Pharmacology Review - NRAN 80424 Spring 2024 - PDF
Document Details
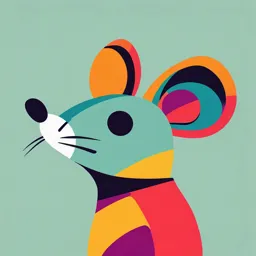
Uploaded by WorkableCreativity2568
TCU
2024
NRAN
Ron Anderson, M.D.
Tags
Summary
This is a pharmacology review document for NRAN 80424, Spring 2024. The document includes an outline, key points, and information on receptors, pharmacokinetics, and pharmacodynamics related to anesthetic agents. It is suitable for medical students or professionals.
Full Transcript
NRAN 80424 PHARMACOLOGY OF ANESTHETIC AGENTS 1 • Section I • • • • Pharmacology Review Mechanisms of Anesthesia IV Sedative-Hypnotics Exam (20% of grade) • Section III • • • • Physiology of Pain Opioids Local Anesthetics Exam (20% of grade) cumulative (< 20%) • Section IV • Section II • Uptake...
NRAN 80424 PHARMACOLOGY OF ANESTHETIC AGENTS 1 • Section I • • • • Pharmacology Review Mechanisms of Anesthesia IV Sedative-Hypnotics Exam (20% of grade) • Section III • • • • Physiology of Pain Opioids Local Anesthetics Exam (20% of grade) cumulative (< 20%) • Section IV • Section II • Uptake and Distribution • Inhaled Anesthetics • Exam (20% of grade) cumulative (< 20%) • Neuromuscular blockers • Exam (20%) cumulative (< 20%) • Section V • Chemotherapy and Antibiotics • Adjunct drugs • Review of Organ System Effects • Final Exam (20%) cumulative (100%) PHARMACOLOGY REVIEW NRAN 80424 SPRING 2024 RON ANDERSON, M.D. 2 KEY POINTS • GABA is the primary inhibitory neurotransmitter in the brain. Activation of the GABAA receptor results in influx of chloride and hyperpolarization of the cell. • Efficacy of a drug is the maximal response when all receptor sites are occupied, and is a function of the nature of the drug as a full, partial, or inverse agonist. • The primary mechanism of termination of action of a bolus dose of an anesthetic induction agent is redistribution to the vessel intermediate group. • Volume of distribution is the dosage divided by the initial plasma concentration of the drug, and is a model used to explain the measured concentration in the central compartment following a given dose. It does not represent actual volume. • Clearance of the high hepatic extraction ratio drugs is dependent on liver blood flow, while that of the low hepatic extraction ratio drugs is more dependent on enzymatic activity. 3 KEY POINTS • Glomerular filtration rate is considered the best measure of renal function, and creatinine clearance is the most reliable measure of GFR. • The pharmacokinetics of most anesthetic drugs can be adequately described by a two or three compartment model. • When a sedative-hypnotic drug is delivered into the central circulation, equilibration with the effect site is so rapid as to be indistinguishable from a pharmacokinetic standpoint. The lag between peak plasma concentration and peak effect (hysteresis or biophase or k) is a pharmacodynamic effect. • The volume of distribution at time of peak effect (Vdpe) provides a more useful guideline for determining proper bolus dosing. • The context-sensitive decrement-time provides us useful information about termination of effect following a drug infusion of a given duration. • Wide interpatient variability exists (much of which is unpredictable) emphasizing the need to individualize and titrate both bolus and infusion dosing. 