IMMS Cell Ultrastructure Revision Guide PDF
Document Details
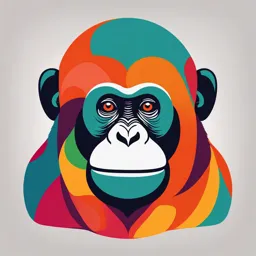
Uploaded by EliteEnlightenment3617
The University of Sheffield
Tags
Summary
This document provides a revision guide on cell ultrastructure, covering various cell organelles and their functions. Key topics include the nucleus, mitochondria, plasma membrane, microtubules, and microfilaments.
Full Transcript
IMMS 1.1 Cell Ultrastructure 1 1.2 Cell Ultrastructure 2 Nucleus Mitochondria Plasma Membra...
IMMS 1.1 Cell Ultrastructure 1 1.2 Cell Ultrastructure 2 Nucleus Mitochondria Plasma Membrane Microtubules Largest membrane bound organelle Site of oxidative phosphorylation Double layer of lipids called 25nm Storage and transmission of genetic Outer membrane – lipid synthesis and fatty acid metabolism phospholipids E.g. tubulin (alpha and beta which information Inner membrane – respiratory chain (electron transport) Hydrophobic head, hydrophilic tail arrange into groups of 13 to form hallow Information (coded in the DNA) Matrix – Krebs Cycle Contain proteins (receptors/channels) tubes) synthesises the protein determining the Intramembranous space – nucleotide synthesis (ADP – ATP) and lipids (cholesterol) Arise from centromere structure and function of the cell. Found in all cells, except RBC’s, so they Double membrane (nuclear envelope) Functions have no nuclei, no cell division, thus with gaps called nuclear pores Controls passage of various molecules structure isn’t required RNA moves out via pores Vesicles Physical barrier DNA and proteins form chromatin – a Very small spherical membrane Nucleolus Selective permeability Intermediate filaments mass of genetic material bound organelles which transport In the nucleus Endo-/exocytosis 10nm At cell division, chromatin becomes and store material and exchange No membrane Cell signaling 6 protein types chromosomes, condenses. cell membrane between Site of DNA transcription Anchored transmembrane proteins which compartments, Forms ribosomal RNA Components can spread through tissues Golgi Apparatus Many types: Cell surface derived, Phospholipids – lipid made of glycerol, 2 Parallel stacks of membrane golgi-derived, ER-derived, fatty acid tails and a phosphate linked head group Microfilaments Processes and modifies macromolecules lysosomes and peroxisomes 5nm synthesised in the ER Cholesterol Membrane proteins Actin forms a something mesh (cell Located close to the nucleus cortex) on the inner surface of the cell In most cells it cant be seen – seen clearly in Vacuole Carbohydrate groups Functions to hold various solutions or These then attach to proteins to form membrane plasma cells. Cis-Golgi material. glycoproteins or glycolipids Nuclear facing – receives from rough ER These can have been created, stored Sphingolipids? Protein phosphorylation occurs here. or excreted and that have been phagocytosed or engulfed. Centrosome Medial Made from 2 centrioles, which are Modifies producers by adding sugars Chamber surrounded by a membrane Cell Junctions microtubule rings Forms complex oligosaccharides by adding – this is semi permeable and only lets Tight junctions – seals neighbouring cells together in an certain molecules through They organize microtubules and provide sugars to lipids and peptides. epithelial sheet to prevent leakage structure for the cell Trans-Golgi Adherens – joins an actin bundle in one cell to a similar Pull chromatids apart during cell division Proteolysis of peptides into active forms and bundle in another cell sorting of molecules into vesicles which bud Desmosomes – joins the intermediate filaments in one cell from the surface to a neighbour Gap junctions – allows passage of small water soluble ions Lysosomes Smooth ER and molecules Contain digestive enzymes Rough ER Site of lipid synthesis Hemi-desmosomes – anchor intermediate filaments in a cell Waste disposal system, and Rough due to the ribosomes on the Proteases and store synthesized to the basal lamina the site of breakdown for surface most molecules Highly folded membrane sheets proteins High folded, flattened membrane Derived from Golgi. H+ which are flat Endosomes ATPase on membrane Site of protein synthesis sheets,. Membrane bound vesicular and creates low pH (pH5) to tubular structures that live enable acid hydrolysis to Ribosomes Cytoplasm between golgi and membrane. function 2 subunits attached to the rough ER Site of glycolysis Acts as a large catalyst The fluid that fills the cell, includes the cytosol and filaments, proteins, ions and macromolecular structures as well as Peroxisomes Translates genetic code into chains of amino acids – organelles. NA the nucleus. Small membrane bound organelles containing enzymes which oxidase these then fold 3 components: long chain fatty acids. Deposits protein into the ER to undergo further Cytoskeleton with associated motor proteins Involved in the process by which fatty acids are broken down to modification Organelles and other multi proteins complexes. generate ATP 3 – 5 amino acids per second in protein production Cytoplasmic inclusions and dissolved solute Hydrogen peroxide is toxic to cells but is destroyed by peroxisomes. 