PHGY 170 - Winter: Introduction to Cells
Document Details
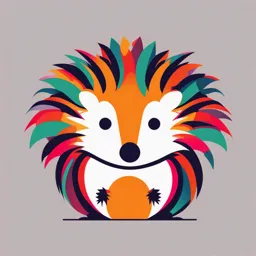
Uploaded by GratefulMercury4168
Tags
Summary
This document provides an introduction to cell biology, including the key components of cells, such as prokaryotic and eukaryotic cells, the roles of various organelles, and cell membranes. It explains how water supports cells, covers lipids, the fluid-mosaic model, and the selective permeability of the cell membrane. It also gives summaries on topics like cellular metabolism, cellular respiration and other sources of cellular energy within the cell, useful for the study of biology for undergraduates.
Full Transcript
January 9th Module 1: Introduction to the Cell and the Central Dogma Introduction to the Cell The Three Tenets of the Cell Theory All living organisms are composed of one or more cells The cell is the basic unit of structure and organization in all organisms All cells come from pre...
January 9th Module 1: Introduction to the Cell and the Central Dogma Introduction to the Cell The Three Tenets of the Cell Theory All living organisms are composed of one or more cells The cell is the basic unit of structure and organization in all organisms All cells come from pre-existing cells Prokaryotic Cells Nucleus: No true nucleus or any membrane bound organelles Cell Size: Smaller cells (approx. 1-5 μm) Uni- or Multicellular: Always unicellular Cell Division: Binary fission Reproduction: Always asexual Examples: Bacteria like E. coli Eukaryotic Cells Nucleus: Has a nucleus and membrane bound organelles Cell Size: Large cells (approx. 10-30 μm) Uni- or Multicellular: Usually multicellular Cell Division: Mitosis/Meiosis Reproduction: Sexual or asexual Examples: Plants and animals The Diversity of Cells Epithelial Cells ○ Form protective barriers in tissues and may be specialized to absorb or secrete specific compounds Muscle Cells ○ Responsible for movement of the skeleton, heart, and many internal organs (ex. stomach). These cells have specialized structures and proteins that allow them to generate motion Nerve Cells ○ Conduct electrical signals throughout the body, control the concentration of muscles, and are responsible for senses including taste, touch, smell, sight, and hearing. Connective Tissue Cells ○ Can create extracellular material that holds cells together in tissue. They may be specialized to absorb or resist external forces (ex. Tendons, vertebral discs) Bone Cells ○ Form the bones of the skeletal system that give strength and support to the body. These cells include osteoclast cells that degrade bone and osteoblast cells that create new bone Secretory Cells ○ Form glands, and as their name implies, secrete substances (ex. Mucus, hormones, enzymes, etc.) Adipose Cells ○ Are located throughout the body to store fat. This fat is in the form of triglycerides which are released when the body is in a period of fasting Red Blood Cells ○ Formed primarily in the bone marrow and released into the circulation where they move and deliver oxygen throughout the body. They do not have nuclei or mitochondria. They have limited lifespans and must be continuously replaced. Introduction to Organelles Peroxisome Breaks down molecules that produce hydrogen peroxide Mitochondrion Produces energy for the cell to use Nucleus Contains the cell’s genetic material and controls access to it Endosome Sorts and condenses content that arrives from outside the cell Plasma Membrane Monitors what can come in and out of the cell and contains organelles Lysosome Breaks down waste and debris Endoplasmic Reticulum (ER) and Golgi Apparatus Carries molecules around the cell and makes proteins and lipids; processes and packages proteins Cytoskeleton Generated motion and stabilizes the cell against deformation The Molecular Building Blocks of the Cell Hydrophilic molecules are attracted to hydrophilic molecules and repel hydrophobic molecules. Hydrophobic molecules are attracted to hydrophobic molecules and repel hydrophilic molecules. For the cell, this means that nonpolar carbon-based structures are attracted to each other and repel water as much as possible. This allows a cell to form membranes and subcellular compartmentalization How Water Supports Cells Polarity The polar properties of water make it an excellent solvent, allowing for the delivery of nutrients and removal of wastes. It also provides an environment that allows cells to exist within a network by facilitating the movement of chemical messengers within and between cells. Specific Heat Capacity The high specific heat capacity of water allows for thermoregulation by acting as a heat sink for the many chemical reactions that occur within cells, as well as the exchange of heat between liquid and air. This is critical for warm blooded organisms, such as humans, that must regulate their body temperature. Lipids Lipids are the building blocks for oils and fats. They are made of hydrocarbon chains and are usually very hydrophobic and therefore insoluble in water. Cholesterol: Regulated cell membrane fluidity and is a biological precursor for compounds such as steroid hormones, bile acids, and certain vitamins. Phospholipids: Are amphipathic (both hydrophobic and hydrophilic in certain sections) and form cell membranes. They have a hydrophilic head and a hydrophobic tail that enables them to form the phospholipid bilayer of cells. Triglycerides: The main component of body fat in animals and are used to store energy. Carbohydrates Monosaccharides Single carbohydrate molecules containing only carbon, hydrogen, and oxygen. Glucose is an example of a monosaccharide, also referred to as sugar. Disaccharides Are two monosaccharides bonded together connected by a glycosidic bond. An example of this is sucrose, which is composed of glucose connected to a fructose. Table sugar is crystallized sucrose. Oligosaccharides Are composed of three to ten monosaccharides linked together. Raffinose is an example of an oligosaccharide. Polysaccharides Are much longer chains, are even more complex, and play many important roles in the cell. An example of this is glycogen. Amino Acids The building blocks of peptides and proteins. They have a consistent structure of an amino group, a central alpha carbon with an R-group, and a carboxylic acid group. Hydrophobic Amino Acids Non-polar Normally found in the core of the protein or interacting with other hydrophobic molecules in the membrane Can be aliphatic (straight or branching chains or non aromatic rings) or aromatic (contains an aromatic ring with a double bond) Charged Hydrophilic Amino Acids Carry a positive or negative charge and are therefore hydrophilic Location of charge is found on the outside of proteins where they can interact with water Polar Amino Acids Polar hydrophilic side chains can form hydrogen bonds that stabilize proteins, more common on the outside of a proteins Aromatic Amino Acids Have ring structures with double bonds that have distinct properties associated with their chemical structure They are very large amino acids and the gain or loss of these amino acids can cause deformities in a protein’s structure. Peptides and Proteins When amino acids are connected together by peptide bonds, they form peptides. Proteins are made up of long chains of amino acids (normally more than 20). These polypeptides fold into a 3D structure that is required for protein function. Proteins include enzymes, receptors, keratin and other structural proteins, and hemoglobin DNA and RNA Structure The basic building blocks of both DNA and RNA are nucleotides. Structure of a Nucleotide Five-Carbon Sugar ○ The 5’ end of the sugar is where the te group is attached in a single nucleotide ○ The 3’ end is where a phosphate group of a different nucleotide can form a bond ○ The five-carbon sugar in RNA contains extra oxygen on the 2’ carbon. DNA on the other hand contains a lone hydrogen on the 2’ carbon and lacks and additional oxygen Phosphate Group ○ Nucleotides generally have one to three phosphates attached to the 5’ carbon. These are high energy bonds and are, in part, why ATP can be used for energy ○ Part of what is called the DNA sugar-phosphate backbone Nitrogenous Base ○ Nitrogen containing compounds are attached to the 1’ carbon of a sugar in a single nucleotide ○ Purines: Have two rings in their structure (Adenine and Guanine) ○ Pyrimidines: Only have one ring in their structure (Cytosine, Thymine, Uracil) From Nucleotides to DNA The sugar-phosphate backbone of DNA is held together by phosphodiester bonds, which are added to the 3’ end of the existing strand. DNA is composed of two strands. Hydrogen bonds between complementary bases on each strand form cross-linkages. The purines in one DNA strand will always base pair with the pyrimidines in the opposing DNA strand. Each strand of DNA is antiparallel to the other, because they run in opposite directions The double-stranded helix structure of DNA is so the nucleotide bases come in contact with as little water