4 OUTLINE • Receptors • Pharmacokinetics • Absorption • Distribution • Elimination • Pharmacokinetic Models • Pharmacodynamics 5 RECEPTORS Receptor Types G Protein-Coupled Ion Channels Ligand-gated Excitatory Inhibitory Voltage-gated G Protein-gated Other-gated The Synapse Receptor Theory 6 RECEPTOR TYPES • Cell Surface Receptors • Bind an extracellular molecule (ligand) and convert this input into a change in intracellular behavior • Intracellular Receptors • Examples • Steroid receptors in nucleus regulate transcription of genes • Cytosolic receptors of phosphodiesterase inhibitors increase cAMP concentration 7 CELL SURFACE RECEPTORS • Three types • G protein-coupled receptors • Largest family of receptors • Acts through activation or inhibition of an: • Enzyme • Ion channel • Other target • Ion channel • • • • Ligand-gated Voltage-gated G protein-gated Other-gated • Enzyme-linked transmembrane • Typically a tyrosine kinase that phosphorylates an intracellular second messenger • E.g. Insulin receptor increases expression of GLUT 8 9 G PROTEIN-COUPLED RECEPTORS • Consists of a protein linked to 7 transmembrane spanning domains • Coupled to specific G proteins • There are many types of Gα,β and γ proteins • Binding activates the Gα protein which then activates or inhibits an: • Enzyme • Ion channel • Other target FLOOD 10 G PROTEIN-COUPLED RECEPTORS • Ligand binding results in a conformational change in the receptor • Allows substitution of GTP for GDP on the Gα protein • GTP bound Gα protein acts as a second messenger where GTP hydrolyzed back to GDP releasing energy to affect the target protein • GDP bound Gα returns and binds to the receptor FLOOD 11 NEUROTRANSMITTERS AND ANESTHESIA-RELATED DRUGS WHICH TARGET G PROTEIN-COUPLED RECEPTORS • Opioids - μ receptor • Atropine and glycopyrollate – muscarinic Ach receptor • Norepinephrine • Substance P • Endogenous and exogenous • Excitatory and inhibitory in CNS • Descending inhibitory pathways in spinal cord • VLPO sleep-wake cycle • Adrenergic drugs • Dopamine • Primary catecholamine in CNS • Excitatory and inhibitory • Neurokinin-1 receptor in spinal cord • Antihistamines • Histamine important in TMN sleep-wake cycle • Adenosine • Some antiemetics • Via dopamine and serotonin receptors 12 ION CHANNEL RECEPTORS • Resting membrane potential ~ -70mV • When ion channels open ions flow in or out based on their concentration gradients • Na+, Cl-, and Ca++ have higher extracellular concentrations • K+ has a higher intracellular concentration • Na+ and Ca++ entry depolarizes the cell • Cl- entry hyperpolarizes the cell • K+ exits the cell to repolarize it following a depolarization • 10,000 – 100,000 ions/channel/millisecond flow through potentially thousands of channels with each action potential FLOOD LIGAND-GATED ION CHANNELS • Excitatory • Glutamate receptors • NMDA, AMPA, Kainate • Nicotinic acetylcholine receptors • CNS and neuromuscular junction • Serotonin • Inhibitory • GABA • Glycine 13 EXCITATORY LIGAND-GATED ION CHANNELS • Glutamate – primary excitatory neurotransmitter in CNS • Important in learning, memory, and central pain transduction • Glutamate receptors • Non-selective cation channels • Inotropic Glutamate Receptors • NMDA • Associated with neuropathic pain and opioid tolerance • Blocked by ketamine • AMPA Both associated with rapid synaptic transmission, synaptic plasticity, • Kainate and long-term potentiation • Metabotropic Glutamate Receptors • G protein-coupled 14 EXCITATORY LIGAND-GATED ION CHANNELS • NAChr • Non-selective cation channels • Acetylcholine acts as an excitatory stimulus at CNS NAChr producing arousal • Produces muscular contraction at the NMJ NAChr • Serotonin • Excitatory • Non-selective cation channel 15 16 INHIBITORY LIGAND-GATED ION CHANNELS • GABA (γ – Aminobutyric Acid) Receptor • Primary inhibitory neurotransmitter in brain • Composed of 5 subunits (2 α , 2 β, 1 γ) • When two GABA molecules bind the receptor a Clchannel opens, hyperpolarizing the cell • Actions of IV anesthetic drugs on GABAA receptor • Benzodiazipines • Increase sensitivity of receptor to endogenous GABA • Propofol, Etomidate, Thiopental • Increase sensitivity of receptor to GABA • At high concentrations can directly open the Cl- channel FLOOD INHIBITORY LIGAND-GATED ION CHANNELS • Glycine Receptor • Spinal cord • Glycine is the primary inhibitory neurotransmitter • Operates via a chloride channel • Brain • Glycine receptors in the brain modulated by multiple anesthetic agents, but currently no defined anesthetic effect related to this • A couple notes: • Absorption of glycine containing irrigant during TURP may produce a transient blindness • Strychnine and tetanus toxin antagonize the inhibitory effect of glycine, producing activation of the EEG and potentially seizures 17 VOLTAGE-GATED ION CHANNELS • Open in response to changes in voltage across the cell membrane • Important function in transmission of impulses in neurons and skeletal muscle • Typically named for the ion that traverses the channel when depolarization occurs • Examples important in anesthesia: • Voltage gated sodium channel • Blocked by the local anesthetics • hERG potassium channel (ether-a-go-go) • Inward rectifying K+ channel • Affected by multiple drugs, e.g. droperidol, volatile anesthetics, locals • May result in iatrogenic LQTS and torsades de point 18 19 G PROTEIN-GATED ION CHANNELS • Ion channel activated by a G protein-coupled receptor from the interior of the cell • Best studied are the G protein regulated inwardly rectifying potassium channels (GIRKs) • K+ flows with the electrical, rather than the concentration, gradient • Activated by the Gβγ rather than Gα • Multiple receptors coupled to GIRKs • • • • • • FLOOD Muscarinic M2 in heart α2 adrenergic A1 adenosine D2 dopamine GABAB etc OTHER-GATED ION CHANNELS • Many other ion channels which respond to: • Ions • Second messengers • Tissue injury 20 THE SYNAPSE 21 FLOOD THE SYNAPSE • Synaptic Modulation • Resting postsynaptic transmembrane potential ~ -70mV in CNS. This is further modulated by postsynaptic excitatory and inhibitory potentials • Synaptic Delay • 0.3 – 0.5 millisecond consisting of: • Neurotransmitter release • Diffusion of NT to postsynaptic receptor • Change in permeability and ion flux due to receptor activation • Synaptic Fatigue • Decreased postsynaptic firing following rapid, repetitive firing • Presumably 20 exhaustion of NT • Posttetanic Facilitation • Rapid firing of an excitatory stimulus followed by a rest period leads to increased response at the postsynaptic neuron • Other factors • Neuronal excitability increased by alkalosis • Hypoxia decreases neuronal excitability 22 23 RECEPTOR THEORY • Agonists are characterized by: • Efficacy • Maximal response when all receptor sites are occupied • Reflects the agonists ability to activate the receptor • Full agonist = high efficacy • Partial agonist = low efficacy • Potency • Defined by the dose (or concentration) needed to produce a defined effect ED50 or (EC50) BARASH RECEPTOR THEORY • Antagonists • Competitive antagonist • Binds at the orthosteric site but doesn’t activate the receptor • Binding of the agonist and competitive antagonist is mutually exclusive • Can be overcome by large doses of agonist • Noncompetitive antagonist • • • • Binds at an allosteric site Can bind whether the orthosteric site is bound by an agonist or not Agonist binding is not affected, but receptor activation is blocked Can not be overcome by large doses of agonist 24 RECEPTOR THEORY • Serial binding-activation vs. Allosteric receptor activation models • Allosteric Receptor Activation Models • Allow for receptor activation in the absence of agonist (Constitutive activity) • Receptors fluctuate between multiple conformations and ligand binding may favor a particular state over another • Provide a better understanding of partial and inverse agonists • Partial agonist • Binds a receptor but does not favor an active state as much as the full agonist • Even if 100% of receptors are bound, less than 100% will be in an active state • Inverse agonist • Binds the receptor, but favors an inactive state by binding to the inactive receptor more strongly than an active receptor 25 RECEPTOR THEORY (SERIAL BINDING-ACTIVATION MODEL) 