1.3 Molecular Building Blocks Basic Building Blocks Lipids Amino Acids Atoms Triglyceride = 3 fatty acids bound to glycerol Building blocks of proteins - 20 in total Carbon, hydrogen, oxygen, nitrogen, sulphur, phosphate Straight carbon chains with a methyl group and a carboxyl group Carbon with amino group, carboxyl group and a specific side at ends chain Macromolecules Tend to be hydrophobic and contain no oxygen in main chain Most natural amino acids are in the L form, in contrast to that of Simple molecules (sugars, lipids, amino acids) form larger more In unsaturated fatty acids - double bonds are commonly cis, sugar whose natural form is D. complex ones spaced at 3C intervals Can have osmotic, structural, optical, enzymatic, and other Charge functions Charge is determined by all three components and on the pH of E.g. haemoglobin, DNA, glycogen, rhodopsin and collagen Proteins the environment Side chain often determines polarity (and thus solubility and Amino acids linked together by peptide bonds permeability) Protein is a large polypeptide –from 10s to 1000s amino acids Carboxyl groups = negative Carbohydrates Function is totally dependent on structure Amino groups = positive Monosaccharides Protein = if it is functional and synthesized by a cell Peptide bond One hydroxyl group Peptide = bit of protein broken off Formed by condensation An aldose has an aldehyde An ketose has a ketone reaction (water released) Folding Generally exist as ring structures (cyclized) Linear chains fold in different shapes to form 3D structures – Properties Determined by charged interactions, flexibility, amino acid Very stable Glycosidic bond sequence, and physical dimensions Cleaved by proteolytic enzymes – proteases or peptidases The hydroxyl group of a monosaccharide can react with an OH or an NH group to form a glycosidic bond. Can have partial double bonds Structure Flexibility around C atoms not involved in bond thus allows O-glycosidic bonds form disaccharides, oligosaccharides and Primary: linear sequence of amino acids, multiple conformations polysaccharides. Secondary: alpha helix or beta pleated sheets formation due to Usually one preferred conformation, determined mainly by types N-glycosidic bonds are found in nucleotides in DNA H+ bonds between amino acids – determined by local of side chains and amino acid sequence interactions between side chains and sequence of amino acids. Disaccharide Tertiary: Overall 3D conformation of a protein. Confirmation can Contain two monosaccharides joined by an o-glycosidc bond. change with temperature or pH. Quaternary: 3D structure of protein with multiple subunits. Enzymes Oligosaccharides Contain 3 – 12 monosaccharides. Catalysts = Provide an alternative reaction pathway with a lower Forces activation energy Product of digestion of polysaccharides, or part of a complex Van der Waals forces: Weak attractive/repulsive force between Enable reactions to occur that otherwise would not be able to protein or lipid. all atoms due to fluctuating electrical charge. occur at physiological (body) temperatures and conditions. Hydrogen bonds: Interaction between polar groups. Bind to the reactants and convert them to products – then Polysaccharides Hydrophobic forces: as uncharged and non-polar side chain are release the products and return to their original form Formed by thousands of MS joined by glycosidic bond. Glycogen – branched polysaccharide formed of glucose residues. repelled by water, hydrophobic side chains form tightly packed Speed up reactions and provide a way to regulate the rate of cores in the interior of proteins, excluding water molecules. reactions Ionic bonds: between fully/partially charged groups. Disulphide bonds: very strong covalent bonds between sulphur Regulation atoms. Altering concentration of substrates, products, inhibitors or Nucleotides activators, can also be regulated by modifying the enzyme itself by phosphorylation Building blocks of DNA. Made from nitrogenous base + sugar + phosphate. Isoenzyme: enzymes that have a different structure and Bonds between bases are hydrogen bonds sequence but catalyze the same reaction Bonds between phosphate and sugar are phosphodiester Coenzyme: cannot in themselves catalyze a reaction but can help Phosphate bonds in nucleotides are a source of energy enzymes to do so. Bind with the enzyme protein molecules to form active enzymes. 1.4 Communication and Homeostasis Types of Communication Body Fluid Compartments – 70kg Man Water Balance Autocrine – messenger molecules bind with receptors in the cell Total volume Water intake: drink, diet and IV fluid. where they are produced 60% of body weight Water loss: kidneys E.g. chemical/secondary messengers 42L insensible losses: sweat, breath, vomiting and faeces Paracrine – messengers in ECF E.g. clotting factors, prostaglandins in childbirth, Regulating hormones: ADH, Aldosterone and atrial natriuretic inflammatory mediators Extracellular fluid (ECF) Intracellular fluid (ICF) peptide (ANP) Endocrine – secretions into blood 14L 28L E.g. insulin Predominant electrolytes = Predominant electrolyte = K+ Dehydration Exocrine – Na+/Cl-/HCO3-/Ca2+/urea/glucose Causes: water deprivation, vomiting, diarrhoea, burns, heavy E.g. sweating, diabetes insipidus, diabetes mellitus and drugs Consequences: thirst, inelastic skin, sunken eyes, raised Intravascular fluid Extravascular fluid haematocrit (viscosity of blood), weight loss, and hypotension. Feedback (plasma) 11L Water Excess 3L Positive Feedback – amplification of signal Circulating extracellular Causes: high intake/ decreased loss of water, excess ADH E.g. clotting cascade, oxytocin, and labour