as possible due to their hydrophobic nature Introduction to RNA RNA is a nucleic acid similar to DNA It plays a critical role in the synthesis of proteins, as it relays information stored in the nucleus (in the form of DNA) to the cytoplasm where all protein is made. The primary role of RNA in eukaryotic cells is to carry information that tells the cell what kinds of proteins to make How RNA Structurally Differs from DNA In RNA the pyrimidine uracil is used instead of thymine The nucleotides in RNA contain ribose rather than deoxyribose RNA is single stranded and is less stable than DNA RNA: Functions and Types Messenger RNA (mRNA) ○ Carries instructions for making proteins in the cell Transfer RNA (tRNA) ○ Brings amino acids for protein synthesis during translation Ribosomal RNA (rRNA) ○ Make up ribosomes, which are in charge of translating RNA into protein The Central Dogma Part 1 — DNA Replication DNA is the genetic material that can be inherited by offspring. The central dogma of molecular biology is a set of principles that explain how DNA contains the instructions for building RNA and subsequent proteins, which are essential for the structure and function of the cell and the body. Introduction to Genes DNA is divided into functional units known as genes, which contain specific instructions for the synthesis of a functional product, a molecule, needed to perform a job in the cell Typically genes contain information to make a protein, and this is referred to as coding DNA. In eukaryotes these genes are located on chromosomes and are separated by large spaces of DNA called non-coding DNA. These non-coding regions do not correspond to protein production Structure of a Gene Note: Most of a gene is not actually used to make a protein - only a small amount is Exons The sections of a gene that contain the information that is used to make a protein, called coding sequences, or coding DNA Introns Introns are sections of DNA that are not used to make a protein, called non-coding sequences, or non-coding DNA Regulatory Sequences Regulatory sequences control when a gene is turned on, or used. The Central Dogma of Molecular Biology There are three key processes that need to occur for information to be converted from DNA to proteins Replication DNA is replicated (copied) before a cell divides so that both the original cell and the new daughter cells each have complete copies of DNA ○ The major enzyme involved in this process is DNA polymerase Transcription Information from a section of DNA is transcribed (copied) into RNA to transport this information out of the nucleus for protein production ○ The major enzyme involved in this process is RNA polymerase Translation The RNA is read and translated from nucleotides to amino acids to produce proteins that perform a specific function in the cell ○ The major enzyme involved in this process is the ribosome Stages of DNA Replication Every cell contains a complete copy of DNA (ie. genome). Therefore, DNA must be copied in preparation for cell division, so the new cell will have a copy of the genome. DNA replication is semi conservative, meaning that when DNA is replicated, one strand of the parent DNA is used as a template for the daughter DNA. The end result is that in every cell, one strand of DNA is the original parent strand, and the other is the newly synthesized (the parent DNA is “semi” conserved.” Initiation of Replication In this stage, double-stranded DNA needs to be separated into single strands to begin replication. This is initiated at regions in the DNA called the origin of replication (Orc) with the help of special proteins Protein Binding ○ A group of proteins binds to the origin of replication to begin replication, the most important being DNA helicase DNA Unwinding ○ DNA helicase unwinds DNA into two single strands, forming a structure that is called the replication fork. This is where the rest of replication occurs. As helicase moves down the DNA strand, so does the replication fork. This step requires energy RNA Primers ○ Following unwinding, the exposed single strands of DNA can be copied. Each single strand will act as a template from synthesis of a new complementary strand. The enzyme that accomplishes this is DNA polymerase. However, it needs several existing nucleotides at the beginning of the complementary strand to begin adding nucleotides with phosphodiester bonds. The solution to this is the enzyme primase, which adds a short number of RNA nucleotides at the beginning of the strand, called an RNA primer. Elongation of Replication Now that the RNA primers have been added, the elongation stage of DNA replication begins. The primers are elongated by the enzyme DNA polymerase. Direction ○ DNA polymerase can only add nucleotides to the 3’ end of a DNA strand, and therefore can only move along the parent strand of DNA in the 3’ to 5’ direction, creating a new strand that is in the 5’ to 3’ orientation. Note: the DNA polymerase on both parent strands move in opposite directions Catalysis ○ DNA polymerase catalyzes the new phosphodiester bonds between an incoming nucleotide and the existing nucleotide on the backbone. ○ It also ensures that the incoming nucleotide is the correct base pair match to the parent strand Elongation of the Leading vs Lagging Strand DNA polymerase synthesizes in the 5’ to 3’ direction. As a result, one new strand is made as a continuous piece, called the leading strand, and the other is made in small pieces, called the lagging strand. Leading Strand ○ Runs in the 3’ to 5’ direction along the parent strand ○ DNA polymerase creates a new strand that is in the 5’ to 3’ orientation, in the same direction that the replication fork is moving, thus the synthesis is continuous ○ The leading strand can be elongated from one RNA primer alone Lagging Strand ○ Built in the 5’ to 3’ direction running away from the replication fork, and because DNA polymerase can only build continuously in the 3’ to 5’ direction, this strand has to be made in fragments. ○ As DNA polymerase moves away from the fork, it must repeatedly release and reattach. Each time DNA polymerase reattaches, it needs primase to make a new RNA primer, thereby creating a new DNA fragment These fragments are called Okazaki fragments ○ To mitigate potential damage to the lagging strand while it is not yet being replicated, single stranded binding proteins (SSBPs) bind to the single stranded DNA. However, they cannot ward off damage indefinitely, and the DNA still needs to be synthesized as soon as possible. Okazaki Fragments In order to ensure the final DNA molecule does not contain any RNA primers, an enzyme goes through and replaces the RNA primers in both the leading and lagging strands with DNA. However, these new fragments of DNA, where RNA primers used to be, are not connected to any other fragments ○ An enzyme called ligase resolves this issue Ligase To remedy this issue of gaps in the DNA, an enzyme called ligase goes through the new strand of DNA and catalyzes the phosphodiester bonds. By sealing these gaps in the DNA backbone, ligase joins the Okazaki fragments on the lagging strand. Once all the RNA primers have been replaced with DNA and ligase has joined the backbones of the Okazaki fragments, the new DNA double helices are almost complete Termination of DNA Replication Once the replication fork reaches the end of a DNA molecule, there will be a stretch of DNA on the lagging strand that cannot be replicated DNA polymerase requires an RNA primer. However, at the end of the lagging strand, there is not enough space for primase to add an RNA primer. As such, DNA polymerase cannot start DNA synthesis. This results in a single stranded stretch of uncopied DNA known as an overhang Overhang: Shortening of Chromosomes If the overhang on the lagging strand were to be left as it is, there would be a very small region of the parent (lagging) strand DNA that is left unreplicated and unpaired (the 3’ overhand). This overhang would be degraded, since single strand DNA is very unstable Therefore, after every cycle of DNA replication, there would be a gradual loss of DNA at this end, causing chromosomes to shrink over time, which would be very damaging to a cell. However, the cell has a mechanism in place to prevent the loss of DNA Telomeres and Telomerase In order to prevent the shortening of chromosomes, the cell has structures that extend the ends of DNA. Telomeres are long, non-coding sections of DNA that are added to the ends of each chromosome. Since they are non-functional, they can be degraded over cell cycles without affecting cell function Telomerase The enzyme that adds telomeres is called telomerase. It is quite different from the other enzymes involved in DNA replication. It carries a short piece of RNA within the enzyme that binds to the 3’ overhand and extends past the DNA. RNA Template Telomerase is an RNA-dependent DNA polymerase, so it can make DNA using RNA as a template The RNA carried by the telomerase is then used