26 FLOOD FLOOD RECEPTOR THEORY (ALLOSTERIC RECEPTOR ACTIVATION MODEL) 27 FLOOD FLOOD RECEPTOR THEORY - ADDITIONAL CONCEPTS • Spare Receptors • Exist in the presence of a maximal tissue response in the absence of 100% receptor binding by an agonist • E.g. Neuromuscular transmission • Very high density of NACHRs in critical muscles like diaphragm • Usually only a small fraction of receptors must be activated to produce contraction • Important in differential blockade of muscle groups with the NDPMRs • Signal Amplification • G protein activation by the GPCRs lasts much longer than the actual binding of ligand at the receptor and may activate multiple second messengers • Signal Damping • Diminished response to repeated drug administration • Multiple mechanisms • • • • Receptor desensitization Downregulation Depletion of neurotransmitters Transcriptional changes 28 PHARMACOKINETICS Absorption Distribution Volume of Distribution Protein Binding Elimination Metabolism Hepatic Clearance Renal Clearance 29 ABSORPTION • Most drugs are weak acids or weak bases • At physiologic pH they exist in non-ionized or ionized forms • The non-ionized form can diffuse across cell membranes • • • • • Blood-brain barrier Renal tubular epithelium GI epithelium Hepatocytes Placenta • When pK = the pH, the drug is 50% ionized • To render a drug more water soluble: • Basic drugs come in an acidic solution • Acidic drugs come in a basic solution 30 REVIEW OF pKa • log protonated = pKa – pH unprotonated • For a weak acid, the protonated form is non-ionized HA A- + H+ • So, the greater the pKa than the pH, the more protonated, non-ionized form is present • For a weak base, the protonated form is ionized HB+ B + H+ • So, the greater the pKa than the pH, the more protonated, ionized form is present pKa EXAMPLES • Local anesthetics (weak bases) • Mepivacaine (pKa = 7.6) • pKa – pH = 0.2 = 61% ionized/ 39% non-ionized • Bupivacaine (pKa = 8.1) • pKa – pH = 0.7 = 83% ionized/17% non-ionized The greater the pKa than the pH the more is protonated and ionized • Opioids (weak bases) • Sufentanil (pKa = 8.0) • pKa – pH = 0.6 = 80% ionized/20% non-ionized • Alfentanil (pKa = 6.5) • pKa – pH = -0.9 = 11% ionized/89% non-ionized If pKa – pH is a negative number, it means that less than 50% of the drug is protonated (which for a weak base is ionized) so greater than 50% is non-ionized. This is the reason for the rapid onset of alfentanil. 33 ABSORPTION NON-IONIZED IONIZED • Lipid soluble • Poorly lipid soluble • Easily absorbed from the GI tract • Poorly absorbed from the GI tract • Pharmacologically active • Pharmacologically inactive • Reabsorbed in the renal tubules • Excreted by the kidneys • Metabolized by the liver • Reduced hepatic metabolism ABSORPTION • Ion Trapping • Only the non-ionized portion of drug equilibrates across a lipid membrane • This can result in varying concentration of drug in compartments with different pHs • Examples: • A basic drug in the stomach becomes highly ionized and concentrated • A basic drug which crosses the placenta in an unionized form becomes ionized in the more acidic fetal circulation • Drug continues to cross into the fetal circulation because only the non-ionized portion is equilibrating, so concentration of the drug occurs • In a compromised (acidotic) fetus this mechanism is compounded 34 ABSORPTION • Routes of Administration • Intravenous • Absorption not really an issue • Oral • Principle site of absorption is small intestine due to the large surface area • First-pass effect (GI tract portal vein liver systemic circulation) • Oral transmucosal • Sublingual, buccal, nasal drains to the SVC • Bypasses the liver 35 ABSORPTION • Routes of Administration • Transdermal • Rate-limiting step is absorption through the stratum corneum, the thickness of which varies widely throughout the body • Available