component of blood Consequences: Hyponatremia (low sodium levels), cerebral over Negative Feedback – the basis for homeostasis perfusion (due to high blood volume and thus pressure) – causes E.g. the majority of endocrine hormones headaches, confusion and convulsions. Dysfunction leads to disease Interstitial fluid Transcellular Dysfunction 10.5L 0.5L Serous effusion: excess water in body cavity Surrounds cells but Makes up CSF, digestive Oedema: excess water in the intercellular tissue space does not circulate juices and mucus Inflammatory (leakage) - proteins leak out due to increased vascular permeability they bring in water, thereby diluting the Hormones Fluid compartments in the body are in osmotic equilibrium. toxins, fibrinogen polymerises to form a fibrin mesh and Molecules that act as chemical messengers Osmotically active substances (solutes) in the ICF (potassium) immunoglobulins collect. 3 types and ECF (sodium, chloride, glucose and urea) create an osmotic Venous (increased end pressure) – due to increased venous gradient across cell membranes. pressure or venous obstruction from a thrombus. 1. Peptide Lymphatic (blocked) – obstructions from a tumour/parasite Made from short chain amino acids. Hypoalbuminemia – lower oncotic pressure Stored in cell and released when needed/signalled Solution/Water Definitions Binds to receptor on membrane ECF osmolality change Produces a quick response via a secondary messenger Osmosis: net movement of solvent molecules through a Leads to rapid response, very tightly regulated cascade semipermeable membrane to higher solute concentration (i.e ECF volume change Examples: Insulin, growth hormone, TSH and ADH. lower water conc.) Slower response 2. Steroid Osmolarity: concentration of solutes in plasma per kg of solution Synthesised from cholesterol, water insoluble and lipid Osmolality: concentration of solutes in plasma per kg of solvent Electrolyte Balance soluble – can cross membranes but requires transport Osmotic pressure: pressure applied to a solution, by a pure Requires transport proteins in blood solvent, required to prevent inward osmosis, through a Sodium Intracellular receptor target semipermeable membrane. Hypernatremia: High sodium. Steroid hormone is made by the cell and diffuses out Oncotic pressure: form of osmotic pressure exerted by protein Hyponatremia: Low sodium once made (not stored that tends to pull fluid into its solution – water moves from ISF Directly affects DNA and alters transcription/translation into plasma. Potassium – this is a slow response as proteins have to be made Hydrostatic pressure: pressure difference between capillary Hyperkalaemia: high potassium Examples: testosterones, oestrogen and cortisol blood (plasma) and interstitial fluid – water and solutes move Hypokalaemia: low potassium 3. Amino-acid derivative from plasma into ISF Synthesised from tyrosine, acts in same way to peptide. Calcium Examples: adrenaline, thyroid hormones (T4 and T3) Hypercalcemia: high calcium. Hypocalcaemia: low calcium 1.5 DNA Structure and Location Functions Mutations Found in nucleus and mitochondria Storing and transferring genetic information Duplications - sections repeating, incorrect protein generated Arranged in a double helix with complimentary base pairing Template and regulator for transcription and protein synthesis. Deletions DNA coils around proteins (histones) and form nucleosomes > DNA is the genetic material thus the structural basic of hereditary Out of frame – sequence shifts meaning the reading supercoils > chromosomes and genetic diseases. frame of the gene is changed. In frame – whereby one codon is removed thus only one 46 Chromosomes, 22 Pairs (autosomes – anything not amino acid is lost. Reading frame is not changed. sex-determined) and a pair of sex chromosomes (XY- Male, XX – Mutations of regulatory sequence – coding sequence still intact, Female) Structure of Nucleic Acids but gene itself is switched on or off etc. Karyotype: number and appearance of chromosomes in a cell. DNA damage – from chemicals, UV and radiation Nucleotides are the building blocks of new DNA DNA repair issues - base or nucleotide excision, mismatch repair Spreads are arranged in size order Free phosphate groups provide energy for the reaction to go or transcription-couples repair through Prokaryotes (less compartmentalization) – no nuclear Non – sense - Point mutation that produces a stop codon - membrane, DNA arranged in single chromosomes. results in an incomplete, usually non-functional protein. E.g. Eukaryotes (more compartmentalization) – DNA is in a nucleus, Duchenne’s muscular dystrophy bound to proteins (chromatin complex). Mis-sense mutation - A point mutation in which a single Each chromosome is made of 2 identical strands of chromatids nucleotide change results in a codon that codes for a different joined in the centre (centromere). amino acid (substitution). This can have a varied affect and can result in a silent mutation and a non functional protein E.g Sickle Some DNA is found in mitochondria (purely maternal DNA.) cell disease where CAG was replaced with CTG. Spice site mutation – affects the accurate removal of an intron Expansion of tri-nucleotide repeat - Huntington's disease : CAG. Folding Triple repeat is repeated several times in the first part of the coding sequence If > 36, the patient will develop Huntington’s- Anticipation: in diseases such as Huntington’s, repeats get bigger when they are transmitted to the next generation resulting in earlier symptoms of greater severity t his process is called anticipation. 