as a template to add a corresponding DNA strand to this extension Overhang There is now an extra long 3’ overhang that contains repetitive, non-functional DNA. An RNA primer is added, and DNA polymerase adds DNA like usual until there is not enough of a 3’ lead for the enzyme to proceed Chromosome Lengthening Telomerase repeats this process several thousand times, creating the long ends of the chromosomes Now, the normal process of DNA replication at the lagging strand can re-commence. Primase lays down an RNA primer which is extended by DNA polymerase DNA Replication Review 1. DNA helicase unwinds the DNA 2. RNA primase adds a primer 3. DNA polymerase begins synthesizing the leading and lagging strands 4. Primase continues to add new RNA primers to the lagging strand 5. DNA polymerase next synthesizes DNA on the lagging strand on new Okazaki fragments 6. An enzyme replaced the RNA primers with DNA 7. Ligase catalyzes phosphodiester bonds forming the backbone of DNA The Central Dogma Part 2 — Transcription Transcription is the process by which information is rewritten. In physiology, transcription refers to rewriting the DNA sequence of a gene into the analogous RNA sequence. Important Proteins in Transcription RNA Polymerase ○ RNA Polymerase I: Responsible for synthesizing most of the rRNA required for a functional ribosome ○ RNA Polymerase II: Synthesizes messenger RNA (mRNA) ○ RNA Polymerase III: Synthesizes transfer RNA (tRNA), as well as some other RNA molecules Transcription Factors ○ A transcription factor is a group of proteins that binds to specific DNA sequences, and by doing so controls the rate of transcription from DNA to RNA ○ Transcription factors bind to regulatory regions of a gene and signal to transcriptional machinery, including RNA polymerase, which genes need to be transcribed. Transcription factors can promote (as an activator) or block (as a repressor) the transcription of genes by altering the ability of RNA polymerase to start transcription Stage 1: Initiation of Transcription Initiation of transcription begins with the binding of transcription factors to a specific DNA sequence. This sequence is a regulatory region of DNA located upstream to the beginning of the gene ○ The regulatory region controls whether a gene is turned on or off Once this happens, RNA polymerase II can bind to the DNA of the gene, attaching to a specific location called the promoter The promoter region is the “start site” for transcription and usually contains the nucleotide sequence known as the TATA box Stage 1: The Transcription Complex The binding of transcription factors facilitates transcription by three mechanisms ○ Guiding RNA Polymerase II to the Correct Strand ○ Unwinding DNA ○ Phosphorylating RNA Polymerase II Stage 2: Elongation of Transcription The moving of the transcription complex forward is the second stage of transcription, and as the RNA polymerase moves forward, it synthesizes the mRNA molecule. This process is called elongation. RNA polymerase II adds nucleotides to the 3’ end of the growing RNA strand. As RNA polymerase moves forward, it extends the mRNA molecule in the 5’ to 3’ direction while reading the DNA template strand. The region of unwound DNA is called the transcription bubble and is covered by the RNA polymerase II to protect the unwound single-stranded DNA from damage Stage 3: Termination of Transcription Proteins can bind to RNA polymerase II to induce termination but this process is not well understood. Transcription normally ends when the RNA is cleaved from the RNA polymerase II by a separate enzyme that is well past the coding sequence of the gene. At this point the transcription bubble collapses, the RNA molecule dissociates from the template, and the RNA polymerase II detaches from the DNA. Unlike DNA polymerase, RNA polymerase II does not have any proofreading function, and has a much lower fidelity. To compensate for this, it will make several short RNA molecules until one has the proper complementary hydrogen bonds to match the DNA template strand to ensure that a correct mRNA molecule is made. Post-Transcriptional RNA Processing RNA is designed to be short-lived in the cell, since the changing demands of the cell need different proteins to be made at different times. However, the cell needs a way to keep the newly made mRNA around long enough to produce the proteins it needs. To preserve the newly produced mRNA molecule, make it functional, and deliver it to its final location, some modifications are made after transcription. These modifications, termed post-transcriptional RNA processing, begin almost immediately after its synthesis RNA Modification: 5’ Methylguanosine Cap The first modification is the methylated 5’ cap on the mRNA synthesis to protect the mRNA molecule from premature degradation by nucleases, which degrade nucleotides. Guanosine Triphosphate A guanosine triphosphate (GTP) is added to the 5’ end of the mRNA via an unusual 5’ to 5’ triphosphate linkage Methylation Immediately after capping, this GTP has a methyl group added to the 7 position of the guanine base RNA Modification: 3’ Polyadenylation After the 5’ methylguanosine cap is added, two modifications occur in the same time period. The first modification is 3’ polyadenylation A different type of polymerase called poly(A) polymerase adds around 200 adenosines to the 3’ end of the mRNA immediately after it is cut from the RNA pol II. This creates a structure called a poly(A) tail The poly(A) tail is essential for binding proteins that are necessary to transport the mRNA out of the nucleus and to start the process of translation RNA Modification: RNA Splicing The third modification that occurs simultaneously with the poly(A) tail is RNA splicing Introns do not correspond to protein production. Therefore, they need to be removed from RNA before it is translated into a protein, which occurs through a process termed splicing RNA Modification: Splicing and Alternative Splicing Some genes will use alternate splicing to create multiple mRNA molecules from a single gene. This can cause exons to be skipped. Most human genes can produce at least two different mRNAs through splicing. Scientists still do not know if or when my of these alternate proteins are made Transport through the Nuclear Pore Complex RNA transcription occurs in the nucleus, since that is where DNA is stored. Once post-transcriptional modifications are completed, the mRNA needs to exit the nucleus to be translated into protein in the cytoplasm A complex of proteins bind to the mRNA to assist in its transport out of the nucleus through a nuclear pore. Some of these proteins will be recycled and transported back into the nucleus and others will help the mRNA in the process of translation. However, the mRNA will not go back into the nucleus, but rather remain in the cytoplasm of the cell. Exceptions to the Central Dogma RNA (not DNA) is used by some viruses as their genetic material, like the Hepatitis C virus. It has specialized enzymes like an RNA polymerase that reads and makes RNA, and RNA helicase which is designed to unwind double-stranded RNA. ○ Neither of these functions naturally occur in human cells. DNA Replication Review Location Nucleus Product Replication of a cell’s entire DNA Direction of Synthesis Replication in both direction with Okazaki fragments Proofreading DNA is proofread in replication and DNA repair happens constantly When it Occurs All DNA is replicated in preparation for cell division Polymerase Involved DNA polymerase RNA Transcription Review Location Nucleus Product RNA made from one or a few genes Direction of Synthesis Single direction transcription in tightly regulated areas of genes Proofreading RNA is not proofread. RNA pol II makes several starts until a matching RNA is generated When it Occurs Specific to cell type, cellular conditions, timing, transcription factors, and gene-specific promoters Polymerase Involved RNA polymerase II The Central Dogma Part 3 — Translation Translation is the third and final step in the central dogma processes. It involves taking the mRNA transcript of a gene and converting it into a functional protein. From Nucleotides to Amino Acids mRNA Cells decode mRNA by reading their nucleotides in groups of three, called codons Amino Acids Each codon corresponds to a specific amino acid. Some codons do not specify and amino acid, while other codons code for the same amino acid Codons It is important that codons are three nucleotides long, as it gives the cell enough possible combinations of nucleotides to account for each of the 20 amino acids in our genetic code. In fact, it actually allows enough different combinations of the four nucleotides to have multiple codons coding for the same amino acid The Standard Genetic Code A single codon can only code for one amino acid, but several codons can code for the same amino acid. Stop Codons UGA UAG UAA Start Codons AUG Codon Redundancies Amino acids that have only a single possible codon are