morphological features. Turbidity Currents and Continental Margin Morphology Turbidity currents are especially important for shaping the continental margin: They erode submarine canyons on the slope, carving deep channels. The transported sediment is deposited at the toe of the slope, forming submarine fans. Over time, overlapping submarine fans build the continental rise. Submarine Fans A submarine fan is a sediment body deposited by gravity flows (mainly turbidity currents). Fan shape is generally radial or elongated with low-relief geometries. Sediment stratigraphy within fans often shows stacked lobes, each representing a deposi- tional event. 98 Case Study: Zaire Submarine Fan Located on the passive margin offshore the Zaire estuary (West Africa). Composed of a complex system of channels: – Each channel starts from an erosional zone. – Progressively deposits sediment downstream. Seismic stratigraphy reveals: – Sequences of lobes stacked over time. – Erosion-deposition transitions. – Formation of levees around channels. 52 Glacial Sedimentary Processes: Ice Grounding and Retreating An additional important sedimentary process in marine environments is related to glacial dynamics, particularly during glacial and interglacial periods. This process, known as ice grounding and retreating, involves the erosion, transport, and deposition of sediments controlled by the movement of glaciers, and more specifically, by ice streams. 52.1 Glacial Periods and Ice Coverage During glacial periods, polar regions—especially the entire continental shelf—are covered by thick continental ice sheets. Unlike the thin Arctic sea ice (approximately 3 meters thick and floating), these glacial ice masses are grounded on the seafloor due to the significantly lower sea level during these times. The ice sheet is not static but slowly moves under the influence of gravity. 52.2 Ice Streams and Erosion Within the broader ice sheet, ice streams form as fast-flowing zones or “rivers of ice.” These ice streams are highly erosive due to their velocity and mass. As they flow over the continental shelf and land, they carve out large U-shaped valleys or glacial troughs. These features are typically bounded by high-relief banks where ice is slower or stagnant. At the base of these glacial troughs, the erosive action of the ice stream results in distinct linear features on the seafloor, known as mega-scale glacial lineations (MSGLs). These are formed by the 99 interaction of the basal ice and the underlying sediment, often aided by embedded rock fragments acting as erosive tools. 52.3 Sediment Transport and Deposition Sediment entrained by the ice stream is transported along the base of the glacier. Upon reaching the grounding line—the point at which the ice sheet detaches from the seafloor and begins to float—the sediments are released. This transition often occurs at the edge of the continental shelf. In glacial environments, instead of turbidity currents, sediment accumulation at the slope base results from the melting and sediment release of ice streams. The resulting depositional body is referred to as a trough-mouth fan (TMF). These fans differ from typical submarine fans in several ways: Smaller in size. Different geometry. Composed of a wide variety of grain sizes, including coarse debris. Trough-mouth fans are built from repeated glacial cycles and reflect the delivery of sediments from grounded ice at the shelf edge to deeper marine basins. 52.4 Ice Stream Erosion and Till Deposits When ice streams reach the edge of the continental shelf, they exert intense shear stress on the seafloor, which results in a very strong erosive action. This erosive capacity is a defining character- istic of ice streams and plays a critical role in shaping the glaciated continental margins. At the front of an advancing ice stream, a distinct type of glacial deposit forms: the till. This term broadly refers to sediment deposited directly by glacial action. Till is typically unsorted and 100 structureless, composed of a fine-grained matrix that includes clasts of highly variable sizes and compositions, ranging from gravel to boulders. The powerful erosive action of ice streams can excavate deep continental shelf troughs, some- times exceeding 1,000 meters in depth. These large-scale morphological features are important indicators of past glacial activity and are typically characterized by the presence of mega-scale glacial lineations (MSGLs) at their base. These glacial lineations reflect the direction of ice stream movement and result from the com- bined effects of ice flow and the incorporation of rock debris within the ice, which further scours the underlying substrate. During glacial maxima, when ice streams reach the shelf break, the release of sediments occurs. These sediments are deposited beyond the continental shelf, leading to the formation of trough- mouth fans (TMFs)—large depositional features at the base of the slope, built up by successive pulses of sediment delivery from glacial activity. 52.5 Grounding Line Retreat During Deglaciation At the end of a glacial period, temperatures begin to rise, initiating the melting of glacial ice. As a result, ice starts to retreat. Despite this melting, the glacier continues to flow. This dynamic leads to an accumulation of sediment at the base or limit of the ice stream, known as the grounding line. During the deglaciation phase, the retreat of the ice stream can be traced through a sequence of grounding lines. These sequential formations are evidence of the stepwise retreat of the glacier. As the glacier melts, it may also produce icebergs, which will later influence the seafloor, but this will be discussed separately. The deposits associated with these grounding lines are referred to as grounding zone wedges. They form as the glacier retreats in stages, leaving behind distinct sedimentary structures. When examining the bathymetry of a continental shelf previously occupied by such an ice stream, the presence of these grounding lines and their associated wedges is a consistent feature. This stratigraphy provides valuable insights into past glacial dynamics and the processes governing ice retreat. 52.6 Iceberg Plough Marks and Other Morphological Features on Glacial Shelves Another significant morphological element found on glacial continental shelves is the presence of plough marks. These features, also known as iceberg plough marks, are incisions on the seafloor created by drifting icebergs. When large ice caps or ice streams begin to melt, numerous icebergs 101 are released into the ocean. As they drift—particularly when they begin to detach from the main ice stream—their submerged bases can come into contact with the seafloor, carving out grooves or incisions. The movement of icebergs is primarily influenced by ocean currents and water dynamics. Consequently, the resulting plough marks often exhibit no consistent orientation; they appear in random patterns across the seafloor. In some cases, due to tidal influences, the incisions can form more regular or even circular patterns. These features are distinctive and readily identifiable on the seabed. Plough marks often appear superimposed over older glacial features, such as mega-scale glacial lineations. These lineations form during glaciation when ice streams strongly erode the seafloor. The combination of mega-scale glacial lineations and iceberg plough marks is a typical characteristic of polar continental shelves. For instance, on the Antarctic continental shelf, both features can be observed, sometimes alongside crag-and-tail formations. These latter structures form when resistant parts of the seabed obstruct the ice flow, resulting in erosional and depositional features downstream of the obstacle. A comprehensive visual and descriptive reference for these glacial landforms is provided in the Atlas of Submarine Glacial Landforms, authored by Dowdeswell, Jakobsson, Hogan, Batchelor, and Ottesen. This publication offers detailed imagery and descriptions of landforms shaped by glacial processes in polar marine environments. 52.7 Biogeochemical Processes and Pore Fluid Overpressure After examining gravity-driven resedimentation and ice-related processes shaping the polar regions, attention shifts to biogeochemical processes—those governed by the geochemistry and, in many cases, the biogeochemistry of sediments. These processes contribute to the formation of distinctive geological features and associated landforms. The focus lies on understanding how sediment chemistry affects the seafloor and the early diagenetic transformations that turn unconsolidated sediments into sedimentary rocks. Particular attention is given to pore fluid overpressure and its geological implications, along with the effects of precipitation and dissolution in shaping the seafloor.