1.6 Replication, Transcription and Translation Replication Transcription 1. Prior to cell division, topoisomerase unwinds DNA and DNA Synthesis of mRNA from DNA helicases separates DNA apart to expose two single DNA strands 4 Stages: and create two replication forks. DNA replication then takes 1. Preparation place simultaneously at each form. Topoisomerase unwinds the double helix by relieving the supercoils. 2. Single stranded binding protein (SSB’s) coat the single DNA DNA helicase then separates the DNA apart exposing the strands to prevent reannealing or snapping back together nucleotides. Single stranded binding proteins (SSBP’s) coat the single DNA 3. The primate enzyme then uses the original DNA sequence on strands to prevent DNA re-annealing. the parent strand to synthesise a short RNA primer. Primers are necessary since DNA polymerase can only extend a nucleotide 2. Production chain, not start one. TATA sequence is the recognition signal for starting AUG is the start codon – codes for methionine 4. DNA polymerase begins to synthesise a new DNA (via Free mRNA nucleotides line up next to their complementary complementary base pairing using free floating nucleotides) bases on the template strand/ antisense strand of DNA strand by extending an RNA primer in 5’ and 3’ direction. Each U-T & C-G parental strand is copied by one DNA polymerase (lead strand Coding strand runs 5’ to 3’ and lagging strand). Template strand runs 3’ to 5’ 5. As replication proceeds RNAse H recognizes RNA primers bound 3. Termination to the DNA template and removes the primers by hydrolyzing RNA polymerase (specifically RNA polymerase 2) joins the mRNA the RNA nucleotides (catalysing phosphodiester bonds between them) Forms antiparallel mRNA strand (with a 5’CAP head and a 3’Poly 6. DNA polymerase can then fill the gap left by RNAse H A tail) - starting at a promoter (specific sequence that RNA polymerase binds to - initiation of transcription. 7. DNA replication process completed when the ligase enzyme Transcription is stopped at the stop codon – UAA/UAG/UGA joins the short DNA pieces (Okazaki fragments) together into one continuous strand. 4. Modification (splicing) The removal of introns Leaves exons – the coding part Template mRNA leaves the nucleus through nuclear pores 1. mRNA attaches to an 80s ribosome with large and small subunits 2. APE section in large subunit – A at the 5’ end of mRNA strand 3. Strand read 5’ to 3’ 4. At ribosome the mRNA (bases on mRNA are read in 3’s - codons) sequence is used as a template to bind to complementary tRNA molecules at their anticodon (3 bases complementary to codon on mRNA). 5. tRNA molecules are attached to specific amino acids 6. Enzymes remove amino acid from tRNA and amino acids are linked together by a peptide bond (created by a condensation reaction), creating a polypeptide chain 1.7 Meiosis and Mitosis Structure and Location Meiosis Mitosis Mitosis Found in nucleus and mitochondria Genetically Genetically Prophase different gametes identical to parent Arranged in a double helix with complimentary base pairing cell Nuclear membrane disintegrates DNA coils around proteins (histones) and form nucleosomes > Chromatin condenses into chromosomes Chromosome Chromosome supercoils > chromosomes number halved number retained Centrosomes nucleate microtubules and move to opposite poles and nucleus Prokaryotes (less compartmentalization) – no nuclear Haploid gametes Diploid daughter Prometaphase cells membrane, DNA arranged in single chromosomes. Nuclear membrane breaks down Eukaryotes (more compartmentalization) – DNA is in a nucleus, Final division in Somatic cell Microtubules invade nuclear space gamete division, gamete bound to proteins (chromatin complex). maturation formation Chromatids attach to microtubules Each chromosome is made of 2 identical strands of chromatids Cell no longer had a nucleus Two divisions One Division joined in the centre (centromere). Chromosomes attach to spindle via centromere Metaphase Some DNA is found in mitochondria (purely maternal DNA.) Chromosomes line up along metaphase plate Anaphase Sister Chromatids separate and are pushed to opposite poles of the cell, centromere first, as spindle fibers contract Telophase Nuclear membrane reforms Mitosis Chromosomes unfold into chromatin Cytokinesis begins Cytokinesis Cell organelles become evenly distributed around each nucleus Cell divides into two daughter cells with a nucleus in each. Meiosis G2 Checkpoint G2 Checks to see if everything is alright Chromosomes condense – coil up and become visible Energy stores accumulate Mitochondria and centrioles double Mitosis See above Cell Division 2 diploid cells produced S (synthesis) Meiosis DNA doubles through DNA replication G1 Histone proteins double through protein Preparation of replication Prophase I – crossing occurs between non-sister chromatids. synthesis Results in genetic diversity Twice as much DNA at the end of Metaphase I – random assortment occurs on the metaphase synthesis G0 plate. This also produces genetic diversity Normal function/repair Meiosis II – sister chromatids separate. Haploid cells are Some cells never leave this state e.g. produced neurons G1 Checkpoint Checks if everything is alright 1.8 