less common, while amino acids with multiple possible codon arrangements are the ones most commonly found in proteins The Components of Translation mRNA mRNA is delivered, along with some protein factors, from the nucleus to the cytoplasm Depending on the starting sequence of the mRNA, it will either stay in the cytoplasm, or it will be directed to the endoplasmic reticulum Protein Factors Translation is initiated by initiation factors that bind to the mRNA, including the methyl-guanosine cap on the 5’ end of the mRNA and the poly(A) tail ○ These factors help the small ribosomal subunit identify the initiation site Elongation factors (EFs) are proteins that assist in elongation ○ Some EFs form complexes to deliver the tRNAs and the GTP energy source to the ribosome tRNA The job of tRNA is to deliver the correct amino acid to a growing peptide. Each tRNA will recognize a codon by containing a complementary sequence to the codon, termed the anticodon. Note that the bond holding the amino acid to the tRNA provides the energy to make the new peptide bond on the growing amino acid chain Ribosome The ribosome is composed of a large and small subunit, both make of rRNA The small subunit is responsible for binding to mRNA The large subunit has three important sites: the A site, P site, and E site Stage 1: Initiation of Translation Translation is initiated when the ribosome assembles around the mRNA. This requires the initiation factors to help the small ribosomal subunit find the correct AUG site (start codon) to begin the translation of mRNA into protein. Initiation Factors Bind to the mRNA molecule, including the 5’ cap binding factors, the poly(A) binding protein (PABP), and another initiation factor binds to the mRNA 3’ poly(A) tail. The small ribosomal subunit complex attaches to the mRNA molecule at the 5’ end near the methyl-guanosine cap guided by the initiation factors. The small ribosomal subunit attaches to the initiator tRNA, which carries the amino acid methionine Start Codon The small ribosomal subunit and initiator tRNA then crawls forward until the correct AUG sequence is found (start codon) The initiator tRNA then binds to the start codon Large Subunit Once the initiator tRNA is bound to the start codon, the large ribosomal subunit encloses the mRNA, with the initiator tRNA in the P site. The formation of the complex of the small and large ribosomal subunits completes the initiation of translation Stage 2: Elongation of Translation Elongation is a multiple step cycle in translation, and moves forward as a continuous loop. Each cycle adds one amino acid to the growing chain of amino acids—the peptide. This process requires energy via the consumption of GTP tRNA Binding The A site of the ribosome is where aminoacyl tRNAs first attach to the ribosome. The new tRNA is charged with GTP and will have an anticodon complementary to the A site codon Peptide Bond Formation The GTP on the aminoacyl tRNA in the A site is converted to GDP, and peptidyl transferase, an enzyme within the large ribosomal subunit, moves the growing peptide in the P site onto the tRNA amino acid in the A site. The P site is where the amino acid chain is removed from the tRNA and added to the next amino acid by a peptide bond in the A site. Translocation The ribosome next translocates down one codon on the mRNA, moving the mRNA and tRNAs down from the A and P sites to the P and E sites. This also requires GTP. The E site is where spent tRNA is ejected from the ribosome Stage 3: Termination of Translocation The growing peptide chain ends when a stop codon is reached (UAA, UAG, UGA). Unlike the other codons, stop codons are not connected to a tRNA molecule. Rather, they attract complimentary release factors, which also fit into the A site of the ribosome. The release factor substitutes water for the amino acid to attach to the peptide in the P site, which leads to the production of a carboxylic acid and releases the peptide. After the peptide is released, a ribosome release factor occupies the A site. This results in the release of the large and small ribosome subunits from the mRNA which can be recycled for more translation. Introduction to Mutations and DNA Repair It is estimated that approximately six errors occur every time the cell replicates. DNA can also be damaged by other factors like ultraviolet light, oxidation, or DNA strand breaks ○ When DNA damage occurs, the result is a mutation in the DNA. A mutation is any change in the DNA structure which results in a variant form that can be passed on to subsequent generations DNA Repair Repair During DNA Replication DNA polymerase has a proofreading function and can check to ensure no mistakes have been made Repair Throughout the Cell Cycle DNA repair proteins are continually scanning DNA for errors and making repairs. Some check immediately after replication, fixing the newly synthesized strand of DNA, whereas others check at different points throughout the cell cycle What Happens if DNA is not Repaired? The DNA repair processes in the cell are important because DNA contains all the information that cells inherit from their ancestors. DNA damage could remove or change some of this information, reducing a cell’s ability to perform vital functions Mutations A mutation is any change in the DNA sequence that results in a variant form that can be passed on to subsequent daughter cells. Changes to DNA will directly change RNA. How these changes impact protein is dependent on if mutations change the way that codons are read and the subsequent amino acids are altered that make up a protein. Types of Mutations Point Mutations Only a single nucleotide is changed, resulting in one of three outcomes: ○ Silent mutation: the amino acid does not change ○ Missense mutation: the amino acid does change ○ Nonsense mutation: the amino acid is replaced by a stop codon, ending translation and preventing the production of the rest of the amino acid Insertion An extra base pair is added to DNA Shifts the 3-base pair reading frame down by one, which can alter every amino acid produced This is a type of frameshift mutation Deletion A base pair or more is removed from the DNA sequence Alters the reading frame if deletion is not in multiples of three This is another form of frameshift mutation Large Scale Deletion, Insertion, Recombination Multiple deletions, insertions, or recombinations of nucleotides can involve entire chromosomes or just parts of chromosomes These changes are often lethal Many cancers are caused by the production of fusion genes (when two genes are combined together), creating new combination proteins with new functions Amino Acid Charges and the Genetic Code Similarly charged amino acids cluster around each other in the genetic code table. If a mutation occurs, this is a mechanism to reduce or prevent it from changing protein structure and function, since amino acids in a group have similar properties. Module 2: The Nucleus and Endomembrane System The Nucleus The primary function of the nucleus is to protect the cell’s DNA ○ This is necessary as DNA stores all the information needed for a cell to survive Ways the nucleus isolates itself from the rest of the cell ○ The Nuclear Envelope: a double layered membrane ○ Having very selective nuclear pores ○ Having a unique fluid called the nucleoplasm Function of the Nucleus In order to protect the DNA, the nucleus must accomplish the following three tasks ○ Allow DNA to be replicated and transcribed into mRNA ○ Regulate what molecules can access the DNA ○ Keep the DNA organized (DNA is fragile and easily damaged) Components of the Nucleus Nuclear Envelope ○ Controls what molecules have access to the nucleus and separates the DNA from the rest of the cell Chromatin & Chromosomes ○ How strands of DNA are organized Stored in chromatin and make up chromosomes Nucleolus ○ Creates rRNA and assembles them into the ribosomal subunits needed to translate proteins Nucleoplasm & Nuclear Matrix ○ A viscous fluid enclosed in the nuclear envelope which suspends nuclear contents ○ The Nuclear Matrix organizes chromosomes and maintains the structure of the nucleus Nuclear Pores ○ Regulate molecular traffic in and out of the nucleus ○ Small molecules like water and oxygen can pass through freely Levels of DNA Packaging Level 1: DNA Double Helix Level 2: Nucleosomes (Beads on a String) Level 3: Chromatin Fibre Level 4: Chromatin Looped Domains Level 5: Heterochromatin Level 2: Nucleosomes (“Beads on a String”) DNA is wrapped twice around proteins called histones, which shortens the DNA seven-fold The DNA wrapped histones are called nucleosomes The nucleosomes are separated by linker-DNA to form the “beads on a string” structure Level 3: Chromatin Fibre The string of nucleosomes are coiled into a spiraling fibre called a ‘chromatic fiber’ ○ Shortens the DNA 42-fold Level 4: Chromatin Looped Domains The chromatin fibre is then looped ○ Shortens the DNA 750-fold Level 5: Heterochromatin The twisted looped domains are