in transdermal preparations • NTG, fentanyl, scopolamine, clonidine, estrogen and progesterone • Rectal • Unpredictable response • Irritation of the rectal mucosa • Subcutaneous and intramuscular 36 DISTRIBUTION • Once reaching the systemic circulation drug is distributed to various tissues relative to the perfusion of those tissues • Most rapid equilibration occurs in the most highly perfused tissues which are also relatively small in volume • • • • Brain Heart Lungs Kidneys • Less well perfused and higher volume tissues equilibrate at a slower rate • Intermediate • Muscle • Slowest • Fat • Bone 37 DISTRIBUTION V2 RAPIDLY EQUILIBRATING COMPARTMENT (VESSEL INTERMEDIATE) (MUSCLE) k12 k13 V1 V3 SLOWLY EQUILIBRATING COMPARTMENT CENTRAL COMPARTMENT (VESSEL POOR GROUP) k21 k31 k10 k1e (FAT, BONE) E EFFECT ke0 38 DISTRIBUTION 39 40 DISTRIBUTION • Redistribution • Following a bolus dose of an anesthetic induction agent, high concentrations of drug are rapidly attained in the central compartment (effect site) • As drug is redistributed to other compartments due to blood flow, lipid solubility, and clearance, the initial effect is rapidly terminated • The primary mechanism of termination of action of a bolus dose of induction agent is REDISTRIBUTION to other tissues, primarily muscle DISTRIBUTION • Redistribution • Drug is distributed by concentration gradients and equilibrium coefficients • As drug is taken up by the less well perfused tissues, brain concentration exceeds blood concentration and drug will move back into the plasma and continue being distributed to other tissues • Repeated boluses or infusion of a lipid soluble drug can result in accumulation of drug in adipose tissue • In the case of propofol, the return of drug from the vessel poor group (fat) is slower than clearance of the drug, so the brain concentration remains very low and there is not delayed awakening due to redistribution from fat to the central compartment 41 VOLUME OF DISTRIBUTION • Vd = Amount of drug administered / Initial drug plasma concentration • Drug distribution and the apparent volume of distribution is a function of: • Affinity of the drug for various tissues versus blood • Mass of tissue the drug is distributed in • Volume of distribution is a model to explain the concentration of drug in the central compartment (blood) following a given dose • May range from actual plasma volume to a number which far exceeds total volume of the body • An imaginary number of liters of blood that would be required to dissolve a dose of drug and result in the measured concentration 42 VOLUME OF DISTRIBUTION • Things affecting Volume of Distribution • • • • Lipid solubility Protein binding pH (Ionization of a drug) Actual volume change e.g. • Pregnancy • Ascites • Change in tissue composition • Age • Obesity • Perfusion?? • Why do we care? • Because: Loading dose = Vd x Target concentration 43 PROTEIN BINDING • Most drugs are protein bound to some extent • Effect on drug distribution • Only free drug can cross cell membranes • Effect on drug potency • Only free drug can bind receptors • Lipophylic drugs more avidly bind plasma proteins and lipids in fatty tissue • The IV sedative-hypnotics are generally highly protein bound and decreases in plasma protein binding may increase the concentration of free drug requiring a reduction in dose 44 PROTEIN BINDING • Things which decrease the concentration of plasma proteins • • • • Significant hepatic disease Age Pregnancy Renal failure • Changes in plasma protein concentrations have the greatest effect on highly protein bound drugs • The effect is not as great as would be predicted by alteration in plasma protein concentration alone 45 ELIMINATION • Elimination consists of: • Metabolism • • • • • Oxidation Reduction Conjugation Hydrolysis Glucuronidation • Clearance • Hepatic • Renal 46 METABOLISM • Cytochrome P450 Enzymes • Location • Primarily Hepatic • Mitochondrial • Designation • Grouped into families e.g. CYP3 and subfamilies CYP3A • Individual enzymes within a subfamily are identified with a number CYP3A4 • The CYP3A4 enzyme is the most common and is responsible for the metabolism of the majority of drugs • Enzyme induction or inhibition can affect metabolism of drugs leading to a lesser or greater drug effect, respectively 47 METABOLISM • Phase I Reactions • Increase the drugs polarity preparing it for phase II reactions • Dependent on CYP450 enzymes • Oxidation • Reduction • Conjugation • Independent of CYP450 enzymes and much of it occurs outside the liver • Hydrolysis • Phase II Reactions • Increases the water solubility of the drug • Transferase enzymes • Glucuronidation 48 49 HEPATIC CLEARANCE • Rate of metabolism (R) occurring in the liver equals the difference between the concentration entering the liver and the concentration exiting the liver times the blood flow through the liver • R = Q (Cinflow – Coutflow) • The hepatic extraction ratio (ER) is the fraction of drug entering the liver which is removed • ER = Cinflow – Coutflow Cinflow • Clearance is the amount of blood cleared of drug per unit time • Clearance = Q x ER = Q Cinflow – Coutflow Cinflow FLOOD HEPATIC CLEARANCE • Hepatic Extraction Ratio • High HER drugs • Liver has very high capacity to metabolize the drug • Clearance is therefore dependent on liver blood flow rather than changes in enzymatic activity • Metabolism is “flow-limited” • E.g. Propofol, lidocaine, sufentanil • Low HER drugs • Liver has limited capacity to take up and metabolize the drug • Clearance is therefore dependent on enzymatic capacity rather than liver blood flow • Metabolism is “capacity-limited” • Metabolism is more affected by enzyme induction or inhibition • E.g. Alfentanil, diazepam 50 51 HEPATIC EXTRACTION RATIO CHANGES IN LIVER BLOOD FLOW CHANGES IN ENZYME ACTIVITY (Vm) FLOOD HEPATIC EXTRACTION RATIOS OF COMMON DRUGS LOW HER Alfentanyl Diazepam Lorazepam Methadone Phenytoin Theophyline Thiopental Warfarin INTERMEDIATE HER Methohiexital Midazolam Vecuronium High HER Bupivicaine Diltiazem Etomidate Fentanyl Ketamine Labetalol Lidocaine Meperidine Metoprolol Morphine Naloxone Propofol Propranolol Sufentanil Verapamil RENAL CLEARANCE • Total amount of drug excreted over a specific time, and involves: • Glomerular filtration • Dependent on: • GFR • Free (unbound) fraction of drug • Tubular secretion • Active process • Different processes for various drugs • May include secretion of protein bound drug • Tubular reabsorption • Passive process • Water soluble drugs • Some return as water is passively reabsorbed from the tubules, some is lost • Lipophylic drugs • Readily cross cell membranes and are extensively reabsorbed from the tubules 53 RENAL CLEARANCE • GFR considered best measure of renal function • Creatinine Clearance is most reliable measure of GFR • Calculated value: Creatinine clearance(ml/min) = (140-age) x lean body mass (kg) Plasma creatinine(mg/dl) x 72 • Measured value: Creatinine Clearance(ml/min) = Urinary creatinine conc. (mg/100ml) Plasma creatinine conc. (mg/100ml) X Urine Volume(ml/min) • Normal values: • Female: 85 – 125 ml/min • Male: 95 – 140 ml/min • Decreases with age 54 PHARMACOKINETIC MODELS Zero and First-Order Processes Physiologic Models Compartment Models One Compartment Multi Compartment Hysteresis – Combined PK/PD Model Stochastic Dosing Guidelines Bolus Dosing Maintenance Context Sensitive Decrement-time Target-controlled Infusions Closed-loop Infusions Response Surface Models 55 ZERO- AND FIRST-ORDER PROCESSES • Zero-order process • Rate of change is constant • A fixed amount of drug is removed per unit time dx = k dt • First-order process • Rate of change is proportional to the amount of drug present dx = k · x dt 56 57 PHYSIOLOGIC MODELS • Involves measurement(at multiple times) the concentration of drug in each tissue • Complex, inefficient, and generally provide no better information than grouping tissues into hypothetical compartments • Did provide definitive evidence that termination of effect of a bolus dose of anesthetic induction agents results from: • Redistribution of drug from brain to muscle, with almost no effect from: • Metabolism of drug • Redistribution to the vessel-poor tissues FLOOD COMPARTMENT MODELS • Group the individual organs of the physiologic models into compartments based on perfusion • The pharmacokinetics of most anesthetic drugs can be adequately described by a two or three compartment model • Assumptions • These assumptions also hold true for non-compartmental analysis and physiologic models • Clearance only occurs from the central compartment • Distribution and elimination of the drug is a linear, first-order process • Time invariant 58 59 TWO COMPARTMENT MODEL BARASH BARASH THREE COMPARTMENT MODEL 60 BARASH THREE COMPARTMENT MODEL MILLER 61 THREE COMPARTMENT MODEL 62 HYSTERESIS FLOOD 63 HYSTERESIS – COMBINED PK/PD MODEL BARASH 64 HYSTERESIS / BIOPHASE – COMBINED PK/PD MODEL MILLER 65 MILLER STOCHASTIC MODELS • Mathematical technique sometimes labeled non-compartmental • May avoid some of the confusion related to trying to perceive a compartment as specific tissues • Make the same “assumptions we discussed with compartmental models • Varies primarily by incorporation of the concept of mean residence time (MRT) • The average time a molecule of drug spends in the body before being eliminated • Fails to adequately describe the redistribution of drug from effect site to less well-perfused tissues, and then ultimately back to the central compartment for clearance 66 DOSING GUIDELINES • Bolus Dosing • In a typical three compartment model there are multiple volumes to consider: • V1 (volume of the central compartment) • V2 (volume of the vessel-intermediate group) • V3 (volume of the vessel-poor group) • Vdss (sum of all the above at steady state) • Vdpe (volume of distribution at the time of peak effect following a bolus dose) 67 68 DOSING GUIDELINES (360) FLOOD DOSING GUIDELINES • Maintenance infusion rate = CT × V1 × (k10 + k12e−k21t + k13e−k31t) • This complex formula accounts for the: • • • • Target concentration Central compartment volume Clearance from the central compartment Redistribution between the central and peripheral compartments • At steady state t = ∞, and the equation becomes CT · V1 · k10 • Bottom Line • We give more at the start to account for redistribution out of the central compartment to the peripheral compartments • Once steady state is achieved the infusion rate to maintain a given concentration essentially matches the amount being cleared 69 70 DOSING GUIDELINES • If you just start an infusion, the initial rate must be much higher to maintain the target concentration at the effect site in the face of redistribution to other tissues • In practice, we often give a bolus dose (based on the Vdpe) which covers some of the initial redistribution • While the infusion rate will still have to be higher initially than at steady state, this reduces the difference to some extent CONTEXT SENSITIVE DECREMENT-TIME • Context-sensitive half-time • The time required for the drug concentration to decrease by 50% following termination of a steady state infusion • Increases with increased duration of the infusion • Due to increased distribution of drug in the peripheral compartments over time • Context-sensitive decrement-time • Allows the substitution for 50% of any decrement which may prove more valuable • MACAWAKE = The drug concentration where 50% of patients follow commands 71 CONTEXT SENSITIVE DECREMENT-TIME 72 MILLER ISOCONCENTRATION NOMOGRAMS AND INFUSION DOSING SCHEMES 