Inheritance and Disease Non-Traditional Homozygous vs. Heterozygous Mitochondrial All mitochondria is inherited from the mother Imprinting One allele active the other is inactive Mosaicism Error in cell division, the same cells have a different genetic makeup Mendelian Autosomal Recessive Disease manifests in homozygous state Requires 2 defected genes Chance having disease = 25% Chance of being a carrier = 50% Chance of affected child’s sibling being a carrier = 66.6% Males/females are equally affected Affected individuals are in single generation E.g. Cystic Fibrosis Autosomal dominant Disease manifests in heterozygous state Males/females affected equally Affects multiple generations Transmission from both sexes to both sexes Both parents can sometimes be unaffected E.g. Mitochondrial Gonadal mosaicism (don’t have genes for it) Mother has reduced penetrance Mother has variable expression Only one defected gene needed = 50% chance offspring affected E.g. Huntington’s Disease X-linked Causes of Disease Caused by mutation on the X chromosome Genetic Never male to male – sons always get X chromosome from Individually rare, but cumulatively enough to have regional mother genetic services All daughters from affected males are carriers Eg: Down syndrome, Cystic Fibrosis, Huntington's, Haemophilia Transmitted usually through unaffected female Multifactorial Can be recessive ( Duchenne’s muscular dystrophy) or dominant Combination of genetic and environmental. Main cause of (Alport’s Syndrome) disease in developed countries Eg: Spina bifida, Cleft palate, Diabetes, Schizophrenia Environmental Chromosomal Main causes of disease in third world and A & E (genetics play Can be numerical or structural small role) Location determines behaviour during meiosis Eg: Poor diet, Infection, Drugs, Accident 1.9 Energy Production Glycolysis Krebs Cycle Takes place in the cytosol of the cell Takes place in the matrix of Rate limiting step controlled by phosphofructose kinase mitochondria This is where insulin and glucagon have their effect and control Amount of ATP produced varies the rate of energy production depending on where NADH and Also regulated by levels of ATP and AMP FADH2 ends up Can take place aerobically or anaerobically A further 12 ATP can be produced 4 ATP produced, 2 NADH enters electron transport chain and can in the electron transport chain by produce a further 6 ATP oxidative phosphorylation The rate limiting step is controlled by isocitrate Energy dependant dehydrogenase The rate can also be determined by the levels of ATP, NADH, and FADH2 High levels inhibit the Krebs Cycle It is activated by high ADP If the cycle is inhibited there is a build up of acetyl coA and this undergoes fatty acid synthesis Energy production Electron Transport Chain Oxidative phosphorylation Takes place on the inner membrane of the mitochondria Made up of multiple complexes Complex I – removes electron from NADH Complex II – removes electrons from FADH2 in the presence of co enzyme Q (ubiquinone) Complexes III, IV and cytochrome C donate these electrons to cytochromes containing iron Oxygen is released as a result and converted into H2O 1.10 Fatty Acids Synthesis Metabolism Occurs when there is an abundance of energy – high ATP levels Method of breaking down fatty acids in order to produce energy inhibits the Krebs Cycle and leads to a build up of acetyl coA Occurs in response to decreased blood glucose and high glucagon Synthesis of fatty acids occurs in the cytosol of the cell not in the – mobilises fatty acids to target tissues mitochondria Fatty acids are long acyl coA chains However acetyl coA cannot cross the mitochondrial membranes Dietary fatty acids tend to have more than 14 carbons Fatty acids longer than 12 carbons in length cannot diffuse Citrate Shuttle through the mitochondrial membrane and must be transported Oxaloacetate binds with acetyl coA to produce citrate This can cross the mitochondrial membrane into the cytosol Carnitine Shuttle Citrate ligase converts citrate back to oxaloacetate The acyl coA chains are converted and the reformed in order to This is then broken down into pyruvate and acetyl coA cross the membrane Pyruvate is recycled back into the mitochondria and then Acyl coA to acyl carnitine (by carnitine acyltransferase 1 –CAT1) converted into oxaloacetate and re-enters the Krebs Cycle Located on the outer mitochondrial membrane The acetyl coA is the converted into fatty acids Co A is recycled Acyl carnitine is reformed to acyl coA (by carnitine acyltransferase 2 – CAT2) On the interior side of the membrane Carnitine this recycled through the outer membrane Beta Oxidation Once acyl coA has crossed the membrane it can now be oxidised This involves the sequential removal of 2 carbon units by oxidation – the second (hence beta) carbon is cleaved Each round of beta oxidation produces 1NADH, 1FADH2 and 1 acetyl coA Examples of Fatty Acids: FADH2 and NAD undergo oxidative phosphorylation Linoleic Acid (18 carbons) Acetyl coA re-enters the Krebs Cycle Oleic Acid (18 carbons) ATP is produced Palmitic Acid (16 carbons) Arachidonic Acid (20 carbons) Ketones The production of ketone usually occurs slowly during normal Results in diabetic ketoacidosis feeding and normal physiological status Impairs O2 binding to haemoglobin so present with In response to carbohydrate shortages there is an increased hyperventilation production of ketone