further compressed and the heterochromatin is hyper condensed DNA ○ Present in the inactive regions of chromosome (interphase) Heterochromatin is further condensed into entire chromosomes when cells are undergoing cell division Euchromatin vs Heterochromatin Levels 1 - 4 of DNA packaging are called euchromatin ○ DNA is active during these levels and can be easily accessed by proteins which replicate the chromosomes and read DNA to make RNA Level 5 of DNA organization is called heterochromatin and DNA is condensed beyond loop domains and is essentially inactive The Endomembrane System The linked machinery that processes the transport of proteins, lipids, and other macromolecules throughout the cell Exocytosis moves this cargo out of the cell via the exocytic pathway Endocytosis moves this cargo into the cell via the endocytic pathway Overview of the Endomembrane System Nucleus Connected to the ER cisternae by the outer layer of the nuclear envelope Some nuclear pores connect to the ER membranes so molecules can easily pass through RER Dotted with ribosomes and is the site of protein translation SER Responsible for lipid processing, carbohydrate metabolism, and calcium storage Transport Vesicles Help move proteins made in the ER to the Golgi Apparatus for further modification Golgi Apparatus Involved in both protein modification and transport Labels proteins and other molecules (imagine bar codes or postal codes) to direct them to their final destination within the cell The Endoplasmic Reticulum Made up of organized flattened disks of membrane called cisternae ○ The cisternae are connected to the outer nuclear envelope Rough Endoplasmic Reticulum (RER) Studded with ribosomes Ribosomes assemble here to produce proteins, but they can also be found floating in the cytosol. These ribosomes only produce proteins that stay in the cytosol Smooth Endoplasmic Reticulum (SER) Lipid Synthesis ○ Such as phospholipids and steroids Carbohydrate Metabolism ○ Includes converting glucose-6-phosphate to glucose Calcium Regulation ○ Regulates calcium ion concentration in muscle cells which is important for muscle contraction Vesicle-Mediated Transport Transport vesicles shuttle material between organelles using vesicle-mediated transport Small vesicles bud off from lipid membranes of organelles to transport cargo such as soluble or membrane-bound proteins After reaching their destination, they fuse with other lipid membranes to deposit their cargo Golgi Networks: Cis, Medial, and Trans Cis Golgi Network Receives proteins from the ER that have entered the endomembrane pathways Medial Golgi Network Oligosaccharide sugar groups are added to proteins Trans Golgi Network Performs the final packaging to send material to different organelles Trans Golgi Network 1) Other Organelles Depending on the type of cell, different organelles require different combinations of proteins 2) Retrograde Transport Some proteins that have arrived from the ER have a retention signal, meaning that they are packed up with COPI coating proteins and recycled back to the ER 3) The Lysosome A potential modification in the medial Golgi network is the addition of M6P sugar. In the trans Golgi network, these tags are recognized and proteins containing them are send to the lysosome where the cell degrades proteins, DNA, lipids, and sugars 4) Cargo Transport Exocytosis allows vesicles to be transported outside of the cell Endosomes, Lysosomes, and Peroxisomes Endosomes Hold content coming into the cell from the extracellular space via endocytosis Sort and send cargo to their correct final locations Lysosomes Responsible for the break down of proteins, lipids, and sugars Involved in waste disposal Peroxisomes Also break down molecules, but specifically those that generate hydrogen peroxide Neutralize hydrogen peroxide to prevent cell damage Endomembrane System Organelles Overview Endoplasmic Reticulum Acts as a highway and warehouse Involved in protein translation and production of membrane phospholipids Transport Vesicles Transport materials between endomembrane organelles Endosomes Involved in endocytosis to bring materials into the cell Golgi Apparatus Acts like a post office Labels and sorts proteins to direct them towards their final destination Lysosome Involved in waste disposal and macromolecule breakdown Peroxisome Involved in breakdown of molecules that generate hydrogen peroxide Prevents cell damage Targeting Proteins in the Cell To facilitate proteins carrying out their specific functions, they must be moved to various areas within the cell. Unique signal-sequences are used to target proteins to these different areas Proteins Targeted to the Endomembrane System Are tagged within the endomembrane system Eventually end up in various other areas of the cell depending on how they are tagged Proteins Destined for the Cytosol Lack signal peptides as free floating ribosomes will translate them in the cytosol Proteins That Need to be Secreted From the Cell Have a distinct signal sequence that are specific for the cargo in the vesicles These proteins can be produced by cells and are essential for bodily functions such as hormones, or the proteins that form the extracellular matrix Translocation Into the ER Signal Sequence Transport begins with the presence of a signal that is translated as part of the protein SRP Binding When this sequence emerges from the ribosome, it interacts with a receptor (SRP) which binds to the ribosome translating the protein This process pauses translation Ribosome Docking During the pause in translation, the ribosome docks onto the ER membrane This is accomplished by the SRP interacting with an SRP receptor and a complex called the translocon This facilitates the translocation of the growing protein into the ER Translocation Once the protein has translocated into the ER, the signal peptide is cleaved off the protein, and the protein folds inside the ER lumen Embedding Into the Lipid Membrane Transmembrane Domain The translocon for transmembrane proteins recognizes a signal (the transmembrane signal anchor sequences) which initiates the process for the protein to become embedded in the lipid bilayer Protein Enters Lipid Bilayer The transmembrane signal becomes embedded in the lipid bilayer and will be the transmembrane domain of the protein once it is functional and mature Post-Translational Modifications of Proteins by the ER These modifications occur after the proteins are in the lumen of the ER and folded ○ The addition of proteins, sugars, lipids, and new functional groups ○ The cutting of the peptide bonds in the protein ○ The removal of the ER signal sequence Modifications of Proteins by the ER The following are two important enzymes involved in protein folding within the ER Protein Disulphide Isomerase Help to form disulphide bonds between thiol groups in the side chains of cysteines These disulphide bonds help the protein to fold properly and stabilize it Binding Protein Help fold the polypeptide by binding hydrophobic patches in recently translated proteins BiP brings these patches together to fold them correctly, creating the hydrophobic protein interior Peptide Bonds Formation of Peptide Bonds The carboxyl group of one amino acid reacts with the amino group of another amino acid. This results in the formation of a peptide bond Rotation of Amino Acids Once bonded, amino acids can rotate around the formed peptide bond, but the bond itself is a rigid plane Ends of Amino Acid Chains There will be two distinct ends in the long chain of amino acids, one with the amino group free (amino terminus), and one with the carboxylic acid group free (carboxy terminus) Protein Structure Primary Linear peptide sequence Secondary Alpha Helix ○ A tight coil that forms hydrogen bonds between the backbones of every fourth amino acid Beta Sheets ○ Are planes formed between rows of amino acids with hydrogen bonds between the backbones ○ Can be parallel or antiparallel Tertiary The 3D structure of a complete protein Molecular chaperones are required to ensure proper shape during folding Quaternary When multiple proteins are assembled into a complex The individual proteins are called subunits of a quaternary structure if they cannot have a function outside of the complex Domains A domain is the basic building block of a protein structure, and some have clearly defined functions, such as in the case of enzymes ○ They are made up of multiple secondary structure units It is a discrete functional unit and is assumed to fold independently from the rest of the protein and maintain its own function How Structure Affects Function A change in the structure or shape of the protein will change how that protein works Types of Protein Changes Covalent Modifications Relatively long lasting Includes disulfide bonds and the addition of lipids or sugar structures Non Covalent Modifications Relatively short lived Can include proteins interacting with each other in binding sites or small molecules like oxygen, calcium, or