73 • Isoconcentration nomogram • Using a pharmacokinetic model, the plasma concentration achieved over time with various infusion rates are plotted • A desired plasma concentration is selected and a horizontal line drawn at that concentration • The vertical lines indicate when you would reduce your infusion rate to maintain the desired plasma concentration BARASH 74 BOLUS AND INFUSION DOSING SCHEME • The BET scheme • B = bolus • E = infusion rate to compensate for elimination • T = decreasing rate over time to compensate for distribution to peripheral tissues TARGET CONTROLLED INFUSIONS • Depend on pharmacokinetic and pharmacodynamics models • Follow the equation CT × V1 × (k10 + k12e−k21t + k13e−k31t) • Must account for: • Desired concentration and plasma volume • Elimination rate • Distribution changes between the central and peripheral compartments over time • Available in several countries, but not currently in the United States • Use pharmacokinetic parameters derived from population studies • Results in up to 30% error due to individual patient variation 75 TARGET CONTROLLED INFUSIONS – OTHER USES • Patient controlled postoperative analgesia • Alfentanil • Patient controlled sedation • Propofol • Mechanism • An infusion rate is established • Patient demand dose increases the rate by a small amount • Lack of subsequent patient demand allows the infusion rate to slowly fall to the initial infusion rate 76 CLOSED-LOOP INFUSIONS • Experimental, not available commercially for anesthetic purposes • Drug delivery is controlled by “output” from the patient • Processed EEG (e.g. BIS) • Auditory evoked potentials • Change in patient “output” result in: • Change in drug delivery rate • + Change in pharmacokinetic model • Limited usefulness at this point due to inadequate definitions of what entails anesthesia and inadequate monitoring of anesthetic depth 77 78 RESPONSE SURFACE MODELS • Designed to look at the net effect of combining two drugs which may be: • Additive • Synergistic • Antagonistic • Components • Concentrations of the two drugs • Desired effect • Probability isoboles • Typically 5%, 50%, and 95% MILLER INDIVIDUAL VARIABILITY • Variation in plasma concentrations using the same dosing regimen • Up to 2-fold • Variation in plasma concentration required to achieve the same effect • Up to 5-fold • Causes • Genetic • Most important determinant of metabolic rate (alteration in CYP450 enzymes) • Greatest effect on low hepatic-extraction-ratio drugs • Disease processes • Changes in receptor numbers and sensitivity • Alterations induced by inhaled anesthetics 79 PHARMACODYNAMICS Concentration-Response Relationships Potency and Efficacy Effective and Lethal Dose Interpatient Variability 80 81 CONCENTRATION RESPONSE RELATIONSHIPS • Potency • Dose required to produce a given effect • Efficacy • The maximum effect the drug can produce • What do these look like? • Partial agonist • Presence of a competitive antagonist • Presence of a non-competitive antagonist BARASH 82 EFFECTIVE AND LETHAL DOSE • ED50 • LD50 • Dose required to produce a specific effect in 50% of patients • Dose producing death in 50% of patients • Therapeutic index • Ratio of LD50/ED50 • Greater the ratio the safer the drug • LD1/ED99 FLOOD INTERPATIENT VARIABILITY • Variation in plasma concentrations using the same dosing regimen • At least 2-fold • Variation in plasma concentration required to achieve the same effect • At least 5-fold • Other factors in dosing decisions: • Hemodynamic consequences of drug administration • Delay in onset of desired effect • Random inter-individual variability 83 SOURCES • Flood Stoelting’s Pharmacology and Physiology in Anesthetic Practice 6th edition. 2022 • Barash Clinical Anesthesia 8th edition. 2017 • Evers Anesthetic Pharmacology 2nd edition. 2011 • Miller Miller’s Anesthesia 9th edition. 2020 84