bodies Low pH, PCO2 and HCO3-. High PO2 This is due to increased beta oxidation and the production of acetyl coA which exceeds the limit of the Krebs Cycle Ketone Synthesis Ketone bodies are used in preference to carbohydrates to help Two Acetyl-CoA’s are converted by thiolase back to Acetoacetyl CoA preserve glucose Two further enzymes convert it to Acetoacetate Can only be used by cardiac and skeletal muscle This can then enter the blood or can be converted to Ketone bodies – acetone, acetoacetate etc. β-hydroxybutyrate, which can also enter blood. Most significant disruption of ketone bodies comes from When the level of glycogen in the liver is high, the production of untreated insulin-dependent diabetes mellitus β-hydroxybutyrate increases. Leads to hyperglycaemia and increased ketone body levels Acetoacetate and β-hydroxybutyrate can both be oxidised as fuels Ketones are very strong acids in most tissues, including skeletal muscle 1.11 Oxygen Toxicity Oxygen and Reactive Oxygen Species Haber-Weiss Cycle Respiratory Burst Oxygen Four reactions which combine to produce OH and consume H2O2 Immune system defence against bacteria Favours reduction in single electron steps, two unpaired Sudden release of ROS by immune cells (neutrophils, electrons, parallel spin, permits combustion, cellular macrophages & monocytes) during phagocytosis respiration (electron transport chain), formation of free radicals & is highly reactive Immune cells utilise NADPH oxidase to reduce oxygen to superoxide. Reactive Oxygen Species (ROS) Superoxide is released Highly reactive oxygen containing compounds that are free Then further reduced to hydrogen peroxide radicals (have a single unpaired electron in their orbital) or This can be reduced to hydroxyl radical etc. which then generates compounds that are readily converted to oxygen free radicals other reactive oxygen species in the cell – these compounds contribute to ageing, homeostasis and some cancers. Hydroxyl Radical OH Neutrophils & monocytes use myeloperoxidase to further Reactive free radical extract electrons (usually as hydrogen combine H2O2 with Cl- to produce hypochlorite (ClO-) [H2O2 + Most potent of ROS atoms) from other compounds to complete their own orbitals, Cl- > H2O + Cl-] which plays a role in destroying bacteria by Lipid soluble (thus can cause damage away from site of formation thereby initiating free radical chain reactions. damaging bacterial cell membranes. as well as damaging lipid bilayers) The hydroxyl radical is probably the most potent of the ROS Absence or impairment of function of NADPH oxidase prevents Initiator of chain reactions that form lipid peroxides & organic Exogenous sources: UV radiation, tobacco and drugs the formation of ROS and causes chronic granulomatous disease Radicals. Endogenous sources: NADPH & the electron transport chain (X-linked) (build up of pathogens in phagocytes since they can engulf but NOT kill them - leads to severe skin infections with bacteria or fungi) Formation Protection against oxygen toxicity: 1. Oxygen is reduced by a single electron to form superoxide Antioxidant enzymes: 2. Superoxide is further reduced to form hydrogen peroxide Superoxide dismutase - converts superoxide to 3. This is reduced to produce a hydroxyl free radical hydrogen peroxide (non toxic unless converted to 4. Further reduced to produce water another ROS) & oxygen Catalase - catalyses conversion of hydrogen peroxide to water & oxygen and protects white blood cells against own respiratory burst. Glutathione Peroxidase - catalyses the reduction of hydrogen peroxide to water and a disulphide (GSSG) Cellular Damage Antioxidant vitamins: Vitamin E - found in liver, free Hydrogen peroxide – not a radical but is a ROS since it is readily radical scavenger, protects against lipid peroxidation and converted to hydroxyl radical (OH-). Also lipid soluble so can Free radicals damage; proteins, lipids, carbohydrates & nucleic terminates free radical propagation in membranes, cause damage away from site of formation. Transition metals acids Vitamin C - e.g ascorbic acid, reacts with superoxide & such as Fe2+ or Cu2+, catalyse the formation of the hydroxyl They damage membranes of; cells, nucleus, mitochondria & hydroxyl radical and regenerates reduced vitamin E radical from hydrogen peroxide in the non-enzymatic endoplasmic reticulum. This cell membrane damage results in Cellular compartmentalisation - respiratory burst taking Fenton reaction (the iron dependent generation of a hydroxyl the increased permeability of the membrane resulting in an place in phagosomes so harmful chemicals don't get out radical from hydrogen peroxide) by donating a single electron. influx of calcium, water & sodium and damage healthy tissue Membrane damage leads to increased permeability which changes the electrical gradients and thus the functioning of the cell The hydroxyl radical can also be formed by the Haber-Weiss DNA can also be damaged by the hydroxyl radical - this results in reaction involving superoxide (O2 –) strand breaks & base alterations – MUTATIONS Diseases associated with free radical damage; Emphysema, Parkinson’s, Acute renal failure & Diabetes 1.12 Acid-Base 1 Revisited in Kidney and Respiratory Definitions Production of H+ Ions Body Buffer Systems Acid: Proton/H+ donor: HA (acid) H+ + A- (conjugate base) Generated by the process of metabolism Bicarbonate: Base: Proton/H+ acceptor: B (base) + H+ BH+ (conjugate acid) Small amounts are from the oxidation of amino acids and the Most important buffer system in the whole body. anaerobic metabolism of glucose to lactic and pyretic acid Bicarbonate is not an efficient buffer Bronstead-Lawry Theory Far more hydrogen ions and thus acid are produced as a result of Its efficiency is improved because CO2 is removed at the lungs Protons can’t exist alone in a solution due to their high charge carbon dioxide (CO2) release from oxidative (aerobic) and bicarbonate is regenerated by the kidneys. density so they bind to a water molecule metabolism. Although CO2 doesn’t contain hydrogen ions it H2O + H+ H3O+ rapidly reacts with water to form carbonic acid (H2CO3), which Proteins: Acidity is the concentration of H+ ions in solution usually described further dissociates into hydrogen and HCO3- Notably albumin as the inverse log Contain weak acidic and basic groups within their structure. Water can dissociate H2O OH- + H+ Thus plasma and other proteins for important buffering systems. In pure water [H+] is 10^-7 or pH7 Intracellular proteins limit pH changes within cells, whilst the protein matrix of bone can buffer large amounts of H+ ion in Strong acid: A compound that ionizes completely in solution to form Reaction can be sped up by carbonic anhydrase. patients with chronic acidosis. hydrogen ions and a base Carbonic acid and bicarbonate form the most important Weak acids and bases: compounds that are only partially ionized in buffering system in the body. Hemoglobin: solution. H2CO3 H+ + HCO3- The resulting anion Its not only important in the carriage of oxygen to the tissues but (bicarbonate) is referred to as the conjugate base of carbonic acid also in transport of CO2 and in buffering hydrogen ions. Haemoglobin binds both CO2 and H+ and is thus a powerful Base excess: the quantity of acid required to return plasma to pH to buffer. normal Henderson-Hasselbach Equation Deoxygenated haemoglobin has the strongest affinity for both Standard base excess: the quantity if acid required to return General form; CO2 and H+; thus its buffering effect is strongest in the tissues. extracellular fluid to normal pH = pK + log10 ([Base]/[Acid]) Little CO2 is produced in red cells thus CO2 produced by tissues For carbonic acid/bicarbonate system; passes easily into the cell down a concentration gradient. Buffers: All are weak acids or bases with the conjugate base or acid pH = 6.1 + log10 (25mM / (0.03 x 40 mmHg)) CO2 then either combines directly reversibly with terminal amine respectively. Solution which resists charges in pH when small 0.03 is a proportionally constant for CO2 which converts the groups on the haemoglobin molecule to form quantities of strong acids or base are added. partial pressure in mmHg into a concentration in solution which carbominohaemoglobin. in turn equates into a H2CO3 concentration pH: -log10[H+]; the inverse logarithm of the concentration of H+ In lungs – CO2 is released and passes down concentration ions gradient in to the alveoli – buffering of CO2. Normal pH range: 7.35 – 7.45 In tissues – dissolved CO2 passes into RBCs where it combines with water to for carbonic acid. Catalysed by by carbonic Acidosis: blood is more acidic than normal anhydrase. Carbonic acid then dissociates into bicarbonate and Alkalosis: blood is more alkaline than normal H+ ions. The H+ ions bind to reduced haemoglobin to form HHb. Acidaemia: low blood pH HCO3- pass back into plasma in exchange for Cl- Alkalaemia: high blood pH Anion Gap Different in serum concentration of cations (positibe) and anions (negative) e.g Cl-, HCO3-, Na+, K+ = ([Na+] + [K+]) – ([Cl-] - [HCO3-]) Not all ions are included e.g. K+, PO4 -, SO4 Normal value is between 3-11mEq/mol Wide anion gap could indicate; lactic acidosis, ketoacidosis, ingestion of acid, renal failure Narrow anion gap could indicate; GI HCO3– loss, renal tubular acidosis 1.13 Acid-Base 2 Revisited in Kidney and Respiratory The Davenport Diagram Metabolic Alkalosis A graphical interpretation of the Henderson-Hasselbalch equation Causes; 3 Axis; · Vomiting (loss of gastric secretions; HCl) · Volume depletion · pH; normal = 7.35-4.45 · Alkali ingestion · HCO3-; normal = 24-25 mM · Hyperaldosteronism · PCO2; normal = 40 mmHg · Hyperkalaemia (leads to increased aldosterone release) The haemoglobin buffer line (curved lines) reflects what the blood is Results in an increased [HCO3-] and buffering capacity leading to a capable of doing with the amount of HCO3– it contains rise in pH Respiratory compensation; The body is always aiming to return to normal pH · Inhibition of chemoreceptors reducing respiration rate · Increases the PCO2 · This decreases the pH Reading the Davenport Diagram 1. Start at normal Respiratory Acidosis 2. What is the change in pH? Causes; CO2 retention · Higher pH = alkalosis · Hyperventilation · Lower pH = acidosis · COPD Compensation · Respiratory failure; type 1 = pulmonary embolism, type 2 = 3. Ignore the opposing side hypoventilation Base excess; compensatory increase or decrease in the base in order to counteract the rise in acid. Blood levels will show an Overall; increase in PCO2 (E.g. from 40 to 60 mmHg) making pH fall 4. Which aspect of this equation has changed? increased/decreased level of base for that level of acid. Base Renal compensation; CO2 + H2O ↔ H2CO3 ↔ H+ + HCO3- excess can be given as positive or