magnesium binding transiently Protein Transportation From the ER Getting a protein into the ER and having it properly folded is the first step into moving it through the endomembrane system as cargo However, to move this cargo to another organelle, a vesicle is required Vesicles are made from the same lipid membranes that make up organelle membranes, meaning they are easily able to embed themselves into the membranes of their destination organelles in order to release their cargo Vesicle Mediated Transport — Cargo Selection Signal sequences and receptors on the membrane gather the cargo to the section of the membrane which will be turned into a vesicle Allows for greater efficiency as cargo is accurately transported to the correct location Vesicle Mediated Transport — Coat Proteins Bind to the area on the membrane which will become the outside of the vesicle COPII Used by the ER to shuttle to the golgi COPI Coats vesicles that carry proteins that need to be returned to the ER Clathrin Used by the golgi to form vesicles for exocytosis, endocytosis, and transport to the endosome. Shuttles vesicles between the trans golgi network and other destinations Vesicle Mediated Transport — Budding Coat proteins interact with proteins called ‘cytosolic adaptor proteins’ in order to form a mesh-like network that acts like a basket to pull the membrane into a bulging shape that will ultimately form the vesicle Vesicle Mediated Transport — Scission Proteins pinch the newly formed vesicle off the membrane Dynamin is the primary protein that fulfills this task to release the budding vesicle into the cytoplasm Vesicle Mediated Transport — Uncoating After the vesicle has been successfully created, the coat and adaptor proteins are disassembled After these proteins are uncoated, they can be recycled in the formation of another vesicle Vesicle Mediated Transport — Transport Motor proteins attach to the vesicle within the cytosol to help move it through the cell, moving along the microtubules of the cytoskeleton This is how the cell controls the direction and destination for each vesicle Vesicle Mediated Transport — Tethering and Docking Once the vesicle has reached its destination, tether proteins attach a receptor on the acceptor protein ○ This allows the vesicle to get close enough the find the correct acceptor target proteins Once the target proteins are found, the vesicle docks on the surface, allowing the vesicle to fuse to the acceptor membrane Vesicle Mediated Transport — Fusion The process of the lipid membrane becoming continuous with the acceptor protein Occurs via pairs of v-SNARES and t-SNARES interacting This process allows the cargo within the vesicle to be transferred into the acceptor organelle Vesicle Mediated Transport — Disassembly The remaining tether and fusion proteins are disassembled and recycled into the initial organelle Stages of Exocytosis Exocytosis is the process of the moving of cargo out of the cell. 1. Proteins are translated in the ER 2. These proteins undergo post-translational modifications and protein folding in the ER 3. Move between the ER and Golgi apparatus via vesicle-mediated transport 4. The Golgi apparatus causes the protein to undergo further modifications 5. Cargo can be secreted Regulation of Exocytosis in the Cell Constitutive Secretion An uncontrolled process which is always happening Ex. extracellular matrix proteins such as collagen Regulated Secretion Requires a signal to stimulate the cell Often have specialized storage granules that are released in response to these signals Ex. neurotransmitters in neurons and insulin from the pancreas Endocytosis: Import of Cargo Once cargo is brought into the cell via endocytosis, it is taken to the endosomes and often the lysosomes Endosomes sort cargo, and if cargo is still within the endosomes during the late stages it is degraded when the endosome matures into a lysosome Formation of the Endosome Clathrin is the coat protein involved in vesicle formation during endocytosis ○ Initiates the process of triggering the plasma membrane to form the vesicle that will take cargo to the early endosome The clathrin coat will be disassembled by cytosolic proteins that cause the vesicle to transport to its location and fuse with the early endosome The Early Endosome Made up of vesicles from the plasma membrane and vesicles from the trans golgi network Endocytic vesicles containing cargo and cargo receptors form the plasma membrane Vesicles which contain M6P receptors fuse with the endocytic vesicle, forming the early endosome ○ Proton pumps begin acidifying the endosome lumen The slight acidity of the early endosome causes the cargo receptors and M6P receptors to change shape and release their cargo Storage Granules Some cells will take in cargo and store it for later Across the Cell Some cells have distinctive functions for different sides of the cell, and will transport cargo across the cell for these functions Into the Cell Endocytosis can be a method to take in signalling molecules that will have a downstream function in the cell, such as turning on gene transcription Endocytosis of a receptor can also be a way to turn off these proteins The Late Endosome Lowered pH Proton pumps present on the vesicles arriving from the trans Golgi network lower the pH of the interior of the endosome Recycling A continuous recycling of receptors and cargo is performed Cargo Degradation Cargo is ultimately degraded as pH continues to drop, causing proteins to unfold This process provides essential building blocks such as sugar and amino acid for repurposing The Lysosome As the pH becomes more acidic, enzymes such as proteases are activated, which causes the late endosome to convert into the lysosome A protective glycolax layer of LIMPs and glycosylated proteins forms to prevent the lysosome from degrading itself The Exocytic Pathway and Proteins Overview 1. The signal sequence targets mRNA to a ribosome on the ER and translation starts 2. In the ER, proteins fold and the proteins are modified 3. Transport vesicles take folded proteins from the ER to the cis Golgi network 4. In the cis Golgi network, proteins are transferred to the medial Golgi Network where they are modified further and moved forward through the trans Golgi network 5. Once the proteins reach the trans Golgi network, they are put into new transport vesicles and transported to the plasma membrane 6. Proteins are released into the extracellular space via exocytosis Module 3: Introduction to the Plasma Membrane and Lipids The plasma membrane is made up of phospholipids which form a double layered sheet called the ‘lipid bilayer’ ○ This layer is semi-permeable Within this layer there are specialized proteins that move molecules through the membrane in and out of the cell ○ Hydrophobic molecules and small neutral molecules can freely diffuse Phospholipids Phospholipids are independent entities that do not form polymers, instead they are separate molecules which cluster together Head Group Attaches to the phosphate group and its chemical properties determine where the phospholipid ends up in the cell membrane Phosphate Group The hydrophilic and charged component of the phospholipid Glycerol A 3-carbon chain with three hydroxyl groups The backbone of the phospholipid Fatty Acid Tails Two long hydrocarbon chains which can vary in composition and bond number (this alters rigidity) Six Major Types of Phospholipid Head Groups Polar PI PG CL Charged PS PE PC Other Common Lipids in the Plasma Membrane Cholesterol Major plasma membrane component in animal tissues Has a hydroxyl group on one of its rings which will interact with the surface of the membrane Glycolipids Have a sugar carbohydrate group attached to the lipid, but lack a phosphate group Often involved in cell-to-cell signalling Most common backbones are sphingosine and glycerol Sphingomyelin Resembles a phospholipid but has a sphingosine backbone rather than glycerol Common to the myelin sheaths found wrapping around the axons of nerve cells The Formation of the Plasma Membrane Micelles and Liposomes When surrounded by polar water, phospholipids will form either droplets with fatty acid tails in the centre (micelles) or tight bilayers with a hollow middle (liposomes) Monolayer At the boundary of water and atmosphere, phospholipids will form a single layer with the polar head groups pointed down into the polar water Bilayer Within cells, phospholipids form bilayers with polar head groups pointed out towards the aqueous environment inside and outside of the cell (tails are in the middle) Plasma Membrane Leaflets In cells, the phospholipid bilayers are referred to as ‘leaflets’ ○ The leaflet facing the cytoplasm (interior of the cell) is called the cytosolic face, or the cytosolic leaflet ○ The leaflet facing the exterior of the cell is the exoplasmic face, or the exoplasmic leaflet For organelles with a single layer plasma membrane, the “endoplasmic” face will now be called the luminal face Composition of the Plasma Membrane Leaflets Plasma Membrane The amount of SM, PC, PS, and PI is variable between the exoplasmic and cytosolic leaflet Exoplasmic Leaflet The major components of the endoplasmic leaflet are positively charged phospholipids such as PC and SM This is where glycolipids are located Cytosolic Leaflet Tends to be made up of neutral and negatively charged phospholipids like PS However, PE, which is positive and polar is also a major component Maintaining Lipid Asymmetry Asymmetry is used by the cell to maintain function of the plasma membrane and the organelle membranes within the cell Floppases Selective floppases keep most PC, sphingomyelin, and cholesterol in the exoplasmic leaflet “flOp Out” Flippases Selective flippases keep most PS, PE, and PI in the cytosolic leaflet “flIp In” Scramblases Briefly disrupt membrane asymmetry by randomizing phospholipids Scramble up the asymmetry The Selective Permeability of the Plasma Membrane Can Diffuse Freely Small uncharged and hydrophobic molecules ○ Oxygen, nitric oxide, carbon dioxide However this can only occur if there is a sufficient gradient moving them in this direction ○ Molecules naturally move from areas of high concentration to areas of low concentration ○ In the case of water is is referred to as an osmotic gradient and described how water moves across a membrane to areas of higher solute concentration ○ Water flows through passive transport channels called aquaporins that have a hydrophilic interior and allow water to move through the plasma membrane Cannot Diffuse Freely Hydrophilic compounds and large molecules cannot pass through Tonicity Osmotic pressure is measured in a cell by a property called tonicity ○ This described the relative concentrations of solutes on either side of a cell’s membrane Hypertonicity Occurs when there are greater amounts of solute outside the cell than inside, this causes water to flow out of the cell The cell loses volume Isotonicity Occurs when there are equal amounts of solute inside and outside of the cell This is the ideal state for a cell Hypotonicity Occurs when there are lower amounts of solute outside the cell than inside, this causes water to flow in This causes the cell to swell and can result in cell lysis The Fluid-Mosaic Model States that membranes can be considered a two-dimensional liquid where all lipid and protein molecules can diffuse more or less easily The “mosaic” part is because there are multiple components to membranes, not just the phospholipid bilayer The “fluid” part of the model is because the lipids and other molecules can move freely in the two-dimensional plane of the membrane, and do so rapidly Membrane Constituents Phospholipids, cholesterol, and proteins can join together to form complexes in the membrane Hydrophilic Groups The hydrophilic head groups of phospholipids can interact with hydrophilic portions of membrane proteins A cluster of membrane proteins, phospholipids, and other membrane constituents is called a lipid raft Factors Affecting Membrane Fluidity Temperature As temperature increases, the membrane becomes more flexible Lipid Content Shorter lipid chains move much more compared to longer chains Unsaturated lipids cause more space in the plasma membrane which leads to move movement Cholesterol Content Cholesterol is bulky and rigid, and acts as a spacer in lower concentrations (under 50%) making membrane more fluid Protein Content Proteins tend to be in complexes with each other and other parts of the cell membrane and sometimes form “rafts” Membranes which are protein rich have less movement in the membrane Membrane Proteins Signalling Molecules Include proteins involved in cell communication Integrins Membrane bound proteins that facilitate cell adhesion and cytoskeleton movement Receptors Those located on the surface of cells can facilitate endo- or exocytosis or can be used in cell signalling Channels and Transporers Move material across the membrane Anchors and Junctions Help cells move and attach to other cells and the extracellular matrix Membrane Transport The process by which cargo that cannot diffuse is moved across the membrane Passive Transport This is the movement of molecules across a membrane down the concentration gradient, meaning this process does not require energy Channel Proteins Form pores allowing water and small charged molecules such as ions to pass through the hydrophobic membrane ○ Ex. Aquaporins Carrier Proteins Undergo a conformational change to allow their cargo to pass from one side of the membrane to the other. They can be considered active transporters if this conformational change requires energy Active Transport Requires energy to move molecules across the membrane against their concentration gradient Direct The pump moves molecules across a membrane against a gradient Ex. Sodium / Potassium pump which directly uses ATP Indirect Ex. Sodium / Glucose symporter which uses the sodium to create a glucose gradient Does not directly use ATP, but uses the sodium gradient established by a direct active transporter Symporters Move molecules in the same direction Antiporters Will move one molecule in and the other molecule out Cellular Metabolism Catabolism The breakdown of cellular macromolecules This causes the release of the energy stored within macromolecules so it can be transferred to other macromolecules This energy is ultimately stored as ATP and is the cell’s primary source of energy End produces are ATP, amino acids, and waste products Anabolism The production of cellular macromolecules These reactions consume the ATP produced by catabolism, as small molecules are built into the macromolecules needed by the cell End products are proteins, complex sugars, and fatty acids Adenosine Triphosphate (ATP) ATP is the primary energy source for cellular processes ○ It is composed of an adenine molecule, a ribose sugar, and a chain of three phosphates Lots of energy is stored between the second and third phosphates ○ When the third phosphate is removed, Adenosine Diphosphate (ADP) is formed and energy is released, which can then be used in cellular processes Guanosine Triphosphate (GTP) Identical to ATP, except the adenosine has been replaced with guanosine Provides the energy for forming the peptide bonds in protein translation Other High-Energy Molecules NAD+ Nicotinamide adenine dinucleotide is converted to a high energy form by the addition of a hydrogen ion and two electrons, producing NADH FAD Converted to a high energy by the addition of two H+ ions and two electrons, which produces FADH2 Mitochondrial Structure Cristae The inner membrane appears to be folded in on itself to form structures known as cristae This is where enzymes convert high-energy compounds into ATP Matrix The inside of the mitochondria is called the matrix and this is where macromolecules are converted into small, high energy compounds such as NADH These compounds are then converted into ATP within the cristae Cellular Respiration (ATP Production) Comprises the catabolic reactions and processes that convert organic macromolecules into ATP Removal of High-Energy Electrons High-energy electrons are stripped from these macromolecules and stored in high-energy electron carriers like NAD+ and FADH Creation of a Proton Gradient High-energy electrons are combined with protons and molecular oxygen to create water Energy is stored as a proton gradients across the mitochondrial inner membrane, which is later used to generate ATP Energy Storage Carbohydrates Stored mainly as glycogen in muscles and the liver Muscle glycogen is more readily available for actively working muscle, this is because liver glycogen needs to be broken down into glucose and then secreted into the plasma Fats Stored as triglycerides throughout the body They must be broke down to release free fatty acids that need to be transported into the cells which require energy production Protein Stored mainly as skeletal muscle ○ This is why muscle wasting occurs if the body needs to use proteins as an energy source Sources of Glucose for Cellular Metabolism Monosaccharides Most commonly consumed in the form of glucose Disaccharides Ex. Lactose Polysaccharides Act as energy storage molecules and must be broken down before they can be used to produce cell energy Getting Glucose into Cells Glucose first enters the bloodstream from ingested foods, through the formation of complex molecules from simpler molecules (de novo synthesis) ○ Or it can be obtained from the breakdown of glycogen stores The most common way to get glucose into cells is by glucose transporters (GLUT), found in most mammalian cells The 10 Steps of Glycolysis As catabolic reactions are exothermic, they release heat. Glucose has a lot of energy stored within it, and if all that energy was released at the same time the resulting heat would likely kill the cell Distributing the process over 10 steps allows the energy stored in glucose to be released little by little, allowing it to be transferred to other cells