negative · Increase HCO3– (high base excess) release into plasma Either; If the pH hasn’t returned to normal, it is partially compensated, if to buffer H+ created by the increased PCO2. · Respiratory: Changes in the PCO2 or H2O shifting equilibrium the pH is normal it is fully compensated Acute phase = CO2 moving into erythrocytes combines · Metabolic: Changes in H+ or HCO3– shifting the equilibrium If there is no base excess after a period of time, the with H2O (and carbonic anhydrase) to produce HCO3-. compensatory systems may be failing and this is a complex Chronic response increase HCO3– reabsorption by the 5. The direction of shift represents the acidosis/alkalosis acid/base disturbance kidneys, better for the stabilisation of pH · Excrete more H+ in the form of ammonia (NH3+ to NH4) 6. All movements must follow either the PCO2 axis or the Hb buffer lines Metabolic Acidosis · If respiratory, follow the Hb buffer line to the new PCO2 level Causes; creation, ingestion, retention of acids e.g. · If metabolic, follow the corresponding PCO2 axis to the · Renal failure appropriate [HCO3-] · GI HCO3– loss e.g. cholera Respiratory Alkalosis · Dilution of blood; the more H20 in the blood the more Causes; CO2 depletion due to hyperventilation 7. Compensation; how to restore pH acidic it gets (H20 dissociates into H+ and OH-) · Hypoxia · Respiratory; airway dysfunction so cannot change PCO2 therefore · Failure of H+ excretion e.g. hypoaldosteronism, less Na+ · Type 1 respiratory failure (e.g. pulmonary embolism, the kidneys compensate (chronically) but also capable of slight reabsorbed on the Na/H counter-transporter so less H+ decreased O2 and a decrease/no change in CO2) buffering in blood (acute) secreted · Metabolic; Usually kidney dysfunction, therefore the respiratory Diabetic ketoacidosis – high production of ketone bodies Results in too much CO2 loss E.g. from 40 to 27 mmHg) and an system compensates increase in pH Overall; increase in acid production of loss of buffering resulting in a Renal compensation; 8. Level of compensation decreased pH · Kidneys decrease H+ secretion by retaining H+ · Complex; no compensation Respiratory compensation; · This also results in a decreased HCO3– reabsorption in the · Partial; not restoring pH · Stimulates chemoreceptors to enhance respiration proximal tubules and collecting ducts (more excreted) · Fully; pH restored resulting in a falling PCO2 · Less buffering capacity, low base excess · Compensation results in a base excess · This increases the pH to return to normal · Therefore pH rises 1.14 Buffer Systems Revisited in Kidney and Respiratory Bicarbonate Buffering Ammonium Buffering Phosphate Buffering HCO3– is freely filterable at the renal corpuscles Significant reabsorption in the proximal tubule , ascending loop In the proximal tubule of Henle, and collecting duct Alkaline phosphate (HPO4 2-) is the most commonest Also excreted in the collecting duct non-bicarbonate urinary buffer Reabsorption is an active process; depending on the segment In the proximal tubule When all of the filtered HCO3- has been combined with secreted mediated by some combination of Na/K ATPase, Na/H Cells take up glutamine from both the glomerular filtrate & H+, the additional H+ secreted (as a result of Na+ absorption, counter-transporter, H/K ATPase, and H+ ATPase peritubular plasma and metabolise it to form NH3 (ammonia) since 1 Na+ is exchanged for 1 H+) then starts combining with The secreted luminal H+ reacts and eventually becomes H2O and & HCO3- (bicarbonate) filtered non bicarbonate buffers - namely HPO4 2- CO2 which can diffuse through the cell membrane or be taken up The NH3 then reacts with the H+ in the cell (either derived H+, generated from the dissociation of H2CO3 from within the by aquaporins from the dissociation of H2CO3 or from that absorbed as a tubular epithelial cells, combines with HPO4 2- to form H2PO4- CO2 and H2O are now intracellular where they dissociate again result of Na+ reabsorption) to form NH4+ (ammonium ion) which is then excreted in urine with carbonic anhydrase to form HCO3– which is transported The NH4+ is then actively secreted via Na+/NH4+ NOTE: the HCO3- produced from the dissociation of H2CO3 then across the basolateral membrane and the H+ is generally countertransport into the lumen and excreted, while the HCO3- enters the interstitial fluid, however, whereas in bicarbonate recycled into the lumen moves into the peritubular capillaries and thereby increases absorption, where there was no net gain in HCO3- since the No gain/loss of bicarbonate HCO3- levels (net gain of HCO3-) thereby ALKANISING the reabsorption HCO3- just replaced the HCO3- lost when it was If almost all of the HCO3– has been reabsorbed then the H+ will blood plasma filtered, with the phosphate buffer, there is no absorption of bind with a filtered non-bicarbonate buffer e.g. HPO4 2- HCO3- from the tubular lumen, yet HCO3- diffuses into the If H+ is binding with another buffer the intracellular HCO3– will interstitial fluid - resulting in a net gain of HCO3- in the be transported into the blood and this alkalinises it interstitium and thus blood plasma. This results in an increase in HCO3- concentration of the plasma and thus ALKALINIZES it FURTHER NOTE: Significant amounts of H+ combine with filtered non bicarbonate buffers such as HPO4 2- ONLY AFTER the filtered HCO3- has virtually ALL BEEN REABSORBED