efficiently without losing large amounts of heat In addition, the 10-step process allows other monosaccharides to enter glycolysis and be converted into one of the intermediary products that are inserted into the process at a later stage ○ However, they do not result in the same energy yield as glucose Glycolysis in Three Stages Stage 1 One glucose molecule is converted into two glyceraldehyde 3-phosphate molecules (G3P) using the first five reactions Requires 2 ATP Stage 2 Each G3P enters the sixth and seventh reactions and is converted to a molecule called 3-phosphoglycerate For each G3P, this stage produces 1 ATP and 1 NADH ○ 2 ATP and 2 NADH are produced Currently at this stage, net ATP is zero Stage 3 Starts with 3-phosphoglycerate and utilized 3 reactions to convert it to pyruvate For each 3-phosphoglyceride, this stage produces 1 pyruvate and generates 1 ATP ○ There is a total of 2 pyruvate and 2 ATP produced By the end of this stage in glycolysis, once glucose molecule has been converted to 2 pyruvate and has produced 2 ATP and 2 NADH Glycolysis Summary Glucose + 2 ADP + 2 NAD+ → 2 pyruvate + 2 ATP + 2 NADH The Fates of Pyruvate Pyruvate is not the endpoint of glucose metabolism ○ As all the enzymatic processes of glycolysis are reversible, if pyruvate were to accumulate into a sufficient amount, then it is possible that the forward conversion of glucose to ATP would stop and discontinue ATP production To prevent this from happening, pyruvate if further processed ○ How this occurs is dependent on whether or not molecular oxygen is present Anaerobic Metabolism Occurs without the absence of oxygen In this scenario, pyruvate undergoes fermentation so that the high energy molecule NADH can be oxidized to NAD+, allowing it to be regenerated so glycolysis can continue to make ATP Anaerobic metabolism produced one of two products ○ Lactate (lactic acid) — produced in most animal cells and bacteria ○ Ethanol — produced in plant cells and yeast Aerobic Respiration In the presence of oxygen, far more ATP can be produced than by glycolysis alone Five Stages of aerobic respiration 1. The conversion of pyruvate to acetyl-CoA 2. Krebs cycle 3. The electron transport chain 4. Chemiosmotic gradient 5. Formation of ATP by ATP synthase Converting Pyruvate to Acetyl-CoA At the end of glycolysis, pyruvate is in the cytosol of the cell and must be transported into the mitochondria (the site of ATP production) ○ A carrier brings pyruvate into the matrix Once pyruvate is within the matrix, it has a carboxyl group removed by the pyruvate dehydrogenase complex ○ This complex takes the pyruvate, an NAD+, and a CoA molecule and converts them into acetyl-CoA, NADH, and CO2 ○ Following this, the acetyl-CoA molecule can then enter the krebs cycle The Krebs Cycle The function of the Krebs cycle is to complete the oxidation of carbohydrates, proteins, and fats to produce the substrates for cellular energy production Acetyl-CoA Enters the Krebs cycle by combining with oxaloacetate to form citrate (lots of energy is stored in it) CoA is released and can be recycled to produce more acetyl-CoA Citrate Citrate is broken down by several chemical reactions to remove two of the carbons and create succinate. The removal of carbons transfers energy to two NAD+ to form two NADH and releases the carbons in the form of tow CO2 and one GTP Succinate Succinate is converted back into oxaloacetate by multiple processes These processes release the high-energy molecules in the form of one NADH and one FADH 2 Products of the Krebs Cycle 4 ATP, 10 NADH, and 2 FADH2 The Electron Transport Chain (ETC) The purpose of the ETC is to use the energy of the energy carriers (NADH and FADH2) produced by the Krebs Cycle to pump protons into the intermembrane space NADH enters the ETC first and moves more protons → generates more ATP Complexes of the ETC Complex 1 Uses electrons from NADH to pump protons from the matrix to the intermembrane space Complex 2 Passes electrons from FADH2 to the electron transport chain, releasing protons into the matrix Complex 3 Uses electrons from both NADH and FADH2 to pump protons from the matrix to the intermembrane space Complex 4 Uses electrons from Complex 3 to pump protons from the matrix to the intermembrane space ATP Synthase The ETC concentrates protons within the intermembrane space, which leads to the generation of ATP in a chemiosmotic reaction ATP synthase is an enzyme located within the inner membrane and uses the established proton gradient to phosphorylate ADP into ATP, creating the useful energy for the cell One glucose molecule that undergoes glycolysis and aerobic respiration can produce 36 ATP (this is the theoretical maximum) ○ 30 ATP are realistically produced per glucose molecule Aerobic Respiration Summary Stage 1 Pyruvate Oxidation ○ Takes place in the mitochondrial matrix and converts pyruvate into acetyl-CoA ○ This process produces one NADH molecule and one CO2 molecule per pyruvate molecule Stage 2 Krebs Cycle ○ Also occurs within the mitochondrial matrix ○ Produces CO2, ATP, NADH, and FADH2 Stage 3 Electron Transport and Proton Pumping ○ The ETC happens across the inner mitochondrial matrix and creates a chemiosmotic gradient of protons with a high concentration in the intermembrane space ○ This process also combines oxygen and protons to make water Stage 4 Chemiosmotic Gradient ○ Protons are in high concentration in the intermembrane space of the mitochondrial membrane and move across this membrane into the matrix where they generate ATP to power ATP synthase Stage 5 ATP Synthesis ○ This process also happens in the inner mitochondrial membrane, and uses the high concentration of protons in the intermembrane space to support the process of phosphorylating ATP Excess ATP The enzyme class creatine kinases convert creatine and ATP into creatine phosphate and ADP Deficiency in ATP The phosphate from creatine creatine phosphate is transferred to ADP to form ATP This pathway for ATP generation does not require molecular oxygen so it can occur during anaerobic conditions Other Sources of Cellular Energy Fat Metabolism Free fatty acids (fatty acids circulating in the bloodstream) are taken up into cells by a family of fatty acid transport proteins Once inside the cytosol of a cell, the fatty acid must be “activated” before it can be transported into the mitochondria. This process of “activation” requires 2 ATP per each FFA Inside the mitochondria, fatty acyl-CoAs are metabolized by beta-oxidation to produce high-energy molecules Transport of Fatty Acids If the fatty acid chain is short enough, it can simply diffuse into the mitochondrial matrix However, long chains must be transported into the mitochondria by a process called the carnitine shuttle Beta Oxidation This is the process by which fatty acids are metabolized in order to release acetyl-CoA ○ A 10 carbon fatty acid would produce 5 acetyl-CoA molecules and would produce 64 ATP total Destination of Protein Metabolites Depending on the original structure of the amino acid, there are different possibilities once the nitrogen has been removed to enter the cell’s metabolism and generate ATP Some result in three-carbon molecules that can be used to synthesize glucose or enter glycolysis Some result in the formation of acetyl-CoA that can directly enter the Krebs Cycle Some result in products that can enter as intermediaries of the Krebs Cycle Energy Preferences The Brain The primary energy source for neurons and the brain is glucose, however it can also derive some of its energy needs from ketones produced from the metabolism of fats in the liver The Heart Primary fuel choice is fatty acids, though some ketones can be used It has the highest mitochondrial density of all cells and it operates exclusively on aerobic respiration ○ This is why that anything which dramatically decreases blood supply to the heart (and therefore oxygen supply) can cause rapid, serious, and irreversible damage Skeletal Muscle The major sources for ATP production are glucose, fatty acids, and ketones Has very large stores of glycogen that can be rapidly broken down to produce ATP Under heavy exercise, oxygen levels may drop such that pyruvate will undergo anaerobic metabolism to produce lactate In resting muscle, the major source for ATP production is fatty acids Energy Demands (Skeletal Muscle and Exercise) Low Intensity Stored fats become a primary source of energy as long as there is sufficient oxygen Medium Intensity There is a shift to using stored carbohydrates, or glycogen, which is converted to glucose Depending on oxygen levels, this newly converted glucose either undergoes aerobic or anaerobic respiration High Intensity Muscle cells are mainly depended on muscle glycogen but this is usually accompanied by a lack of oxygen and therefore anaerobic respiration with lactate accumulation Creatine phosphate is also used as ATP is depleted Generally proteins are not used for energy production unless all other options have been exhausted